Figures & data
Figure 1. Induction Nano Intravital Device (iNANIVID) optimized for intravital imaging. (A) iNANIVID next to a 17.9 mm U.S. dime for reference. (B) Use of the iNANIVID for intravital imaging within solid tumors imposes strict requirements of the design of the device. Insertion into stiff tumor tissue requires a small form factor (3 mm) and a sharp point. The chamber outlet is designed to be at the proper depth for multiphoton imaging when the device is fully inserted into the tumor (back edge flush with the tumor surface). Fluorescent beads placed in etched wells within the cover aid in locating the device outlet under the microscope. An obtuse shape prevents shadowing of the outlet during imaging. (C) The small size of the iNANIVID makes manual manipulation challenging. An insertion tool designed to facilitate implantation into the tumor tissue aids in manipulation and insertion. The front edge of the tool matches the obtuse shape of the iNANIVID and ensures insertion at the proper angle. The plunger ensures insertion to the proper depth. Scale bar = 10 mm. (D) 3D computer aided design rendering of the device with the direction of insertion indicated. Yellow indicates the back surface which is visible under the microscope. An asterisk indicates the location on the back surface, directly above the device outlet. (E) Schematic of a cross section of the tumor with the implanted device illustrating a diffusion gradient emanating from the device outlet. Red dots indicate the fluorescent bead chambers etched into the cover. (F) View of the tumor with the implanted device as would be viewed from the microscope perspective. Asterisk indicates the device outlet.
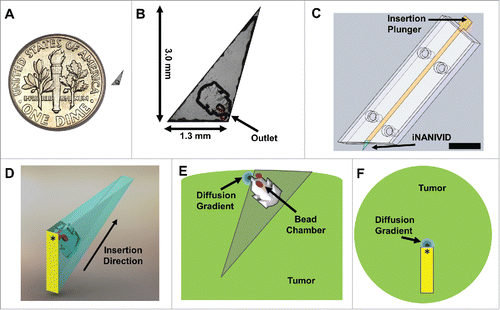
Figure 2. Tumor fixturing. Visualization of single cell movement within a tissue requires motion and artifact free imaging that can be obtained by proper tissue fixturing. (A) Supine view of a mouse after skin flap surgery to expose the underlying mammary tumor. (B) A hole slightly larger than the tumor is cut into a sheet of rubber and placed around the tumor forming a hydration chamber that is filled with PBS to keep the tissue hydrated over the course of the experiment. To provide support, plastic-backed foam is attached to the skin behind the tumor with surgical staples. (C) Side view showing the tumor within the hydration chamber with the fixturing apparatus in place. Strips of hard rubber are affixed with tape to the foam backing to immobilize the tissue to the microscope stage adaptor, without causing tissue compression. iNANIVID devices are inserted into the tumor and a coverslip is held in place with an O-ring.

Figure 3. MDA-MB-231 tumor cells expressing Dendra2 migrate in vivo up a chemoattractant gradient produced by an iNANIVID loaded with 2 µM EGF. After iNANIVID insertion, a region of the tumor approximately 150 µm away from the device was photocoverted (red). Non-photoconverted cells can be seen closer to the device (green) with collagen fibers (blue) visualized by second harmonic generation. Timelapse multiphoton microscopy was performed every 3 minutes for 4 hours to obtain a Z-stack of the tumor. (A) Image of the iNANIVID outlet region taken 120 minutes after device insertion (time noted on top right). An approximate outline of the top surface of the iNANIVID (see ) at the bottom of the image has been overlaid for clarity (white). The device outlet location has been marked with an asterisk. Cell outlines are shown from manual tracing to track migration over the time lapse. Scale bar = 100 µm. (B) At 145 minutes, cells have begun to migrate up the gradient of EGF produced by the iNANIVID. Cells have been outlined and their paths highlighted. (C) By 168 minutes, additional cells have responded to the gradient and migrated toward the device. Multiple cells have moved over 100 µm. (D) Vector plot showing directional migration of 33 tumor cells from 3 experiments. Each vector represents the net displacement of an individual cell plotted on a common origin.
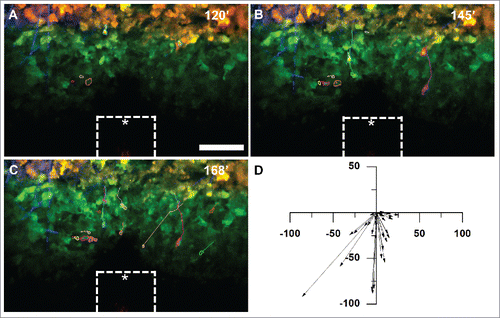
Figure 4. Tumor cells do not migrate toward an iNANIVID loaded with hydrogel that does not contain EGF. Timelapse multiphoton microscopy was performed with an iNANIVID that did not contain a chemoattractant as a control. Experimental procedure was the same as that of . An approximate outline of the top surface of the iNANIVID (see ) at the bottom of the image has been overlaid for clarity (white). (A) Image taken 120 minutes post iNANIVID insertion. Since cell movement was random and to a lesser extent than in the EGF experiments, the 20 most motile cells in the timelapse were chosen for tracking. Scale bar = 100 µm. (B) At 145 minutes, most cells have moved less than 20 µm with no directional trend toward the iNANIVID. (C) At 168 minutes, only random migration is observed and most cells have moved less than 20 µm. (D) Vector plot showing displacement of 46 tumor cells after 51 minutes have elapsed. (D) Vector plot showing displacement of 46 tumor cells (from 3 experiments) after 51 minutes have elapsed.

Figure 5. Analysis of tumor cell motility parameters in response to the iNANIVID. Cell motility parameters derived from the tracks of cells that migrated at least 10 µm in triplicate experiments. Cells migrated with (A) a significantly higher directionality (***, p<0.001) and (B) a significantly lower turning frequency toward the EGF iNANIVID than in the absence of the iNANIVID or with the control devices loaded with PBS indicating more directional higher efficiency migration (***, p<0.001). (C) Chemotactic index toward the EGF loaded device was also greatly increased compared with the control consistent with panels A&B (**, p<0.01). (D) While mean velocities were not significantly different between the three conditions, the variability of the instantaneous cell velocities was higher with the EGF device and velocities of 4.0 – 10.0 µm / min were frequently observed. (E) Histogram showing distribution of instantaneous velocities of all tracked cells. Most of the cells in the control have a velocity less than 2.0 µm / min and none were observed above 4.0 µm / min.

Figure 6. Generation of defined microenvironments via the iNANIVID. (A) Protein expression of membrane GLUT1 (red) and DAPI nuclear stain (blue) is shown in HEp3 tumors exposed to PBS, CoCl2 or DFOM loaded devices. Insets added to show detail. (B) GLUT1 and VEGF-A mRNA expression in the proximal and distal regions to the outlet of the CoCl2-iNANIVIDs (*, p<0.05; ****, p<0.0001). p values calculated with Student's t-test.
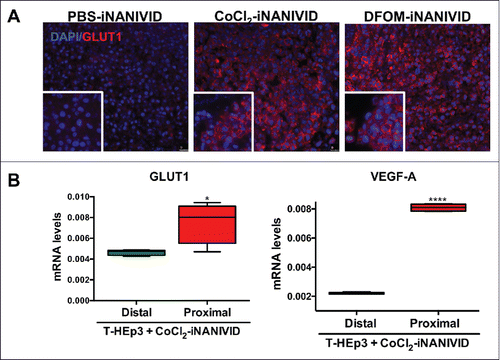
Figure 7. Physiological Effects of iNANIVID. (A) Tissue remains viable days after implantation of device. Histological (H&E) staining of HEp3 tumors after 3 days of being exposed to PBS or DFOM-loaded iNANIVIDs. Insets added to show detail. Neither implant shows signs of necrosis or apoptosis, while the DFOM exposed tissue shows a higher density of blood vessels (arrows). (B) Vascular perfusion near outlet is preserved after insertion of device. Stitched merge of two best-focus 5x stereoscope images showing intravenously injected, fluorescently-labeled, high molecular weight dextran (green signal) in flowing vasculature after insertion of a NANIVID (yellow box) into a mammary tumor. The vessels in the region adjacent to the outlet of the device (asterisk) and within the 512µm field of view that would be captured with multiphoton imaging (red square), are still intact and perfused. (C) Quantification of collagen damage. Collagen fibers near device entrances were imaged in 16 different mice. The distance from the edge of the device (yellow bar – opening indicated by asterisk) to the end of the damaged collagen region is measured (red arrow and line). Distances are plotted in a dot plot (right).

Figure 8. Spatial and temporal extent of the gradient in vivo. (A) Intravital multiphoton image of fluorescently labeled EGF (green signal) diffusing from the outlet (asterisk) of an implanted device (yellow box, 1.3mm wide). Line averaging the EGF signal within the red box produces plots (B-F) showing the onset (B) and (C), the peak at 123 min (D) and the initial decline (E) and (F) of the gradient. (G) Plotting the peak value for each time point shows the temporal extent of the gradient which lasts well in excess of 4 hours.
