Abstract
Aims
Based on our previous research on the specific miRNAs identified from Gastrodia elata, we selected Gas-miR2-3p to investigate its effects on neuroinflammation via in vitro and in vivo experiments.
Results
RT-qPCR analysis indicated that G. elata specific Gas-miR2-3p was detected in all murine tissues post-oral administration, suggesting their potential as orally bioavailable miRNA. The analysis of RT-qPCR, Western blotting and ELISA assays consistently demonstrate that the expression of inflammatory factors as TNF-α, IL-6, IL-1β was decreased and the expression levels of p-p65 and p-IκBα were downregulated after the action of Gas-miR2-3p in both cell and animal experiments.
Conclusion
Gas-miR2-3p can attenuate neuroinflammation by regulating the inflammation factors and suppressing the activation of the NF-κB signaling pathway. Our findings indicate that G. elata miRNAs, as novel active components, perform a modulatory role in the NF-κB signaling pathway associated with neuroinflammation in a cross-species way.
Introduction
The inflammatory response can be triggered by various factors, wherein the upregulation of pro-inflammatory mediators represents a salient feature in its molecular mechanism. NF-κB serves as a pivotal regulator across diverse biological contexts such as immunity, ontogenesis and oncogenesis by precisely coordinating an extensive range of cellular events [Citation1-3]. In the context of the inflammatory response, the activation of the NF-κB signaling pathway induces a cascade of cytokines associated with immune function and pro-inflammatory factors (e.g. TNF-α, IL-6, IL-1β, COX2, iNOS, among others). Aberrant activation of the NF-κB signaling pathway in the nervous system can lead to dysregulation of the aforementioned inflammatory factors, resulting in neuroinflammation [Citation4]. The dysregulated inflammatory factors further aggravate neurological disorders by inducing neuronal damage [Citation5]. Subsequent investigations have revealed a compelling correlation between neuroinflammation and the NF-κB signaling pathway, prompting extensive exploration in this field.
MicroRNAs (miRNAs), a class of non-coding single-stranded RNA molecules with a length of 22-24 nucleotides encoded by endogenous genes of higher eukaryotes, have been demonstrated to play a pivotal role in diverse biological and cellular processes [Citation6,Citation7]. For instance, miR-101 exerts inhibitory effects on liver cancer metastasis by targeting multiple genes and suppressing hepatocyte-epithelial mesenchymal transition; whereas miR-34a suppresses tumor proliferation; miR-672-5p regulates cardiac hypertrophy by targeting the Jun gene; microRNA-122 as a potential biomarker for liver injury; miR-452 promotes liver cancer growth and spread by targeting Cyclin-Dependent Kinase Inhibitor 1B;miR-126 regulates angiogenesis and vascular integrity in animal models; regressions in cognitive function are correlated with reductions in plasma levels of miR-342-5p [Citation8-13]. In the regulation of inflammation, miR-223 and miR-105 can modulate toll receptor protein expression, whereas miR-9 and miR-21 are closely associated with the regulation of cellular pathways mediated by nuclear factor, miR-584 and miR-146a play crucial roles in inflammation and infection in acute respiratory distress syndrome [Citation14–18]. Moreover, the regulation of NF-κB signaling pathways by miRNAs is crucial in inflammatory response. For example, studies have shown that miR-146a/b can act as negative regulators of NF-κB protein activity in breast cancer, suggesting their therapeutic potential for inhibiting metastasis [Citation19]. In summary, miRNA plays a vital role in regulating inflammation via the NF-κB signaling pathway, offering novel therapeutic avenues for neuroinflammatory disorders.
In 2012, Zhang et al. made the groundbreaking discovery that miR-168a in rice can regulate target genes in humans through interspecies regulation, thus opening up a new research field on the cross-species regulation of plant miRNAs [Citation20]. In 2016, Wang et al. [Citation21] demonstrated that plant-derived miR-159 significantly suppressed the growth of mouse xenograft breast tumors. Other studies have shown that plant-derived miRNAs can withstand oral administration in animals and resist degradation by RNA hydrolases in the digestive tract. It is believed that these miRNAs can enter the systemic circulation and maintain stability for a certain period, indicating their potential for interspecies regulation in animals. Subsequently, accumulating evidence has suggested that miRNAs serve as connectors between different eukaryotic species, playing important biological roles in both animals and plants [Citation22–26].
Gastrodia elata Blume (G. elata), a perennial parasitic herb belonging to the family of Orchidaceae, is commonly referred to as ‘red arrow’ and is extensively utilized in traditional Chinese medicine through the use of its dried tubers [Citation27]. In ancient China, G. elata was found to treat various brain-related symptoms including convulsions, dizziness, headaches, and limb numbness as documented in the ‘Ming Yi Bie Lu’. Studies conducted by researchers in recent years have investigated its anti-inflammatory effects, which include alleviating pain and swelling while reducing bleeding and infection [Citation28–30]. At the molecular level, G. elata may achieve its anti-neuroinflammatory effect by inhibiting apoptosis-related pathways or activation of the NF-κB signaling pathway [Citation31,Citation32]. It has been demonstrated that gastrodin, a crucial constituent of G. elata, exerts inhibitory effects on the NF-κB signaling pathway, attenuates the release of inflammatory mediators, and mitigates chondrocyte matrix catabolism [Citation33]. Moreover, a plethora of other studies have demonstrated that the bioactive components and diverse extracts present in G. elata exhibit a certain degree of inhibitory activity toward neuroinflammation [Citation34–38].
Based on our previous research, 38 novel G. elata miRNAs were identified through high-throughput sequencing and bioinformatics analysis. Our findings indicate that G. elata specific miRNA Gas-miR01 (formally named Gas-miR2-3p) can regulate the A20 gene in an interspecific manner [Citation39]. Therefore, it can be inferred that G. elata specific miRNAs may modulate human signaling pathways via cross-species to fulfill their biological function as new active components of G. elata. To validate this conjecture, we conducted pertinent studies to investigate the important role of G. elata miRNA Gas-miR2-3p in modulating the NF-κB pathway during neuroinflammation.
Materials and methods
Materials
ICR female mice were procured from the animal experiment center of Zhejiang Chinese Medical University while C57BL/6 male mice were purchased from the animal experiment center of Hangzhou Normal University. The animal experiment has been approved by the Animal Ethics Committee of Zhejiang Sci-Tech University(Approval no. 202010086). Gas-miR2-3p mimics and negative control were synthesized by Gene Pharma in Shanghai, and BV2 microglia were derived from ATCC. The sequence for Gas-miR2-3p mimics is as follows: 5′-GUUCAGGAAUGCUGUGGGAAG-3′.
Animal experiment
ICR mice were divided into 6 experimental groups (Gastrodia decoction 0.5 h/3 h; Gastrodia powder 0.5 h/3 h; G. elata total RNAs 0.5 h/3 h) and a control group, with oral gavage treatment, following the previous treatment protocol, the dosage for the gavage treatment was determined based on our early experimental exploration [Citation39]. The cerebrum, cerebellum, hypothalamus, spleen and kidney were collected at 0.5 h/3 h after oral gavage. Animals were caged (3–5 mice per cage) at a temperature of 24 °C.
C57BL/6 mice were divided into three experimental groups: control, negative control, and Gas-miR2-3p group. The Gas-miR2-3p group and the negative control group received intrathecal injections of Gas-miR2-3p (2 μg/each) and negative control (2 μg/each), respectively, for six consecutive days. After that, the Gas-miR2-3p group and the negative control group were intraperitoneally injected with lipopolysaccharide (LPS, 1 mg/ml, 1 mg/kg) for three consecutive days. Six hours after the last injection, mice were anesthetized and their tissues were harvested.
Transfection and cell experiment
Gas-miR2-3p mimics and negative control mimics were transfected into the BV2 microglia at a transfection ratio of 70 pmol/1 × 105 cells using Lipofectamine™ 3000 transfection reagent according to the manufacturer’s instructions. Subsequently, the cells were harvested after induction with LPS (1 μg/mL) for further experimental procedures.
RNA extraction and RT-qPCR
Total RNA samples extracted from mouse tissues and LPS-stimulated BV2 cells were subjected to reverse transcription using the PrimeScript™ RT reagent Kit (Takara). The RT-qPCR was performed with Fast Start Universal SYBR Green Master (Rox, Roche) according to the following program: 95 °C for 10 min, followed by 40 cycles of 95 °C for 15 s, 60 °C for 15 s, and 72 °C for 30 s (). Stem-loop primer was also used to examine miRNA by using 5.8S rRNA as normal control (). RT-qPCR primers and stem-loop primers of Gas-miR2-3p are as follows:
Table 1. The RT-qPCR primers of Mus 5.8S, Mus TNF, Mus IL-6, Mus IL-1β, Mus COX-2, Mus iNOS and Gas-miR2-3p.
Table 2. Stem-loop primer of Gas-miR2-3p.
ELISA
The levels of pro-inflammatory cytokines TNF-α, IL-6, and IL-1β were quantified using a Mouse ELISA Kit (Lianke, China) following specific instructions for samples and standards preparation after collecting animal tissues and BV2 cell medium induced by LPS. The microplate was soaked and dried, and then the corresponding samples and standards were added. The detection antibody was then incubated with the microplate for a specified duration. Subsequently, an enzyme working solution was added, followed by washing and drying of the microplate. The substrate was then added and incubated in darkness before finally adding a termination solution to measure the degree of detection.
Western blotting
The brain tissues obtained from animal experiments and LPS-induced BV2 cells were lysed with Cell lysis buffer (Beyotime, China) to extract total protein. The protein concentration was determined using the BCA Protein Assay Kit (Beyotime, China). Subsequently, the proteins were separated on a 12% SDS-PAGE gel and transferred onto a polyvinylidene difluoride (PVDF) membrane (Millipore, USA) via electroblotting. After blocking with 5% skim milk for 2 h, the protein was detected with β-tubulin (Proteintech, USA) and NF-κB Pathway Simple Kit (Cell Signaling Technology, USA) overnight at 4 °C. The following day, the membrane was rinsed with TBST at room temperature and then incubated with HRP-labeled mouse anti-rabbit secondary antibody and HRP-labeled horse anti-mouse secondary antibody for one hour. The expression level of related proteins was detected using the ECL Detection Kit (Advansta).
Data analysis
The data presented are the means of three independent experiments expressed as mean ± sd. Statistical analysis was performed using t-test.
Results
Detection of G. elata miRNAs in mouse tissues after oral administration
Gas-miR2-3p was detected by RT-qPCR analysis, although its level in Gastrodia decoction and Gastrodia powder samples was slightly lower compared to total RNAs (). RNA was extracted from murine tissues and reverse transcribed, followed by RT-qPCR quantification to assess the level of Gas-miR2-3p. Significant elevation of Gas-miR2-3p levels in the spleen, kidney, cerebrum, and hypothalamus were observed following oral gavage compared to the control group. At 3 h post-gavage, there was a significant elevation in the levels of Gas-miR2-3p observed in the spleen, kidney, cerebellum, and hypothalamus, while a marked increase was detected only in the cerebrum at 0.5 h post-gavage (). These results indicate that oral administration is an effective method for delivering Gas-miR2-3p into mice.
Figure 1. The relative quantity of Gas-miR2-3p in G. elata total RNAs, Gastrodia decoction, Gastrodia powder and different mouse tissues. Each mouse was fed with G. elata total RNAs, Gastrodia decoction, Gastrodia powder. The relative quantity of Gas-miR2-3p was detected by RT-qPCR (A) Gastrodia decoction, Gastrodia powder and G. elata total RNA; (B) spleen; (C) kidney; (D) cerebrum; (E) cerebellum; (F) hypothalamus; *p < 0.05; **p < 0.01; ***p < 0.001, compared to control group, n = 6.
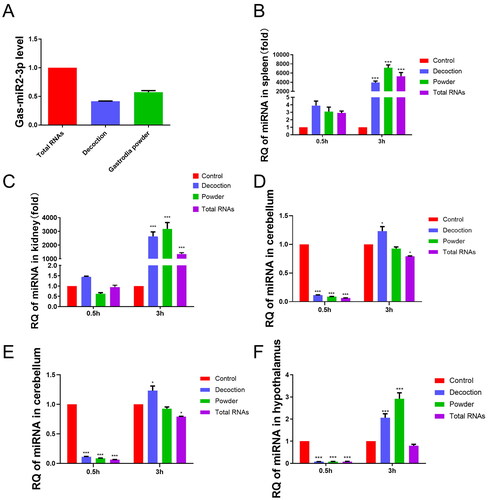
Gas-miR2-3p mitigates LPS-induced inflammation in BV2 microglia
To investigate the regulatory impact of Gas-miR2-3p on the NF-κB signaling pathway and its potential anti-inflammatory effects, we performed in vitro cellular experiments. We quantified the mRNA expression levels of inflammatory cytokines in BV2 microglia using RT-qPCR and observed a significant upregulation of these cytokines in the neuroinflammatory cell model upon induction via LPS. Importantly, treatment with Gas-miR2-3p resulted in a significant attenuation of the mRNA levels of TNF-α, IL-6, IL-1β, COX2, and iNOS compared to the NC group ().
Figure 2. Effect of Gas-miR2-3p on mRNA levels of inflammatory factors in BV2 cells induced by LPS. The mRNA levels of TNF-α (A), IL-6 (B), IL-1β (C), COX-2 (D), iNOS (E). *p < 0.05; **p < 0.01; ***p < 0.001, vs NC, NC: negative control.
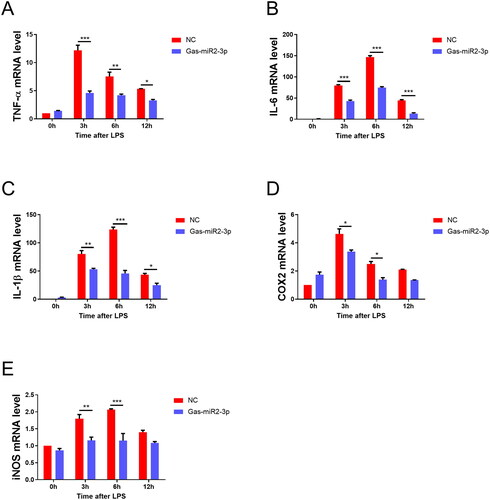
Additionally, we utilized Western blotting to assess the levels of relevant proteins in the NF-κB signaling pathway in BV2 cells following LPS induction. The results showed a significant increase in the levels of p-p65 and p-IκBα upon LPS stimulation. However, transfection ofGas-miR2-3p into BV2 cells resulted in a significant reduction of p-p65 protein and a notable decrease in p-IκBα protein at 120 min post LPS induction (). Likewise, transfecting with Gas-miR2-3p markedly decreased the levels of TNF-α, IL-1β and IL-6 as measured by ELISA in BV2 cells stimulated with LPS (), suggesting that Gas-miR2-3p may modulate the NF-κB signaling cascade to suppress neuroinflammatory responses.
Figure 3. Effect of Gas-miR2-3p on inflammatory factors and NF-κB signaling pathway in LPS-induced BV2 cells. The levels of p65, p-p65 (A), and p-IκBα, IκBα (C) protein after LPS induction. The relative intensity of p65, p-p65 (B), and p-IκBα, IκBα (D) was normalized. The levels of TNF-α (E), IL-1β (F), and IL-6 (G) after 6 h induced by LPS in BV2 cell. *p < 0.05; **p < 0.01; ***p < 0.001, vs NC, NC: negative control.
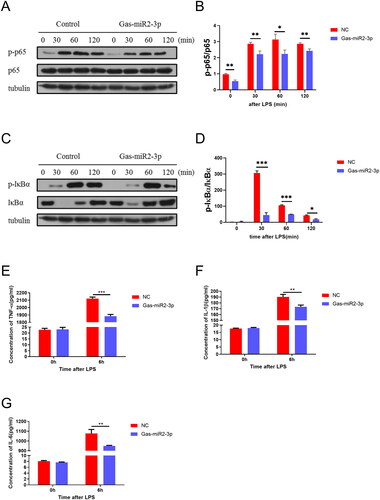
Gas-miR2-3p alleviates LPS-induced neuroinflammation in mice
To further validate our cellular findings, we conducted animal experiments wherein an intraperitoneal injection of LPS was administered on day 4, resulting in weight loss among the mice. By the sixth day, a significant difference between the Gas-miR2-3p group and the NC group was observed, with the former exhibiting less weight loss compared to the latter (). Moreover, the mRNA levels of TNF-α, IL-6, IL-1β, COX2, and iNOS were significantly upregulated following LPS induction, suggesting the mouse neuroinflammation model was established successfully. In contrast, treatment with Gas-miR2-3p resulted in a downregulation in the mRNA expression of each inflammatory factor (). The aforementioned results suggest that Gas-miR2-3p may exert an inhibitory effect on the inflammatory response in mice.
Figure 4. Determination of body weight of mice and effect of Gas-miR2-3p on mRNA levels of inflammatory factors in mice induced by LPS. (A) The weight of mice was detected daily. The mRNA levels of TNF-α (B), IL-6 (C), IL-1β (D), COX-2 (E), iNOS (F) in mouse brain. *p < 0.05; **p < 0.01; ***p < 0.001, vs NC, NC: negative control.
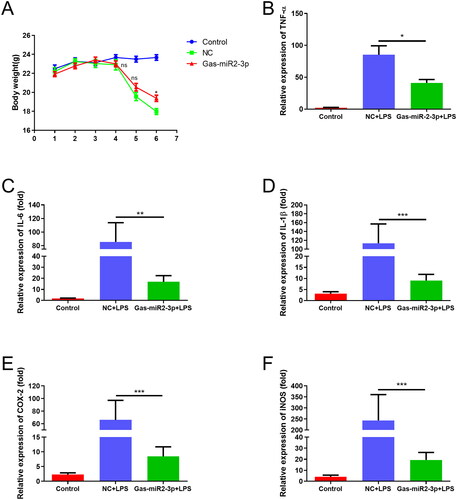
We conducted Western blotting to evaluate the levels of specific proteins in the NF-κB signaling pathway in mouse brain tissue. The results showed that LPS stimulation induced an upregulation of p-p65 and p-IκBα levels, wherein treatment with Gas-miR2-3p resulted in a downregulation of p-p65 and p-IκBα levels. Notably, the expression level of the p65 protein remained constant throughout the experiment (). Furthermore, we quantified the levels of inflammatory cytokines in the mouse brain using ELISA. The results indicated that the levels of TNF-α, IL-1β, and IL-6 were elevated following intraperitoneal administration of LPS. Conversely, intrathecal injection with Gas-miR2-3p treatment significantly reduced the levels of inflammatory factors. (). Gas-miR2-3p exhibits a certain inhibitory effect on neuroinflammation in mice, indicating its potential therapeutic value for diseases of the nervous system.
Figure 5. Effect of Gas-miR2-3p on inflammatory factors and NF-κB signaling pathway in LPS-induced mice. The levels of p65, p-p65 (A), and p-IκBα, IκBα (C) protein after LPS induction. The relative intensity of p65, p-p65 (B), and p-IκBα, IκBα (D) was normalized. The levels of TNF-α (E), IL-1β (F), and IL-6 (G) after 6 h induced by LPS in mice. *p < 0.05; **p < 0.01, vs NC, NC: negative control.
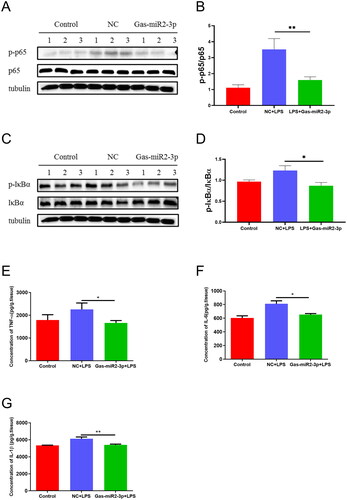
Discussion
Research has shown that plant-derived miRNAs can interact with animal mRNA, indicating their significant biological role [Citation40–42]. Our study detected the G. elata specific Gas-miR2-3p in mice, demonstrating its oral bioavailability and laying a foundation for interspecies regulation in animals.
G. elata has been utilized for over a millennium and pharmacological studies have demonstrated its multifaceted therapeutic effects, particularly in the inhibition of neuroinflammatory activation [Citation43–47]. The ethanol extract of G. elata can relieve TNF-α induced vascular inflammation by suppressing oxidative stress and NF-κB pathway activation [Citation48]. Moreover, the crucial role of miRNA in the context of inflammatory stress cannot be underestimated, particularly in the neuroinflammation-associated NF-κB signaling pathway [Citation49–51].
Neuroinflammation is a multifaceted response triggered by exogenous agents and/or tissue injury, which confers significant short-term benefits by eliminating hazardous stimuli and initiating reparative processes [Citation52-54]. Chronic low-grade neuroinflammation can disrupt the body’s homeostasis, leading to a cascade of pathological conditions such as Alzheimer’s disease (AD) and major depressive disorder (MDD). Blocking the NF-κB signaling pathway has been recognized as an effective approach to treating inflammation [Citation39, Citation55]. Therefore, we screened for G. elata specific miRNA that could target this signaling pathway.
To further investigate the underlying mechanisms and correlations between G. elata specific Gas-miR2-3p and NF-κB signaling pathway, we conducted in vitro cellular and in vivo animal experiments. RT-qPCR, Western blotting, and ELISA assays collectively demonstrated that Gas-miR2-3p exerts inhibitory effects on the NF-κB signaling pathway, resulting in downregulation of mRNA levels of inflammatory factors and attenuation of TNF-α, IL-6 and IL-1β expression. Results suggest that the NF-κB signaling pathway can be regulated by Gas-miR2-3p, highlighting its pivotal role in the suppression of neuroinflammation and providing novel insights into the interspecies regulatory mechanism of G. elata specific miRNAs. Modulating the NF-κB pathway through Gas-miR2-3p resulted in a significant reduction of inflammatory factors at both cellular and organismal levels. As a natural component of traditional Chinese medicine, G. elata specific miRNAs can enter the systemic circulation and function via cross-species regulation. Modulation of Gas-miR2-3p on neuroinflammation is helpful to prevent other neurological diseases caused by an imbalance in inflammatory factors within the nervous system.
It is widely acknowledged that miRNAs exert their inhibitory effects on specific proteins at the translational level by selectively targeting the mRNA of these proteins. Given the indisputable potent efficacy of Gastrodia elata in central nervous system (CNS) disorders, we focused on G. elata specific miRNAs. Our previous experiments have demonstrated that G. elata-specific miRNAs effectively target crucial proteins within the human NF-κB signaling pathway across species. The results in this study indicate that G. elata specific miRNA could downregulates the hyperactivation of the NF-κB signaling pathway resulting from aberrant stimulation, thereby preventing the excessive release of inflammatory factors and protecting the central nervous system (CNS) against inflammatory attacks. It is of paramount importance for the prevention of central nervous system (CNS) diseases to suppress neuroinflammation, thereby mitigating the occurrence of refractory conditions that pose significant therapeutic challenges. The cross-species regulatory effect of G. elata specific miRNA was validated in this experiment, further substantiating that not only phytochemicals like gastrodin can exert regulatory functions in the central nervous system (CNS), but also G. elata miRNAs can confer beneficial regulatory effects on the human CNS across species. The results provide a new understanding on the transboundary regulatory mechanism of Gastrodia elata specific miRNAs, and also lay a theoretical basis for the subsequent research on Gastrodia elata miRNAs as a novel pharmacodynamic substance.
Conclusion
In this study, we initially conducted the animal experiment and utilized RT-qPCR to detect miRNA expression profiles across various tissues. Our findings indicate that G. elata specific miRNA can be absorbed by entering the internal environment of mice through the digestive tract, as evidenced by the detection of Gas-miR2-3p in certain mouse tissues. Our in vitro experiment demonstrated the inhibitory effect of Gas-miR2-3p on neuroinflammation in BV2 cells. The combined use of RT-qPCR, Western blotting and ELISA assays revealed that Gas-miR2-3p suppresses the NF-κB signaling pathway, leading to a decrease in inflammatory factors and attenuation of TNF-α、IL-6、IL-1β expression. Furthermore, the in vivo findings were consistent with those obtained in vitro, suggesting that Gas-miR2-3p may attenuate neuroinflammation by inhibiting the NF-κB signaling pathway through interspecies regulation.
Authors’ contributions
J.F. and Z.T. wrote the manuscript, G.W., Z.Y., X.W., S.L., D.W., Z.N., and Q.S. contributed to the writing of the manuscript. Q.S. is responsible for the article.
Disclosure statement
The authors declare that the research was conducted in the absence of any commercial or financial relationships that could be construed as a potential conflict of interest.
Additional information
Funding
References
- Zhang Q, Lenardo MJ, Baltimore D. 30 years of NF-kappaB: a blossoming of relevance to human pathobiology. Cell. 2017;168(1–2):37–57. doi: 10.1016/j.cell.2016.12.012.
- Luo Y,Yang Z,Su L, et al. Non-cscs nourish cscs through interleukin-17E-mediated activation of NF-κb and JAK/STAT3 signaling in human hepatocellular carcinoma. Cancer Lett. 2016;375(2):390–399. doi: 10.1016/j.canlet.2016.03.012.
- Yang Y,Sheng Q,Nie Z, et al. Daphnetin inhibits spinal glial activation via Nrf2/HO-1/NF-κb signaling pathway and attenuates CFA-induced inflammatory pain. Int Immunopharmacol. 2021;98:107882.doi: 10.1016/j.intimp.2021.107882.
- Wang D,Lu Z,Zhang H, et al. Daphnetin alleviates experimental autoimmune encephalomyelitis via regulating dendritic cell activity. CNS Neurosci Ther. 2016;22(7):558–567. doi: 10.1111/cns.12537.
- Kempuraj D, Thangavel R, Natteru PA, et al. Neuroinflammation induces neurodegeneration. Other. 2016;1(1):1003.
- Felekkis K, Touvana E, Stefanou C, et al. microRNAs: a newly described class of encoded molecules that play a role in health and disease. Hippokratia. 2010;14(4):236–240.
- Li Y,Jiang Z,Xu L, et al. Stability analysis of liver cancer-related micrornas. Acta Biochim Biophys Sin (Shanghai). 2011;43(1):69–78. doi: 10.1093/abbs/gmq114.
- Zheng F, Liao YJ, Cai MY, et al. Systemic delivery of microRNA-101 potently inhibits hepatocellular carcinoma in vivo by repressing multiple targets. PLoS Genet. 2015;11(2):e1004873. doi: 10.1371/journal.pgen.1004873.
- Shi Y, Liu C, Liu X, et al. The microRNA miR-34a inhibits non-small cell lung cancer (NSCLC) growth and the CD44hi stem-like NSCLC cells. PLoS One. 2014;9(3):e90022. doi: 10.1371/journal.pone.0090022.
- Lu Y,Wu F. A new mirna regulator, mir-672, reduces cardiac hypertrophy by inhibiting JUN expression. Gene. 2018;648:21–30. doi: 10.1016/j.gene.2018.01.047.
- Ding X, Ding J, Ning J, et al. Circulating microrna-122 AS A potential biomarker for liver injury. Mol Med Report. 2012;5:1428–1432. doi: 10.3892/mmr.2012.838.
- Xu J,Wu K-J,Jia Q-J, et al. Roles of mirna and lncrna in triple-negative breast cancer. J Zhejiang Univ Sci B. 2020;21(9):673–689. doi: 10.1631/jzus.B1900709.
- Zheng Q,Sheng Q,Jiang C, et al. MicroRNA-452 promotes tumorigenesis in hepatocellular carcinoma by targeting cyclin-dependent kinase inhibitor 1B. Mol Cell Biochem. 2014;389(1-2):187–195. doi: 10.1007/s11010-013-1940-z.
- Wang J, Bai X, Song Q, et al. miR-223 inhibits lipid deposition and inflammation by suppressing toll-like receptor 4 signaling in macrophages. Int J Mol Sci. 2015;16(10):24965–24982. doi: 10.3390/ijms161024965.
- Benakanakere MR, Li Q, Eskan MA, et al. Modulation of TLR2 protein expression by miR-105 in human oral keratinocytes. J Biol Chem. 2009;284(34):23107–23115. doi: 10.1074/jbc.M109.013862.
- Huang G, Liu X, Zhao X, et al. MiR-9 promotes multiple myeloma progression by regulating TRIM56/NF-κB pathway. Cell Biol Int. 2019;43(11):1223–1233. doi: 10.1002/cbin.11104.
- Pan YQ, Li J, Li XW, et al. Effect of miR-21/TLR4/NF-κB pathway on myocardial apoptosis in rats with myocardial ischemia-reperfusion. Eur Rev Med Pharmacol Sci. 2018;22(22):7928–7937.
- Zhang S,Hong Y,Liu H, et al. miR‑584 and miR‑146 are candidate biomarkers for acute respiratory distress syndrome. Exp Ther Med. 2021;21(5). doi: 10.3892/etm.2021.9873.
- Bhaumik D, Scott GK, Schokrpur S, et al. Expression of microRNA-146 suppresses NF-kappaB activity with reduction of metastatic potential in breast cancer cells. Oncogene. 2008;27(42):5643–5647. doi: 10.1038/onc.2008.171.
- Zhang L, Hou D, Chen X, et al. Exogenous plant MIR168a specifically targets mammalian LDLRAP1: evidence of cross-kingdom regulation by microRNA. Cell Res. 2012;22(1):107–126. doi: 10.1038/cr.2011.158.
- Chin AR, Fong MY, Somlo G, et al. Cross-kingdom inhibition of breast cancer growth by plant miR159. Cell Res. 2016;26(2):217–228. doi: 10.1038/cr.2016.13.
- Liang G, Zhu Y, Sun B, et al. Assessing the survival of exogenous plant microRNA in mice. Food Sci Nutr. 2014;2(4):380–388. doi: 10.1002/fsn3.113.
- Pastrello C, Tsay M, Mcquaid R, et al. Retraction: circulating plant miRNAs can regulate human gene expression in vitro. Sci Rep. 2017;7(1):46826. doi: 10.1038/srep46826.
- Jiang M, Sang X, Hong Z. Beyond nutrients: food-derived microRNAs provide cross-kingdom regulation. Bioessays. 2012;34(4):280–284. doi: 10.1002/bies.201100181.
- Zeng J, Gupta VK, Jiang Y, et al. Cross-kingdom small RNAs among animals, plants and microbes. Cells. 2019;8(4):371. doi: 10.3390/cells8040371.
- Trivedi, R, Asif, MH, Trivedi, PK, et al. Dietary plant miRNAs as an augmented therapy: cross-kingdom gene regulation. RNA Biol. 2018;15(12):1433–1439. doi: 10.1080/15476286.2018.1551693.
- Commission CP. Pharmacopoeia of the people’s republic of China. Beijing: China Medical Science Press; 2015.
- Shao QH, Zhang XL, Chen Y, et al. Anti-neuroinflammatory effects of 20C from gastrodia elata via regulating autophagy in LPS-activated BV-2 cells through MAPKs and TLR4/akt/mTOR signaling pathways. Mol Immunol. 2018;99:115–123. doi: 10.1016/j.molimm.2018.04.014.
- Kho MC, Lee YJ, Cha JD, et al. Gastrodia elata ameliorates high-fructose diet-induced lipid metabolism and endothelial dysfunction. Evid Based Complement Alternat Med. 2014;2014:101624–101610. doi: 10.1155/2014/101624.
- Li Y, Wang LM, Xu JZ, et al. Gastrodia elata attenuates inflammatory response by inhibiting the NF-kappaB pathway in rheumatoid arthritis fibroblast-like synoviocytes. Biomed Pharmacother. 2017;85:177–181. doi: 10.1016/j.biopha.2016.11.136.
- Kim BW, Koppula S, Kim JW, et al. Modulation of LPS-stimulated neuroinflammation in BV-2 microglia by gastrodia elata: 4-hydroxybenzyl alcohol is the bioactive candidate. J Ethnopharmacol. 2012;139(2):549–557. doi: 10.1016/j.jep.2011.11.048.
- Huang H, Zhang Y, Yao C, et al. The effects of fresh gastrodia elata blume on the cognitive deficits induced by chronic restraint stress. Front Pharmacol. 2022;13:890330. doi: 10.3389/fphar.2022.890330.
- Chen J, Gu Y-T, Xie J-J, et al. Gastrodin reduces IL-1β-induced apoptosis, inflammation, and matrix catabolism in osteoarthritis chondrocytes and attenuates rat cartilage degeneration in vivo. Biomed. Pharmacotherapy. 2018;97:642–651. doi: 10.1016/j.biopha.2017.10.067.
- Xiang B, Xiao C, Shen T, et al. Anti-inflammatory effects of anisalcohol on lipopolysaccharide-stimulated BV2 microglia via selective modulation of microglia polarization and down-regulation of NF-kappaB p65 and JNK activation. Molecular Immunol. 2018;95:39–46. doi: 10.1016/j.molimm.2018.01.011.
- Ng CF, Ko CH, Koon CM, et al. The aqueous extract of rhizome of gastrodia elata blume attenuates locomotor defect and inflammation after traumatic brain injury in rats. J Ethnopharmacol. 2016;185:87–95. doi: 10.1016/j.jep.2016.03.018.
- Chen C, Fu YH, Li MH, et al. Nuclear magnetic resonance-based metabolomics approach to evaluate preventive and therapeutic effects of gastrodia elata blume on chronic atrophic gastritis. J Pharm Biomed Anal. 2019;164:231–240. doi: 10.1016/j.jpba.2018.10.035.
- Zhang T, Huang S, Qiu J, et al. Beneficial effect of gastrodia elata blume and poria cocos wolf administration on acute UVB irradiation by alleviating inflammation through promoting the gut-skin axis. Int J Mol Sci. 2022;23(18):10833.
- Zhao J, Bi W, Xiao S, et al. Neuroinflammation induced by lipopolysaccharide causes cognitive impairment in mice. Sci Rep. 2019;9(1):5790. doi: 10.1038/s41598-019-42286-8.
- Xia C, Zhou H, Xu X, et al. Identification and investigation of miRNAs from gastrodia elata blume and their potential function. Front Pharmacol. 2020;11:542405. doi: 10.3389/fphar.2020.542405.
- Yang J, Farmer LM, Agyekum AA, et al. Detection of dietary plant-based small RNAs in animals. Cell Res. 2015;25(4):517–520. doi: 10.1038/cr.2015.26.
- Yang J, Farmer LM, Agyekum AA, et al. Detection of an abundant plant-based small RNA in healthy consumers. PLoS One. 2015;10(9):e0137516. doi: 10.1371/journal.pone.0137516.
- Liu Y-C, Chen WL, Kung W-H, et al. Plant miRNAs found in human circulating system provide evidences of cross kingdom RNAi. BMC Genom. 2017;18(S2):112. doi: 10.1186/s12864-017-3502-3.
- Zhou Z, Lin Y, Zheng H, et al. Anticonvulsive and neuroprotective effects of synergetic combination of phenytoin and gastrodin on the convulsion induced by penicillin in mice. Fundam Clin Pharma. 2015;29(4):371–381. doi: 10.1111/fcp.12127.
- Yuan F, Xu X, Wu Y, et al. Gastrodin ameliorates acute rejection via IRE1alpha/TRAF2/NF-kappaB in rats receiving liver allografts. Biomed Res Int. 2019;2019:1–11. doi: 10.1155/2019/9276831.
- Xi Z, Qiao Y, Wang J, et al. Gastrodin relieves inflammation injury induced by lipopolysaccharides in MRC-5 cells by up-regulation of miR-103. J Cell Mol Med. 2020;24(2):1451–1459.
- Qiu H, Tang W, Tong X, et al. Structure elucidation and sulfated derivatives preparation of two alpha-D-glucans from gastrodia elata Bl. and their anti-dengue virus bioactivities. Carbohydr Res. 2007;342(15):2230–2236.
- Song Z, Luo G, Han C, et al. Potential targets and action mechanism of gastrodin in the treatment of attention-deficit/hyperactivity disorder: bioinformatics and network pharmacology analysis. Evid Based Complement Alternat Med. 2022;2022:1–12.
- Ahn EK, Jeon HJ, Lim EJ, et al. Anti-inflammatory and anti-angiogenic activities of gastrodia elata blume. J Ethnopharmacol. 2007;110(3):476–482. doi: 10.1016/j.jep.2006.10.006.
- Mo JS, Han SH, Yun KJ, et al. MicroRNA 429 regulates the expression of CHMP5 in the inflammatory colitis and colorectal cancer cells. Inflamm. Res. 2018;67(11–12):985–996. doi: 10.1007/s00011-018-1194-z.
- Wendlandt EB, Graff JW, Gioannini TL, et al. The role of microRNAs miR-200b and miR-200c in TLR4 signaling and NF-κB activation. Innate Immun. 2012;18(6):846–855. doi: 10.1177/1753425912443903.
- Dai L, Gu L, DI W. MiR-199a attenuates endometrial stromal cell invasiveness through suppression of the IKKβ/NF-κB pathway and reduced interleukin-8 expression. Mol Hum Reprod. 2012;18(3):136–145. doi: 10.1093/molehr/gar066.
- Piscopo P, Albani D, Castellano AE, et al. Frontotemporal lobar degeneration and MicroRNAs. Front Aging Neurosci. 2016;8:17. doi: 10.3389/fnagi.2016.00017.
- Jo SL, Yang H, Jeong KJ, et al. Neuroprotective effects of ecklonia cava in a chronic neuroinflammatory disease model. Nutrients. 2023;15(8):2007. doi: 10.3390/nu15082007.
- Song D,Tao W,Liu F, et al. Lipopolysaccharide promotes NLRP3 inflammasome activation by inhibiting TFEB-mediated autophagy in NRK-52E cells. Molecular Immunology. 2023;163:127–135. doi: 10.1016/j.molimm.2023.09.008.
- Wang P, Li C, Liao G, et al. Vanillin attenuates proinflammatory factors in a tMCAO mouse model via inhibition of TLR4/NF-κB signaling pathway. Neuroscience. 2022;491:65–74. doi: 10.1016/j.neuroscience.2022.03.003.