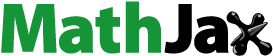
ABSTRACT
1. Xylo-oligosaccharides (XOS) stimulate proliferation of beneficial bacteria in the gastrointestinal tract of broiler chickens. This results in enhanced utilisation of dietary non-starch polysaccharides and increased production of valuable short-chain fatty acids. However, these positive effects do not always translate into improved bird productive performance, with inconsistent performance responses observed between bird trials.
2. A holo-analysis was conducted to determine the effects of supplementing XOS into broiler diets on bird feed intake, body weight gain, feed conversion and mortality. This was done by comparing the XOS supplemented treatment to the control treatment. A total of 53 studies which met the criteria for inclusion were used in the analysis.
3. The results showed that XOS had a notable positive impact on bird mortality; XOS reduced mortality by 0.69% for every 1% increment in the control group. XOS supplementation induced a positive effect on the feed conversion ratio (FCR). However, the efficacy of XOS at improving FCR was dependent on the efficiency of the control group (performance of the flock), and the concentration of total arabinoxylan, protein and phytase in the diet. There were insufficient data points to predict the effect of XOS on body weight and feed intake.
4. In conclusion, the holo-analysis revealed that supplementing XOS to broiler chicken diets reduces bird mortality. XOS can also improve FCR, but the scale of response is dependent on the diet composition and control flock performance. Additional studies are required to confirm the effects of XOS on body weight and feed intake.
Introduction
Xylo-oligosaccharides (XOS) are xylose polymers produced by hydrolytic degradation of xylan by endo-xylanases (Moure et al. Citation2006). The XOS possess prebiotic properties, whereby they selectively stimulate the growth of beneficial bacteria, resulting in decreased pathogenic bacteria colonisation in the gastrointestinal tract (GIT) and increased production of volatile fatty acids (VFA) and lactic acid, by enhancing fermentation of non-starch polysaccharides (NSP; Courtin et al. Citation2008; Keerqin et al. Citation2017; Morgan et al. Citation2022; Ribeiro et al. Citation2018). The XOS induce these positive effects when fed at very low doses, 10–100-fold lower than is required by other functional oligosaccharides (González-Ortiz et al. Citation2021; Vázquez et al. Citation2000). This has led to the development of the term ‘stimbiotics’, which is defined as a product that accelerates the development of an NSP-degrading microbiome when added in very small quantities. This effect is driven by the stimbiotic selectively stimulating bacteria that produce endogenous NSP-degrading enzymes. The difference between prebiotics and stimbiotics is that prebiotics are supplemented at high doses (kilograms per tonne) and are rapidly and quantitatively fermented to short-chain fatty acids (SCFA) in the caeca and ileum, whereas stimbiotics are supplemented at very low doses (g/t) and do not directly promote significant fermentation, with effects taking several days or more to materialise (Bedford and Apajalahti Citation2022). Hence, XOS act as both prebiotics and stimbiotics.
Xylanase application in poultry diets is common practice, to combat the anti-nutritional effect of dietary xylan on digesta viscosity and entrapment of nutrients (Baker et al. Citation2021). The XOS are produced in situ in the GIT, in the presence of both endogenous and exogenous xylanases. Recently there has been increased interest in supplementing XOS directly into poultry diets. The benefit of this is that it ensures the microbiota receive a consistent supply, as opposed to being dictated by the xylan content of the diet and efficacy of supplemental xylanase. The increased thermostability of XOS compared to other oligosaccharides or xylanases (Vázquez et al. Citation2000) mean they can be included before pelleting without loss of efficacy (Courtin et al. Citation2009). Combining XOS and xylanase together has been shown to be beneficial (González-Ortiz et al. Citation2021; Morgan, Gomes, and Kim Citation2021) by both supplying ample XOS to stimulate and fuel the beneficial microbiota and eliminating the negative effects of dietary xylan, respectively. However, data on the effects of combined XOS and xylanase is equivocal, thus the value of such a combination needs more robust establishment.
In published literature, supplemental XOS doses range from as low as 2 g/t (Yuan et al. Citation2018) to 25–100 g/t (Singh et al. Citation2021; Suo et al. Citation2015), 1000–5000 g/t (Bautil et al. Citation2020; Courtin et al. Citation2008; Craig et al. Citation2020; De Maesschalck et al. Citation2015; Morgan et al. Citation2022; Samanta et al. Citation2017), and as high as 20 000 g/t (Zhenping et al. Citation2013). There is a lack of consistency between studies regarding broiler performance responses to dietary XOS. For example, supplementing 5000 g/t XOS to wheat-based diets resulted in improved feed conversion (FCR) in studies run by De Maesschalck et al. (Citation2015) and Courtin et al. (Citation2008), but no performance effect was observed by Bautil et al. (Citation2020). In corn-based diets, Suo et al. (Citation2015) found supplementing XOS at 100 g/t had a positive impact on FCR, but Singh et al. (Citation2021) saw no effect. This variability could have been associated with the different physiochemical properties and structures of the XOS used or the small animal numbers used in some individual studies. One way to understand the efficacy of any active supplement is by conducting a holo-analysis. This involves a fully comprehensive statistical analysis of all available data and variables, which allow for identification of factors (breed, sex, age, feed materials) that influence responses to feed products, which could never be recognised in a single trial. As recently shown by Dale and Bedford (Citation2023), holo-analysis avoids subjective interpretations and bias in the selection of papers and data filtering by authors when reviewing a topic generally or, for example, in meta-analysis. Holo-analyses identify these factors and help with the interpretation of conflicting results between different studies.
The aim of the following study was to collect performance data from multiple published trials that tested the effects of supplemental XOS in broiler diets, and use this data to quantify the effects of XOS on broiler body weight gain, feed intake, FCR and mortality.
Materials and methods
Data collection
A systematic literature review was conducted in May 2023 using the Web of Science, Google Scholar and PubMed databases, and searching the journals Animal Nutrition, Poultry Science and British Poultry Science. No temporal or language restrictions were applied to the searches. Keyword combinations were used to identify articles, including poultry, broiler, xylo-oligosaccharides, XOS, xylan and xylanase, to form the initial database. Information from AB Vista’s internal dataset was included as this contained performance data from every broiler chicken study run globally by AB Vista that tested the effects of XOS supplementation.
Exclusion criteria were applied to the complete dataset to determine the studies that would be included in the final holo-analysis. Studies were removed from the dataset if they did not use broiler chickens (Ross, Cobb or Arbor Acres), directly supplement XOS into the diet, have a control diet without XOS, feed from hatch, provide body weight gain and feed intake data, present the ingredient composition of the diets, state the concentration of XOS supplemented into the diet or use birds free from disease challenge. This resulted in 174 total observations being included in the final database, where an observation was the effect of the XOS treatment on the parameter of interest when compared with the relevant control. These were from 53 different sources, 52 observations from the literature search and 122 from the AB Vista internal database, covering a 15-year period, from 2007 to 2022.
The presence of supplemental xylanase and phytase was recorded in the database, as was the level of corn, wheat, soybean meal (SBM), sorghum, rye, sunflower meal, canola meal, corn gluten meal, wheat bran, wheat germ, barley, DDGS and full-fat canola in the diet. Recorded information included the analysed and/or calculated metabolisable energy, gross energy, digestible lysine, protein on an as-is basis, and NSP, arabinoxylan (AX) and fibre content of the diet on a dry matter basis. If not presented, total, insoluble, and soluble NSP and total AX content of the diets were predicted, based on the ingredient composition and average NSP and AX values for these ingredients (Nguyen et al. Citation2022).
Data analysis
The data was compiled in a Microsoft Excel spreadsheet, with each treatment representing a separate row of data in the database. The spreadsheet included columns listing author or trial reference, country, journal or internal trial code from AB Vista, year, dietary phases (including number of days per phase), supplemented XOS dose (as a product), diet composition and nutrient parameters, whether birds were ad libitum fed, breed and sex of birds, body weight gain (BWG), feed intake (FI), mortality and feed conversion ratio (FCR). The effect of XOS on broiler chicken BWG, FI, FCR and mortality was determined by comparison to the relevant control treatment, thus calculating the delta (δ) number. In some studies, there was more than one control diet, yielding multiple comparisons from one study.
Data analysis was performed using JMP Pro 15 (SAS) statistical software following Dale and Bedford (Citation2023) procedures for data-driven reviews with holo-analysis. Descriptive statistics were summarised, and the normal distribution of the continuous variables and identification of outliers was carried out before analysis of the data. Firstly, principal component analysis was performed to identify the collinearity of the continuous variables. Secondly, decision trees were applied to identify the factors that most influenced the predicted delta BWG, FI, FCR and mortality. Once factors were identified, regression models were applied through the Standard Least Squares Restricted Maximum Likelihood (REML) method, including the source of information as a random effect. Models were cross validated five times (A, B, C, D and E) by splitting out 60% of the observations for training and using the remaining 40% of the observations under validation. The closer the R2 of the validated model was to that of the training model, the more reliable the model was, reducing the likelihood of any model being caused randomly. A validation of less than 50% of the R2 of the training model was considered an unreliable predicting model. Both the training and validation model had their own unique random noise, but the Root Average Squared Error (RASE) differences between the training and the validated models were used to assess reliability of the models (Grayson, Grayson et al. Citation2015; Klimberg and McCullough Citation2016).
Results
Descriptive results
shows a summary of key information included in the holo-analysis. Data was primarily obtained from the internal trials sponsored by AB Vista, with 30% from peer-reviewed manuscripts. Most studies used pelleted diets (75%) and fed the birds ad libitum (97%). Ross was the main chicken breed (60%) and mainly male birds (83%) were used. The top five countries where XOS effects have been evaluated in broiler chickens since 2007 were UK (25%), US (16%), Finland (16%) and Australia (8%).
Figure 1. All nominal variables included in the prediction models were based on 53 sources of information (literature and internal reports) and 174 observations.

As highlighted in , the median bird trial ran for 42 d (average of 37.6 d), ranging from a minimum of 10 d to a maximum of 59 d. The average number of replicates per treatment was 7.6, ranging from 3 to 16. Most trials contained corn as the sole cereal source, or corn combined with wheat, resulting in a median of 56.6% for dietary corn inclusion. Xylanase was supplemented in 53% of the studies, and, when supplemented, xylanase activity ranged from 9,600 to 22 700 BXU/kg. Median phytase activity in the test diets was 500 FTU/kg.
Table 1. Minimum, mean and maximum values of the most relevant independent variables in all trials evaluating the efficacy of xylo-oligosaccharides in broiler chickens.
Effect of XOS on mortality
Average mortality for the control treatments was 4.91% and ranged from 0% to 18.13% (). Supplementing with XOS reduced mortality by 0.69% for every 1% increment in mortality recorded in the control group, as illustrated by the δMortality (). The optimal regression model for δMortality, based on 84 observations, explained 50% of the variation. Despite this limited number of observations, the R2 of the validation test matched 68% of the training test (0.407 vs. 0.601), meaning this was a reliable and robust prediction model.
Figure 2. Mortality records; a) Mortality (as %) reported in the control treatment across all studies included in the holo-analysis; b) Delta mortality (δ) presented as the difference between the relevant control treatment and the XOS treatment for each study and comparison. The right side of each graph shows the summary statistics for each parameter, presenting the mean, the standard error of the mean (SEM), coefficient of variation (CV), the maximum, median and minimum levels, and the number of observations recorded.

Figure 3. Reduction in mortality by xylo-oligosaccharides (%, y-axis) as a function of control mortality (%, x-axis).

Mortality in the control birds (cMOR) was the main and unique significant (P < 0.05) factor influencing δMortality ().
Table 2. Prediction models for the effect of xylo-oligosaccharides (XOS) on broiler chicken mortality.
Thus, it is possible to predict the influence of XOS on mortality when cMOR was provided using the following equation:
Effect of XOS on performance
Effects of XOS on overall performance is presented in . Generally, XOS supplementation had a positive impact on broiler performance, but the magnitude of this response varied considerably between individual trials, illustrated by the large CV values (δBWG = 334%; δFI = 748%; δFCR = −300%).
Table 3. Overall performance results of the control treatments and delta observations from all trials evaluating the efficacy of xylo-oligosaccharides in broiler chickens.
Average δBWG and δFI were 31 g/bird and 23 g/bird, respectively, but it was more relevant to look at the predictive models, due to high variation (). The best regression models for BWG and FI explained 76% and 71% of the variation, respectively. The dose of XOS (logXOS), xylanase supplementation, phytase supplementation, apparent metabolisable energy and corn inclusion were factors that influenced variability the most (data not shown). However, when the models were cross validated, the average R2 of the validated models did not reach 50% of the R2 of the training models (0.228 vs. 0.715 for BWG; 0.228 vs. 0.724 for FI). This lack of validation meant that the models derived from the current dataset were not robust enough to predict the efficacy of XOS for these parameters. Therefore, the factors selected in the models were deemed irrelevant. Additional data could help with validating the models, allowing for determination of the effects of XOS on BWG and FI.
Table 4. Prediction models for the effect of xylo-oligosaccharides (XOS) on broiler chickens body weight gain (BWG) and feed intake (FI).
Average δFCR was −0.017, and a total of 151 observations were used to predict the effect of XOS supplementation on δFCR (). The best regression model to predict δFCR explained 84% of the variation, with concentration of protein and total AX in the diet, level of supplemental phytase, and FCR of the control birds proving to be the most influencing factors (P < 0.07).
Table 5. Prediction models for the effect of xylo-oligosaccharides (XOS) on broiler chickens feed conversion ratio (FCR).
When the models were cross-validated the R2 of the validation test matched 50% of the training test (0.438 vs. 0.872), meaning that this model was at the threshold of being considered a reliable prediction model, and significant effects could be discussed with confidence. The influence of XOS on broiler FCR can be predicted using the following the equation:
This suggested that the beneficial effects of XOS on FCR were greater when the FCR of the control diet was high and the diet was rich in total AX. The efficacy of XOS was reduced in high protein diets or those supplemented with higher levels of phytase.
Discussion
Supplementing XOS reduced mortality and improved FCR, despite large variability between individual studies. A total of 84 observations were used to predict δMortality. The number of data points used was below the recommended minimum of 100 observations (Pesti et al. Citation2009), yet positive correlations between XOS and mortality were still observed. To the best of the authors’ knowledge, this is the first time a holistic approach has been used to determine the ability of XOS to reduce mortality in broilers. A possible explanation for this finding was that XOS reduced the prevalence of pathogenic bacteria in the GIT, such as Clostridium spp. and Salmonella spp. and induced bacteriostatic actions against other pathogens, such as Vibrio spp. (Zhou et al. Citation2009). Enhanced immunologic competence has previously been observed with supplemental XOS application, which increased resistance to pathogenic bacteria colonisation (De Maesschalck et al. Citation2015; Luo et al. Citation2021; Pourabedin et al. Citation2017), and expression of protective mucin glycoprotein in intestinal epithelial cells (Yuan et al. Citation2018). Thus, it was predicted that the reduced mortality was a consequence of XOS improving gastrointestinal health. Further data points are needed to confirm XOS mechanisms of action, and bird response to XOS under disease-challenged conditions. Moreover, it should be noted that purity of the XOS products is rarely reported. It was predicted that most of the studies included in the holo-analysis tested XOS with approximately 35% purity, but it was not possible to confirm this. Future trials should therefore include XOS purity.
One of the goals of this holistic study was to identify the predictive factors influencing the efficacy of XOS on BWG and FI. Xylanase level was one which seemed to influence the efficacy of XOS on δBWG, with increased response observed in the presence of xylanase. Unfortunately, it was not possible to validate the models for these performance parameters with confidence using the current dataset. There were more than the required 100 observations (Pesti et al. Citation2009), but variability between individual studies was too high to allow derivation of robust models. This highlighted the importance of viewing data from a holistic perspective, rather than relying on overall means and correlation coefficients to confirm the efficacy of a test product. For example, Rysman et al. (Citation2023) tried to associate the relationship between gut morphology parameters and bird performance from a highly populated dataset using multivariate analysis. However, their outcomes relied on correlation coefficients without considering that correlation (r) does not mean causation (R2), which can result in misleading conclusions. More data points are needed to confirm if the factors identified could predict the efficacy of XOS on BWG and FI.
Control group FCR and total dietary AX content dictated the efficacy of XOS on FCR. This was attributed to ability of XOS to favourably manipulate GIT microbiota composition. Rinttilä and Apajalahti (Citation2013) observed that low performing birds (with high FCR and low metabolisable energy utilisation) have a different microbiota composition compared to higher performing birds. Birds with poor FCR had lower representation of groups such as Lactobacillus salivarius, Ruminococcus spp. and Faecalibacterium prausnitzii, while top performing birds showed higher counts of these phylotypes, alongside Clostridiales and Lachnospiraceae. It has been shown that XOS can promote the colonisation of the GIT with some of these phylotypes (De Maesschalck et al. Citation2015), establishing a more beneficial microbiota and enhancing diet utilisation, particularly in inefficient birds. Indeed, Morgan et al. (Citation2019) found that dietary supplementation of arabinoxylo-oligosaccharides improved the net utilisation of dietary energy and bird performance, credited to stimulation of beneficial bacteria and SCFA production. Increased XOS efficacy in the presence of high dietary AX may be because the stimbiotic effect of supplementation facilitated significantly more AX fermentation than could ever be elicited in a low AX diet (Morgan et al. Citation2022). Supporting this theory, Singh et al. (Citation2021) increased total AX content of a corn-SBM diet through the addition of wheat bran. Xylanase supplementation resulted in in situ production of XOS in the GIT, which directly improved growth performance and SCFA (VFA and lactic acid) concentrations, and relative abundance of fibre-utilising bacteria in the caeca. The SCFA provide energy and a carbon source to the bird, which is used particularly for the development of the gastrointestinal tract lining, improving intestinal health and increasing nutrient absorption (Craig et al. Citation2020; Ding et al. Citation2018; Sun et al. Citation2021). For example, De Maesschalck et al. (Citation2015) showed that XOS fuels Lactobacilli, resulting in increased lactic acid production. Lactic acid can be consumed by butyrate-producing bacteria, increasing intestinal butyrate concentrations which improves gut intestinal integrity (Mátis et al. Citation2022), enhancing digestion and nutrient absorption (Li et al. Citation2017; Marinho et al. Citation2007). Feeding diets with high soluble AX content and XOS to birds from an early age means that these birds will likely have a microbiota that is better adapted to utilising xylan as the bird ages (Bautil et al. Citation2020). Results from this holo-analysis supported this observation.
Increased dietary protein levels negatively influenced the efficacy of XOS on δFCR. It may be that XOS is not able to induce effects in birds fed a high protein diet, as bird requirements are already met, but, in low protein diets, XOS improves amino acid digestion, primarily through peptide tyrosine-tyrosine (PYY) gizzard feedback mechanisms (Herwig et al. Citation2020). Another possible explanation is that there may have been more undigested protein in the gastrointestinal tract of birds fed the protein-rich diets, which fuelled pathogenic bacteria abundance (Elling-Staats et al. Citation2022). A consequence of this is reduced ability of beneficial bacteria to flourish, including XOS-utilising species. This means that XOS supplementation may have fuelled pathogenic bacteria species or have been wasted, eliminating its ability to enhance bird gastrointestinal health and performance. Heightened protein fermentation by putrefactive bacteria, particularly in the caeca, may result in production of harmful and toxic compounds, such as ammonia, phenols and amines, which generally reduce host health (Apajalahti and Vienola Citation2016). It may be that the XOS was not efficient enough to combat these effects, or that the supplemented levels were too low, as putrefaction becomes the dominant type of fermentation when there is insufficient carbohydrate in the distal intestine. Given that bacteria preferentially ferment carbohydrates, it may be that it is necessary to feed a higher dose of XOS, or XOS combined with other oligosaccharides and/or NSP-degrading enzymes when feeding high protein diets, to reduce putrefaction in the caeca.
The efficacy of XOS on δFCR was reduced by increasing phytase level. This was likely because phytase increases protein digestibility (Cowieson et al. Citation2017), again limiting the ability of XOS to induce any notable impacts on amino acid digestion and bird performance. Supplying XOS reduces GIT pH, through generation of SCFA, but phytase increases pH (Selle et al. Citation2023). Pathogenic bacteria are acid-sensitive, suggesting presence of a higher pH meant they could proliferate and induce detrimental effects on bird health (Fathima et al. Citation2022). However, Shang and Kim (Citation2017) concluded that fructo-oligosaccharides and phytase have a synergistic relationship, with the lower pH induced by the oligosaccharides providing an optimum environment for the phytase. In the absence of phytase, XOS may counteract the deleterious effects of phytic acid through increasing caecal mineral absorption and stimulating bacteria that can ferment phytate. This is lessened in the presence of phytase, diluting the observed benefits of XOS. Further research is warranted into the synergistic effects of prebiotics and phytase.
In conclusion, holo-analysis revealed that supplementing XOS into broiler diets reduced bird mortality and improved FCR. It identified certain factors that should be considered when the goal is to optimise broiler response to XOS. As this was a preliminary holistic evaluation, the models constructed will evolve as additional data is derived and added to the body of information in the future. However, it is likely that the directional variables have already been identified. The general level of performance of the flock and dietary levels of protein, phytase and total AX directly influences the efficacy of XOS as a performance-enhancing feed ingredient.
Disclosure statement
No potential conflict of interest was reported by the author(s).
References
- Apajalahti, J., and K. Vienola. 2016. “Interaction between chicken intestinal microbiota and protein digestion.” Animal Feed Science and Technology 221:323–330. https://doi.org/10.1016/j.anifeedsci.2016.05.004.
- Baker, J. T., M. E. Duarte, D. M. Holansa, and S. W. Kim. 2021. “Friend or Foe? Impacts of Dietary Xylans, Xylooligosaccharides, and Xylanases on Intestinal Health and Growth Performance of Monogastric Animals.” Animals 11 (3): 609. https://doi.org/10.3390/ani11030609.
- Bautil, A., J. Verspreet, J. Buyse, P. Goos, M. R. Bedford, and C. M. Courtin. 2020. “Arabinoxylan-Oligosaccharides Kick-Start Arabinoxylan Digestion in the Aging Broiler.” Poultry Science 99:2555–2565. https://doi.org/10.1016/j.psj.2019.12.041.
- Bedford, M. R., and J. H. Apajalahti. 2022. “The Role of Feed Enzymes in Maintaining Poultry Intestinal Health.” Journal of the Science of Food and Agriculture 102 (5): 1759–1770. https://doi.org/10.1002/jsfa.11670.
- Courtin, C. M., W. F. Broekaert, K. Swennen, O. Lescroart, O. Onagnesan, J. Buyse, E. Decuypere, et al. 2008. “Dietary Inclusion of Wheat Bran Arabinoxylooligosaccharides Induces Beneficial Nutritional Effects in Chickens.” Cereal Chemistry 85 (5): 607–613. https://doi.org/10.1094/CCHEM-85-5-0607.
- Courtin, C. M., K. Swennen, P. Verjans, and J. A. Delcour. 2009. “Heat and pH stability of Prebiotic Arabinoxylooligosaccharides, Xylooligosaccharides and Fructooligosaccharides.” Food Chemistry 112 (4): 831–837. https://doi.org/10.1016/j.foodchem.2008.06.039.
- Cowieson, A. J., J.-P. Ruckebusch, J. O. B. Sorbara, J. W. Wilson, P. Guggenbuhl, and F. F. Roos. 2017. “A Systematic View on the Effect of Phytase on Ileal Amino Acid Digestibility in Broilers.” Animal Feed Science and Technology 225:182–194. https://doi.org/10.1016/j.anifeedsci.2017.01.008.
- Craig, A. D., F. Khattak, P. Hastie, M. R. Bedford, and O. A. Olukosi. 2020. “Xylanase and Xylo- Oligosaccharide Prebiotic Improve the Growth Performance and Concentration of Potentially Prebiotic Oligosaccharides in the Ileum of Broiler Chickens.” British Poultry Science 61 (1): 70–78. https://doi.org/10.1080/00071668.2019.1673318.
- Dale, T., and M. R. Bedford. 2023. “Reviewing the Literature on Feed Additives: Considerations in Data Collection and Data Analysis (Chapter 8).” In Pre and Probiotics for Poultry Gut Health, edited by H. Masey O’Neill, E. Burton, and D. Scholey, 108–127. Wallingford, Oxfordshire, UK: CABI International.
- de Maesschalck, C., V. Eeckhaut, L. Maertens, L. de Lange, L. Marchal, C. Nezer, S. D. Baere, S. Croubels, G. Daube, and J. Dewulf. 2015. “Effects of Xylo-Oligosaccharides on Broiler Chicken Performance and Microbiota.” Applied & Environmental Microbiology 81 (17): 5880–5888. https://doi.org/10.1128/AEM.01616-15.
- Ding, X., D. Li, S. Bai, J. Wang, Q. Zeng, Z. Su, Y. Xuan, and K. Zhang. 2018. “Effect of Dietary Xylooligosaccharides on Intestinal Characteristics, Gut Microbiota, Cecal Short-Chain Fatty Acids, and Plasma Immune Parameters of Laying Hens.” Poultry Science 97 (3): 874–881. https://doi.org/10.3382/ps/pex372.
- Elling-Staats, M. L., M. S. Gilbert, H. Smidt, and R. P. Kwakkel. 2022. “Caecal protein fermentation in broilers: a review.” World’s Poultry Science Journal 78 (1): 102–123. https://doi.org/10.1080/00439339.2022.2003170.
- Fathima, S., R. Shanmugasundaram, D. Adams, and R. K. Selvaraj. 2022. “Gastrointestinal Microbiota and Their Manipulation for Improved Growth and Performance in Chickens.” Foods 11 (10): 1401. https://doi.org/10.3390/foods11101401.
- González-ortiz, G., T. T. dos Santos, and M. R. Bedford. 2021. “Evaluation of Xylanase and a Fermentable Xylo-Oligosaccharide on Performance and Ileal Digestibility of Broiler Chickens Fed Energy and Amino Acid Deficient Diets.” Animal Nutrition 7 (2): 488–495. https://doi.org/10.1016/j.aninu.2020.07.008.
- Grayson, J., S. Gardner, and M. L. Stephens. 2015. Building Better Models with JMP® Pro. Cary, NC: SAS Institute Inc.
- Herwig, E., K. Schwean-Lardner, A. van Kessel, R. K. Savary, H. L. Classen, and S.-B. Wu. 2020. “Assessing the Effect of Starch Digestion Characteristics on Ileal Brake Activation in Broiler Chickens.” PLoS ONE 15 (2): e0228647. https://doi.org/10.1371/journal.pone.0228647.
- Keerqin, C., N. K. Morgan, S. Wu, R. A. Swick, and M. Choct. 2017. “Dietary Inclusion of Arabinoxylo-Oligosaccharides in Response to Broilers Challenged with Subclinical Necrotic Enteritis.” British Poultry Science 58 (4): 418–424. https://doi.org/10.1080/00071668.2017.1327705.
- Klimberg, R., and B. D. McCullough. 2016. Fundamentals of Predictive Analysis with JMP®. 2nd ed. Cary, NC: SAS Institute Inc.
- Li, D., X. Ding, K. Zhang, S. Bai, J. Wang, Q. Zeng, Z. Su, and L. Kang. 2017. “Effects of Dietary Xylooligosaccharides on the Performance, Egg Quality, Nutrient Digestibility and Plasma Parameters of Laying Hens.” Animal Feed Science and Technology 225:20–26. https://doi.org/10.1016/j.anifeedsci.2016.12.010.
- Luo, D., J. Li, T. Xing, L. Zhang, and F. Gao. 2021. “Combined Effects of Xylo-Oligosaccharides and Coated Sodium Butyrate on Growth Performance, Immune Function, and Intestinal Physical Barrier Function of Broilers.” Animal Science Journal 92 (1): e13545. https://doi.org/10.1111/asj.13545.
- Marinho, M. C., M. M. Lordelo, L. F. Cunha, and J. P. B. Freire. 2007. “Microbial Activity in the Gut of Piglets: 1. Effect of Prebiotic and Probiotic Supplementation.” Livestock Science 108 (1): 236–239. https://doi.org/10.1016/j.livsci.2007.01.081.
- Mátis, G., M. Mackei, B. Boomsma, H. Fébel, K. Nadolna, L. Szymański, J. E. Edwards, Z. Neogrády, and K. Kozłowski. 2022. “Dietary Protected Butyrate Supplementation of Broilers Modulates Intestinal Tight Junction Proteins and Stimulates Endogenous Production of Short Chain Fatty Acids in the Caecum.” Animals 12 (15): 1940. https://doi.org/10.3390/ani12151940.
- Morgan, N. K., G. A. Gomes, and J. C. Kim. 2021. “Comparing the Efficacy of Stimbiotic and a Combination of Xylanase and Beta-Glucanase, in Broilers Fed Wheat-Barley Based Diets with High or Low AME.” Poultry Science 100 (10): 101383. https://doi.org/10.1016/j.psj.2021.101383.
- Morgan, N. K., C. Keerqin, A. Wallace, S.-B. Wu, and M. Choct. 2019. “Effect of Arabinoxylo-Oligosaccharides and Arabinoxylans on Net Energy and Nutrient Utilization in Broilers.” Animal Nutrition 5 (1): 56–62. https://doi.org/10.1016/j.aninu.2018.05.001.
- Morgan, N. K., A. Wallace, and M. R. Bedford. 2022. “Improving sorghum digestion in broilers by targeting fermentation of xylan.” Animal Nutrition 10:198–206. https://doi.org/10.1016/j.aninu.2022.03.004.
- Moure, A., P. gullón, H. Domínguez, and J. C. Parajó. 2006. “Advances in the Manufacture, Purification and Applications of Xylo-Oligosaccharides as Food Additives and Nutraceuticals.” Process Biochemistry 41 (9): 1913–1923. https://doi.org/10.1016/j.procbio.2006.05.011.
- Nguyen, H. T., M. R. Bedford, S.-B. Wu, and N. K. Morgan. 2022. “Dietary Soluble Non-Starch Polysaccharide Level Influences Performance, Nutrient Utilisation and Disappearance of Non-Starch Polysaccharides in Broiler Chickens.” Animals 12 (5): 547. https://doi.org/10.3390/ani12050547.
- Pesti, G. M., D. Vedenov, J. A. Cason, and L. Billard. 2009. “A Comparison of Methods to Estimate Nutritional Requirements from Experimental Data.” British Poultry Science 50 (1): 16–32. https://doi.org/10.1080/00071660802530639.
- Pourabedin, M., Q. Chen, M. Yang, and X. Zhao. 2017. “Mannan-And Xylooligosaccharides Modulate Caecal Microbiota and Expression of Inflammatory-Related Cytokines and Reduce Caecal Salmonella Enteritidis Colonisation in Young Chickens.” FEMS Microbiology Ecology 93 (1): fiw226. https://doi.org/10.1093/femsec/fiw226.
- Ribeiro, T., V. Cardoso, L. Ferreira, M. Lordelo, E. Coelho, A. Moreira, M. Domingues, M. Coimbra, M. Bedford, and C. Fontes. 2018. “Xylo-Oligosaccharides Display a Prebiotic Activity When Used to Supplement Wheat or Corn-Based Diets for Broilers.” Poultry Science 97 (12): 4330–4341. https://doi.org/10.3382/ps/pey336.
- Rinttilä, T., and J. Apajalahti. 2013. “Intestinal Microbiota and Metabolites—Implications for Broiler Chicken Health and Performance.” Journal of Applied Poultry Research 22 (3): 647–658. https://doi.org/10.3382/japr.2013-00742.
- Rysman, K., V. Eechaut, R. Ducatelle, E. Goosens, and F. van Immerseel. 2023. “Broiler Performance Correlates with Gut Morphology and Intestinal Inflammation Under Field Conditions.” Avian Pathology 52 (4): 232–241. https://doi.org/10.1080/03079457.2023.2201169.
- Samanta, A., A. Kotte, A. Elangovan, A. Dhali, S. Senani, M. Sridhar, and N. Jayapal. 2017. “Effects of Corn Husks Derived Xylooligosaccharides on Performance of Broiler Chicken.” Indian Journal of Animal Science 87 (5): 640–643. https://doi.org/10.56093/ijans.v87i5.70270.
- Selle, P. H., S. P. Macelline, P. V. Chrystal, and S. Y. Liu. 2023. “The Contribution of Phytate-Degrading Enzymes to Chicken-Meat Production.” Animals 13 (4): 603. https://doi.org/10.3390/ani13040603.
- Shang, Y., and W. K. Kim. 2017. “Roles of Fructooligosaccharides and Phytase in Broiler Chickens: Review.” International Journal of Poultry Science 16 (1): 16–22. https://doi.org/10.3923/ijps.2017.16.22.
- Singh, A. K., B. Mishra, M. R. Bedford, and R. Jha. 2021. “Effects of Supplemental Xylanase and Xylooligosaccharides on Production Performance and Gut Health Variables of Broiler Chickens.” Journal of Animal Science and Biotechnology 12 (1): 98. https://doi.org/10.1186/s40104-021-00617-8.
- Sun, B., L. Hou, and Y. Yang. 2021. “The Development of the Gut Microbiota and Short-Chain Fatty Acids of Layer Chickens in Different Growth Periods.” Frontiers in Veterinary Science 8:666535. https://doi.org/10.3389/fvets.2021.666535.
- Suo, H.-Q., L. Lu, G.-H. Xu, L. Xiao, X.-G. Chen, R.-R. Xia, L.-Y. Zhang, and X.-G. Luo. 2015. “Effectiveness of Dietary Xylo-Oligosaccharides for Broilers Fed a Conventional Corn-Soybean Meal Diet.” Journal of Integrative Agriculture 14 (10): 2050–2057. https://doi.org/10.1016/S2095-3119(15)61101-7.
- Vázquez, M. J., J. L. Alonso, H. Domínguez, and J. C. Parajó. 2000. “Xylo-Oligosaccharides: Manufacture and Applications.” Trends Food Science and Technology 11 (11): 387–393. https://doi.org/10.1016/S0924-2244(01)00031-0.
- Yuan, L., W. Li, Q. Huo, C. du, Z. Wang, B. Yi, and M. Wang. 2018. “Effects of Xylo-Oligosaccharide and Flavomycin on the Immune Function of Broiler Chickens.” PeerJ 6:e4435. https://doi.org/10.7717/peerj.4435.
- Zhenping, S., L. Wenting, Y. Ruikui, L. Jia, L. Honghong, S. Wei, W. Zhongmie, L. Jingpan, S. Zhe, and Q. Yuling. 2013. “Effect of a Straw-Derived Xylooligosaccharide on Broiler Growth Performance, Endocrine Metabolism, and Immune Response.” Canadian Journal of Veterinary Research 77 (2): 105–109.
- Zhou, E., X. Pan, and X. Tian. 2009. “Application Study of Xylo-Oligosaccharide in Layer Production.” Modern Applied Science 3 (1): 103–107. https://doi.org/10.5539/mas.v3n1p103.