Figures & data
Figure 1. ACK’s architecture and activation. (upper panel) ACK’s domain architecture with domain structures (kinase domain-SH3 PDB: 4HZS, CRIB PDB: 1CF4) or predictions (AlphaFold) shown. ACK encompasses a sterile alpha motif (SAM) domain, nuclear export signal (NES), TK domain, Src homology-3 (SH3) domain, CRIB motif, clathrin binding region, epidermal growth factor receptor-binding (EBD) domain and a ubiquitin association (UBA) domain. Amino acid numbering of domain boundaries is shown above the respective domains. (lower panel) ACK signals downstream of [Citation1] G protein-coupled receptors (GPCRs) [Citation2], Receptor tyrosine kinases (RTKs) and [Citation3] Integrins. RTK stimulation (2a) causes relief of ACK’s autoinhibitory interactions, (2b) SAM-mediated dimerization and (2c) ACK activation. To become fully active, 2(d) ACK is phosphorylated, either by Src or autophosphorylation, on its activation loop at Tyr284. Figure created in BioRender.
![Figure 1. ACK’s architecture and activation. (upper panel) ACK’s domain architecture with domain structures (kinase domain-SH3 PDB: 4HZS, CRIB PDB: 1CF4) or predictions (AlphaFold) shown. ACK encompasses a sterile alpha motif (SAM) domain, nuclear export signal (NES), TK domain, Src homology-3 (SH3) domain, CRIB motif, clathrin binding region, epidermal growth factor receptor-binding (EBD) domain and a ubiquitin association (UBA) domain. Amino acid numbering of domain boundaries is shown above the respective domains. (lower panel) ACK signals downstream of [Citation1] G protein-coupled receptors (GPCRs) [Citation2], Receptor tyrosine kinases (RTKs) and [Citation3] Integrins. RTK stimulation (2a) causes relief of ACK’s autoinhibitory interactions, (2b) SAM-mediated dimerization and (2c) ACK activation. To become fully active, 2(d) ACK is phosphorylated, either by Src or autophosphorylation, on its activation loop at Tyr284. Figure created in BioRender.](/cms/asset/c71714d7-df4c-473a-b857-343bcb669a8e/ksgt_a_2212573_f0001_oc.jpg)
Figure 2. ACK regulates the stability of diverse proteins. Schematic representation of ACK’s involvement in protein homoeostasis. ACK binds p53, p27, Wwox and RhoGDI and phosphorylates all except RhoGDI-3, targeting the proteins for subsequent changes in ubiquitination and proteasomal degradation. Figure created in BioRender.
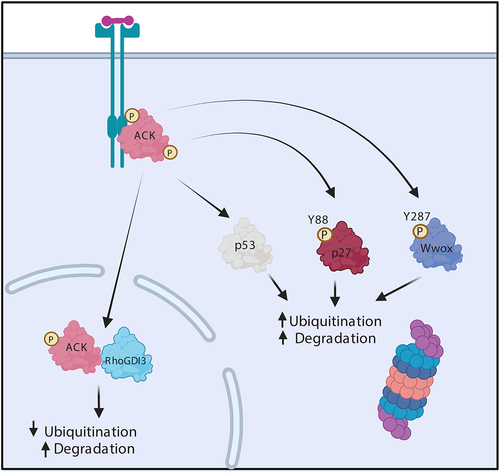
Figure 3. ACK regulates EGFR degradation. (a) in normal physiology, ACK binds to EGFR following EGF stimulation. ACK co-localizes with EGFR during internalization and is subsequently ubiquitinated by Nedd4-1/2 and degraded alongside EGFR. ACK ubiquitination acts as a sorting signal that directs the complex to late endosomes/lysosomes. (b) in cancer, ACK overexpression slows EGFR endocytosis and causes EGFR retention in early endosomes leading to increased cell survival and proliferation signals. Little EGFR is degraded and most internalized EGFR is eventually recycled. Figure created in BioRender.
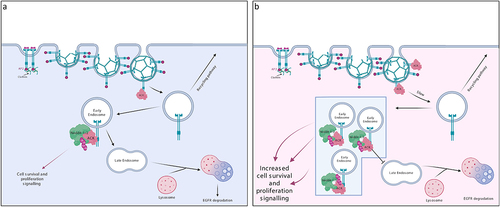
Figure 4. ACK regulates the stability of the PI3-Kinase regulatory subunits. ACK binds and phosphorylates the PI3-Kinase regulatory subunits p85α(Tyr607), p55α(Tyr337), p50α(Tyr307) and p85β(Tyr599). ACK protect the isoforms from ubiquitination and proteasomal degradation. Phosphorylated p85 isoforms dimerize through pTyr607-nSH2 interactions and promote cell proliferation in a PI3-kinase independent mechanism. ACK also regulates a minor pathway in AKT activation. ACK binds and phosphorylates AKT at Tyr176 leading to plasma membrane recruitment via an interaction with phosphotidic acid, after which pTyr176-AKT can be further phosphorylated and activated. pTyr176-AKT in complex with ACK is also found in the nucleus where it acts to phosphorylate Fox0, which is the excluded from the nucleus. Figure created in BioRender.
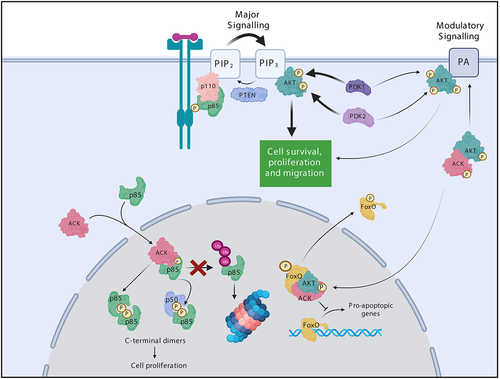