Figures & data
Figure 1. An example of a 2D pin-cell represented by bi-quadratic NURBS patches (macro-elements) in the real physical space, as denoted by the parameters x and y (Wilson, Eaton, and Kópházi Citation2024). The domain, represented in the parametric space,
is formed from the tensor-product of two 1D spaces, as denoted by the parameters
and
The parametric space has been decomposed into non-vanishing knot-spans (micro-elements), over which, numerical integration is performed by an affine mapping from the parent domain,
as denoted by the parameters
and
The patch boundaries are highlighted in red; whereas the non-vanishing knot-spans within a patch are separated by black lines. The image of given non-vanishing knot-span, shaded in dark grey, can be represented in all three spaces. (V. the web-based version for reference to color.).
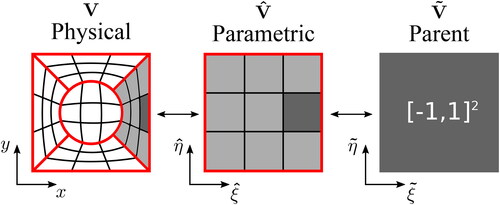
Figure 2. The Jacobian determinant for the example of a 2D unit circle as represented by a single degenerate bi-quadratic NURBS patch (left) and five non-degenerate bi-quadratic NURBS patches (right). The geometrical mapping is non-invertible at the corners of the circle on the left-hand side where the Jacobian determinant becomes negligibly small. (V. the web-based version for reference to color.).
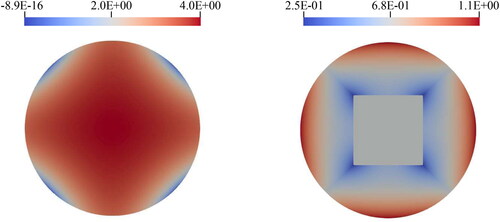
Figure 3. The left and right-hand sides are the result of inserting knots at with multiplicity
respectively, into a basis of quadratic (
) B-splines with no initial internal refinement structure; i.e.,,
In either case, continuity in the basis has been reduced enough to form a new patch boundary,
and the parametric space has been renormalized to
in each of the newly formed patches,
and
C0-continuity across
is naturally enforced in a strong sense for
however, the basis becomes discontinuous, or
-continuous across
for
where it must be explicitly enforced in either a strong sense or a weak one. (V. the web-based version for reference to color.).
![Figure 3. The left and right-hand sides are the result of inserting knots at ξ=0.5 with multiplicity m˙=p,p+1, respectively, into a basis of quadratic (p=2) B-splines with no initial internal refinement structure; i.e.,, Ξξ={0,0,0,1,1,1}. In either case, continuity in the basis has been reduced enough to form a new patch boundary, ∂V̂12, and the parametric space has been renormalized to [0,1] in each of the newly formed patches, V̂1 and V̂2. C0-continuity across ∂V̂12 is naturally enforced in a strong sense for m˙=p; however, the basis becomes discontinuous, or C‐1-continuous across ∂V̂12 for m˙=p+1, where it must be explicitly enforced in either a strong sense or a weak one. (V. the web-based version for reference to color.).](/cms/asset/4bae1fc8-5873-4e8a-80b3-dbc65fe982b2/ltty_a_2334277_f0003_c.jpg)
Figure 4. A comparison between the solutions (right) of an SIP-DG-IGA discretization and its CBG-IGA counterpart along the line at over an irregular mesh (left) for the example of a simple problem; a one-speed, (
) homogeneous bare-square with isotropic unit-source,
c = 0.7. Across 52 bilinear patches, strict C0-continuity enforced in the CBG discretization (73 DoFs), whilst this is relaxed in the DG discretization (208 DoFs). (V. the web-based version for reference to color.).
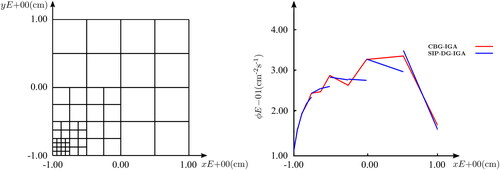
Figure 5. For the example of a 2D unit pin-cell mesh of 13 non-degenerate bi-quadratic NURBS patches (left), a comparison is made between those TICs, and
as calculated from the literature, EquationEquation 69
(69)
(69) , the mass matrix method, EquationEquation 66a
(66a)
(66a) , and the stiffness matrix method, EquationEquation 66b
(66b)
(66b) , respectively. The ten distinct faces are labeled 1 through 10; and all TIC values are normalized by
The distinction between Faces
and
is such that the former is the the single distinct face of the central (red) circle constructed from a single degenerate NURBS patch; whereas the latter belongs to the set of distinct faces that belong to the central circle as it is presented, constructed from five non-degenerate NURBS patches. Those TIC values that are invariant with any change to the radii are presented in the table (right). (V. the web-based version for reference to color.).

Figure 6. A parametric study that compares TIC values for the distinct Faces (top left),
(top right),
(middle left),
(middle right),
(bottom left), and
(bottom right) of a 2D unit pin-cell mesh as the outer-radius is varied; v. . In each instance, the volume patch and the face patches may be defined by
which is varied between 0.05 and 0.45 cm. In the case of
and
is fixed at 0.025 cm. (V. the web-based version for reference to color.).
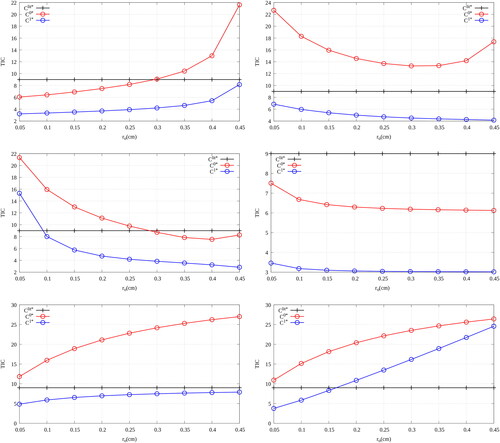
Figure 7. A parametric study that compares TICs for the distinct Faces (left) and
(right) of a 2D unit pin-cell mesh as the number of quadrature points is varied; v. . In each instance, the TICs are invariant with any change to the radii. (V. the web-based version for reference to color.).
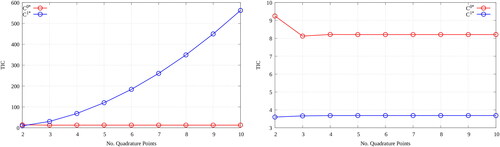
Figure 8. A parametric study of the -error (right) and the spectral conditioning (right) of the SIP-DG-IGA discretization of the 1G NDE for the example of a 2D unit pin-cell mesh; qq.v. and Section 6.1.2. The penalty parameters of each face are varied as
(V. the web-based version for reference to color.).
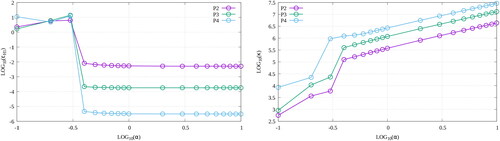
Table 1. The nuclear data for the 2D 1G MMS verification.
Figure 9. The convergence plots, v h (left) and
v DoFs (right), for the MMS verification of the uniform refinement of the SIP-DG-IGA 1G NDE over a 2D Cartesian mesh. The manufactured solution is chosen as per Equation 76 for n = 0 (top row), n = 1 (middle row) and n = 2 (bottom row). (V. the web-based version for reference to color.).
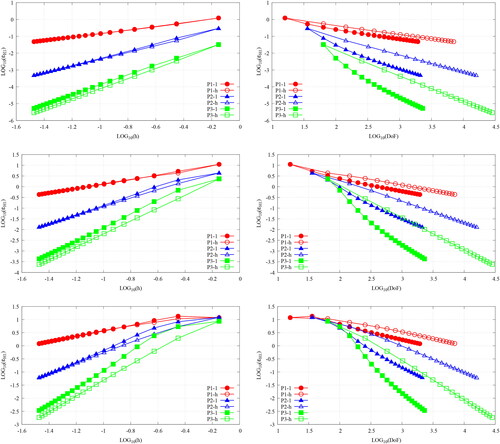
Table 2. The asymptotic orders of accuracy, Equations 74, for the MMS verification of the uniform refinement of the SIP-DG-IGA 1G NDE over a 2D Cartesian mesh.
Figure 10. The convergence plots, v DoFs, for the MMS verification of the NRG-AMR-h(p) of the SIP-DG-IGA 1G NDE over a 2D Cartesian mesh. The manufactured solution is chosen as per Equation 76 for n = 0 (top left), n = 1 (top right) and n = 2 (bottom). (V. the web-based version for reference to color.).
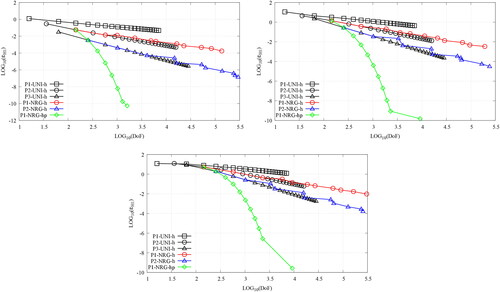
Table 3. The nuclear data for the 2D 2G MMS verification.
Figure 11. The convergence plots, v h (left) and
v DoFs (right), for the MMS verification of the uniform refinement of the SIP-DG-IGA 2G NDE over a 2D pin-cell mesh. The manufactured solutions are chosen as per Equation 77 for g = 1, 2. (V. the web-based version for reference to color.).
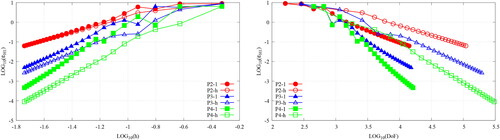
Table 4. The asymptotic orders of accuracy, Equations 74, for the MMS verification of the uniform refinement of the SIP-DG-IGA 2G NDE over a 2D pin-cell mesh.
Figure 12. The convergence plots, v DoFs, for the MMS verification of the NRG-AMR-h(p) of the SIP-DG-IGA 2G NDE over a 2D pin-cell mesh. The prefixes M1- and M2- denote the use of energy-independent and energy-dependent meshes, respectively. The manufactured solutions are chosen as per Equation 77 for g = 1, 2. (V. the web-based version for reference to color.).
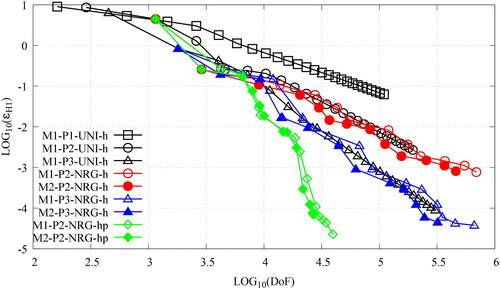
Figure 13. The plots of the effectivity index, v DoFs, for the MMS verification of the NRG-AMR-h(p) of the SIP-DG-IGA 2G NDE over a 2D pin-cell mesh. The prefixes M1- and M2- denote the use of energy-independent and energy-dependent meshes, respectively. The manufactured solutions are chosen as per Equation 77 for g = 1, 2. (V. the web-based version for reference to color.).
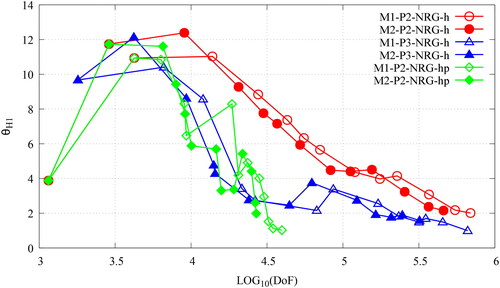
Figure 14. The spatial distributions of the multi-group components of the scalar neutron flux (left) and the scalar neutron importance (right) of the 2G NDE for the 2D IAEA/ANL BSS-11 benchmark. The QoI is the (V. the web-based version for reference to color.).
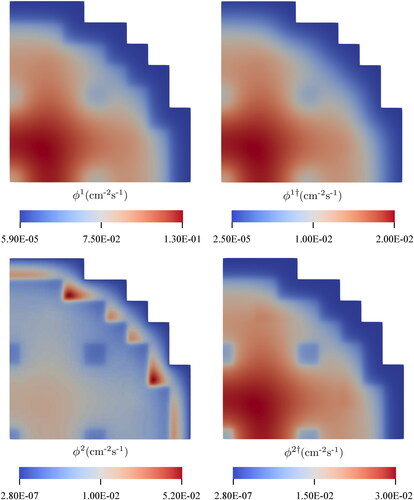
Figure 15. The energy-independent meshes (top) and the energy-dependent meshes (middle and bottom) generated at the AMR-iteration of the NRG-AMR-h (left) and the DWR-AMR-h (right) of the SIP-DG-IGA 2G NDE for the 2D IAEA/ANL BSS-11 benchmark. The QoI is the
(V. the web-based version for reference to color.).
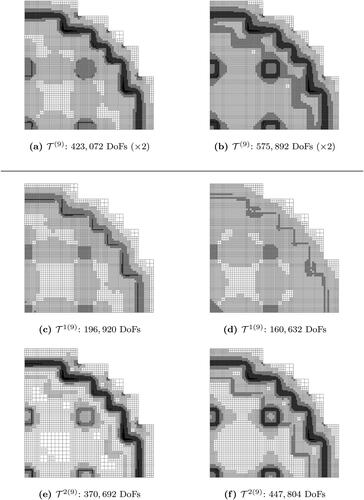
Figure 16. The energy-independent meshes (top) and the energy-dependent meshes (middle and bottom) generated at the AMR-iteration of the NRG-AMR-hp (left) and the DWR-AMR-hp (right) of the SIP-DG-IGA 2G NDE for the 2D IAEA/ANL BSS-11 benchmark. The QoI is the
(V. the web-based version for reference to color.).
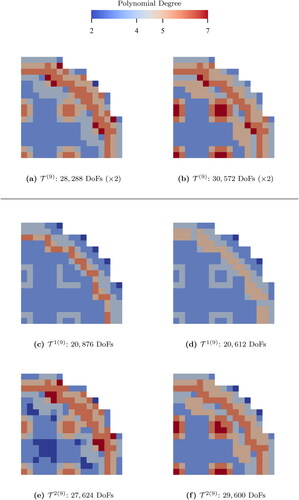
Figure 17. The convergence plots, ϵQoI v DoFs, for the uniform refinement (black), the NRG-AMR (red) and the DWR-AMR (blue) of the SIP-DG-IGA 2G NDE for the 2D IAEA/ANL BSS-11 benchmark. The prefixes M1- and M2- denote the use of energy-independent and energy-dependent meshes, respectively. The QoI is the (V. the web-based version for reference to color.).
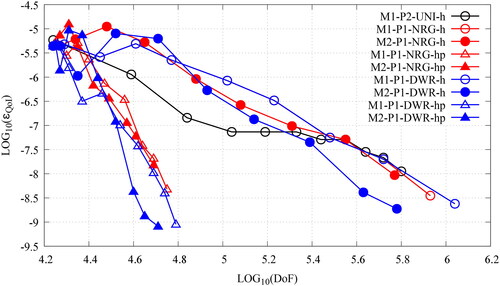
Figure 18. The spatial distributions of the multi-group components of the scalar neutron flux (left) and the scalar neutron importance (right) for the 2G NDE for the 2D BIBLIS benchmark. The QoI is the absorption in Material 5. (V. the web-based version for reference to color.).
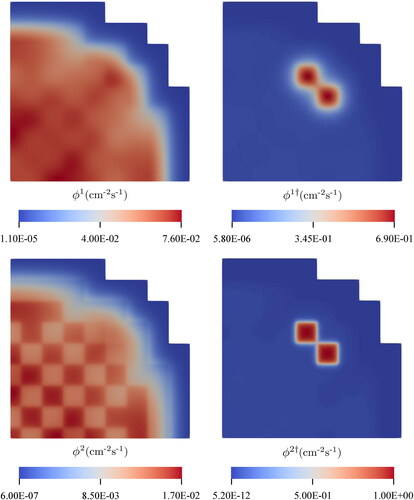
Figure 19. The energy-independent meshes (top) and the energy-dependent meshes (middle and bottom) generated at the AMR-iteration of the NRG-AMR-h (left) and the DWR-AMR-h (right) of the SIP-DG-IGA 2G NDE for the 2D BIBLIS benchmark. The QoI is the absorption in Material 5. (V. the web-based version for reference to color.).
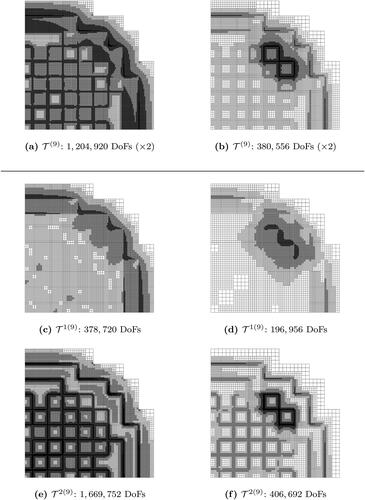
Figure 20. The energy-independent meshes (top) and the energy-dependent meshes (middle and bottom) generated at the AMR-iteration of the NRG-AMR-hp (left) and the DWR-AMR-hp (right) of the SIP-DG-IGA 2G NDE for the 2D BIBLIS benchmark. The QoI is the absorption in Material 5. (V. the web-based version for reference to color.).
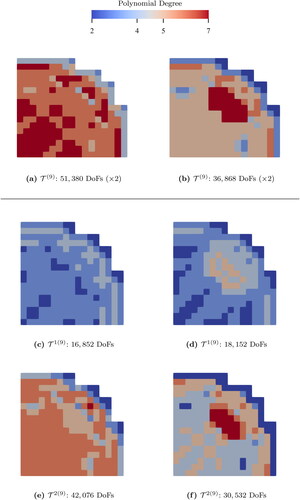
Table 5. The indicative timings for the different stages of analysis at the 9th AMR-iteration of the NRG-AMR-h(p) and DWR-AMR-h(p) of the SIP-DG-IGA 2G NDE over energy-dependent meshes for the 2D BIBLIS benchmark.
Figure 21. The convergence plots, ϵQoI v DoFs, for the uniform refinement (black) and the NRG-AMR (red) and the DWR-AMR (blue) of the SIP-DG-IGA 2G NDE for the 2D BIBLIS benchmark. The prefixes M1- and M2- denote the use of energy-independent and energy-dependent meshes, respectively. The QoI is the absorption in Material 5. (V. the web-based version for reference to color.).
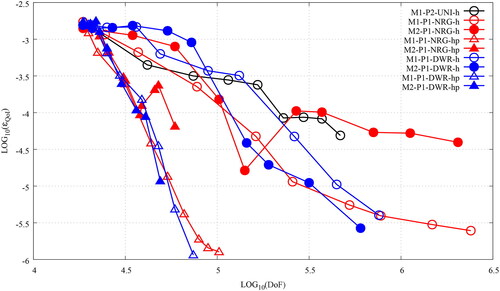
Data statement
In accordance with EPSRC funding requirements, all supporting data used to create figures in this paper may be accessed via the following URL: https://doi.org/10.5281/zenodo.10370345.