Figures & data
Figure 1. Transfer functions of the sixth-order compact Padé filters for various free filtering parameters.
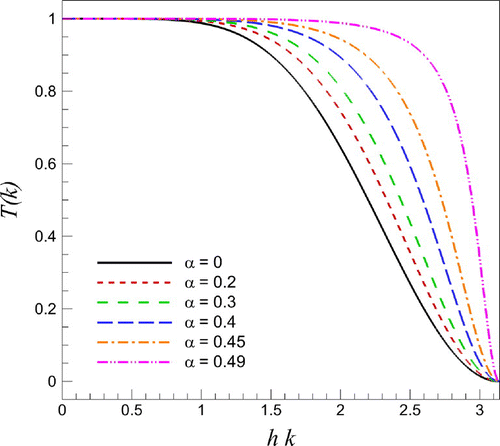
Figure 2. Conservative formulation results for solving the Burgers equation initiated by the single-mode sine wave: (a) time evolution by DNS with resolution of ; (b) under-resolved numerical simulation (UNS); (c) dynamic Smagorinsky model (D-SMA); and (d–f) the proposed dynamic AD model (D-AD) with various orders of Q. In (b–f), we present the final snapshots at time
using resolutions of 512, 1024, and 2048 where the results for 512 and 1024 resolutions are shifted in the x-axis for the purpose of illustration.
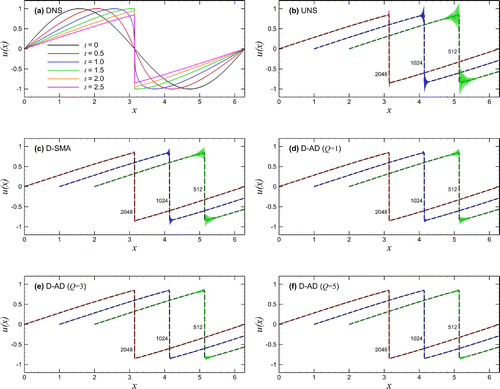
Figure 3. Conservative formulation for the shock formation problem initiated by a single-mode sine wave using 512 grid points: a comparison for the time evolution of the dissipation rate (left) and its close-up for local enlargement (right).
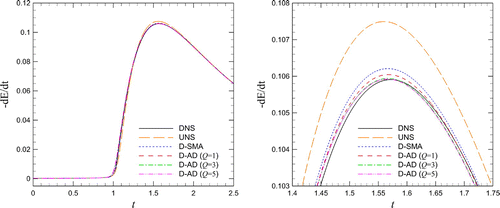
Figure 4. Skew-symmetric formulation simulation results for solving the Burgers equation initiated by the single-mode sine wave: (a) time evolution by DNS with resolution of ; (b) under-resolved numerical simulation (UNS); (c) dynamic Smagorinsky model (D-SMA); and (d–f) the proposed dynamic AD model (D-AD) with various orders of Q. In (b–f), we present the final snapshots at time
using resolutions of 512, 1024, and 2048 where the results for 512 and 1024 resolutions are shifted in the x-axis for the purpose of illustration.
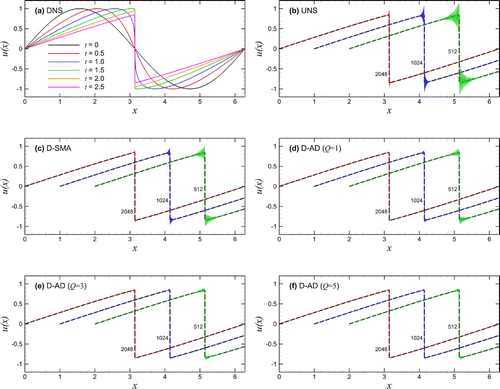
Figure 5. Skew-symmetric formulation for the shock formation problem initiated by a single-mode sine wave using 512 grid points: a comparison for the time evolution of the dissipation rate (left) and its close-up for local enlargement (right).
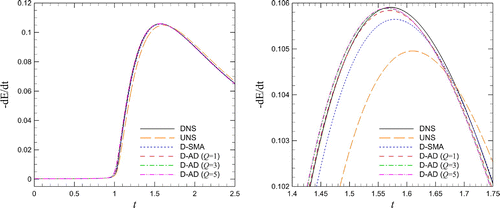
Figure 6. A comparison of energy spectra for resolution results at time
obtained by the conservative (left) and skew-symmetric (right) formulations for the shock formation problem initiated by a single-mode sine wave.
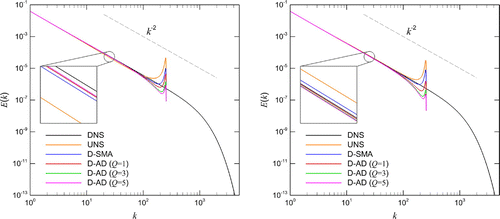
Figure 7. Intercomparison of resolution results for solving the Burgers equation initiated by the single-mode sine wave using the conservative formulation (left column) and the skew-symmetric formulation (right column). Note that the simulation results for UNS, D-SMA, D-AD (
), and D-AD (
) are shifted in x-axis for the purpose of illustration.
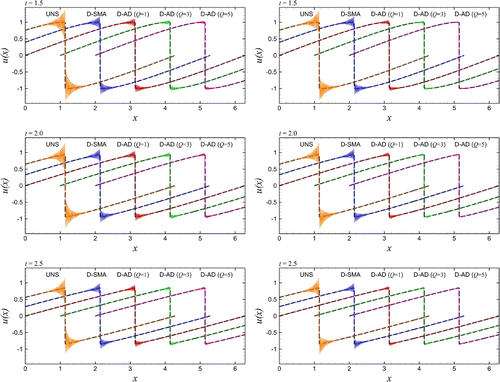
Figure 8. Space–time solutions of the Burgers equation using three different initial conditions generated from Equation (Equation40(40)
(40) ).
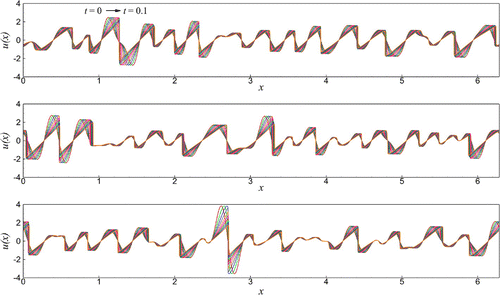
Figure 9. Time evolution of the energy spectrum for the Burgers turbulence problem based upon the ensemble average of 64 sample fields obtained by DNS () (left), and a comparison of energy spectra at time
obtained by various spatial resolutions ranging from
to
(right).
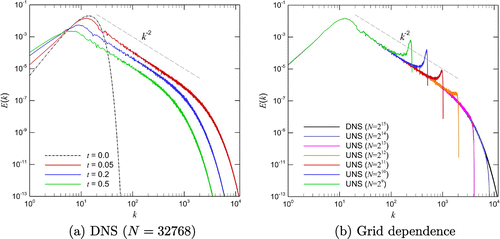
Figure 10. Results for the SGS modeling of the Burgers turbulence problem obtained using resolution with the conservative formulation of the nonlinear term: time evolution of the total dissipation rate (left) and energy spectra at time
(right).
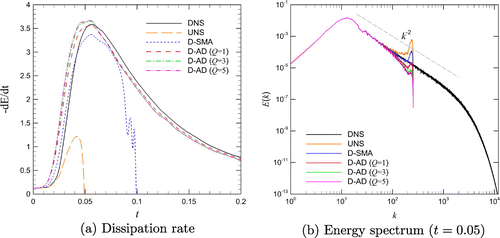
Figure 11. Time evolution of the energy spectrum for the Burgers turbulence problem based upon the ensemble average of 64 sample fields obtained by DNS () (left), and energy spectra at time
obtained by various spatial resolutions ranging from
to
(right).
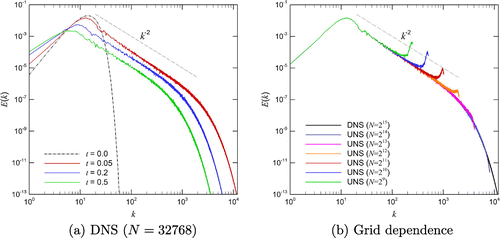
Figure 12. Results for the SGS modeling of the Burgers turbulence problem obtained using resolution with the skew-symmetric formulation of the nonlinear term: time evolution of the total dissipation rate (left) and energy spectra at time
(right).
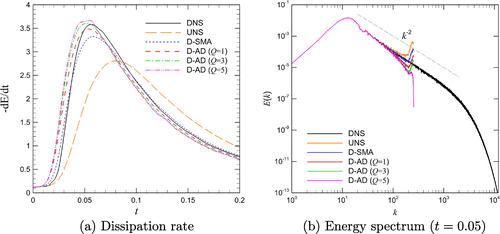