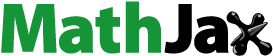
ABSTRACT
We examined the effect of laser processing surface treatment on the mechanical strength of adhesion. In addition to laser processing, double cantilever beam (DCB) and lap joint (LJ) specimens were prepared using an epoxy adhesive on aluminum plates that underwent acid etching, sandblasting, and acetone wiping. The open face method was employed to accelerate moisture degradation, and the tensile-shear strength and energy release rate of the specimens were measured. Laser processing resulted in remarkably high values of the measured energy release rate, even when the adhesive deteriorated. To examine the reason for the high strength, we performed a detailed fracture surface analysis and electron microscopic observation of the adhesive interface. When the interface of the fractured surface was observed, the laser treated surface was densely formed with fine filamentous protrusions that held the adhesive well. These filaments exhibited high oxidation resistance and achieved strong adhesion even when the adhesive and adherend deteriorated because of moisture. The laser treated adherend surface exhibited two strong anchoring effects owing to a significant increase in the surface area and the microfilamentous structure on the surface. However, the type of surface treatment did not have a significant effect on the LJ adhesion strength.
1. Introduction
There is a strong need to reduce the weight of aircraft and vehicles to meet the demands of energy conservation and environmental protection. One of the most effective methods is to join dissimilar materials, which requires an innovative adhesive bonding technology. However, to obtain an adhesive joint for practical use, reliability and durability are important.[Citation1, Citation2] The strength of a joint is determined by three elements, namely the adhesive, adhesive interface, and adherend. The most significant factor that contributes to failure is moisture deterioration of the adhesive.[Citation3–7] Previous studies investigated the effects of fatigue, creep, and environmental temperature loads.[Citation8–10] Deterioration at the interface causes interfacial fractures, resulting in extremely decreasing strength and durability. To use adhesive bonding for structural purposes, it is necessary to design interfaces that are highly resistant to fracture.
Therefore, this study focused on surface treatment, which has a significant effect on the adhesive interface strength. Many studies have proposed different surface treatment methods for adhesion. These include degreasing to eliminate oil contamination that weakens bonds, chemical treatment[Citation11] to create surface nanostructures that promote the formation of an anchor effect, and blasting to roughen, grind, and clean surfaces. Flame treatment,[Citation12] atmospheric pressure plasma treatment,[Citation12–14] and laser treatment,[Citation15–17] as well as removing surface contaminants, enable control of the formation of reactive functional groups on the resin surface. In recent years, several researchers have focused on improving the durability of metal adherends using laser processing to achieve high adherend corrosion resistance and cohesive fracture in severe environments.[Citation18–21] However, few studies have examined the relationship between mechanical strength and various types of surface treatments that significantly accelerate the deterioration of adhesives with open-faced specimens.[Citation22] Furthermore, studies of continuous observation of specimens with deteriorated fracture surfaces over time are also necessary. In our previous research, we systematically examined the relationship between the laser processing conditions, surface texture, and adhesive strength, and clarified the best processing conditions.[Citation23] In this study, test specimens of lap joints (LJ) and double cantilever beams (DCB) were processed using four types of surface treatments: laser, acid, sand blast, and acetone wipe. Open-faced specimens[Citation24] (hereafter referred to as Open LJ and Open DCB were fabricated using test specimens with accelerated deterioration by hot water, and their mechanical strength was evaluated. To examine the effect of the laser treated adherends on the adhesive strength, we analyzed the adhesive interface using scanning electron microscopy (SEM).
The rest of this paper is organized as follows. Section 2 presents the specimen and materials used in the experiment, as well as the experimental procedure. We prepared LJ and compared the effects of surface treatment on adhesive strength and its dispersion using the Weibull probability function, as described in Section 3-1. Next, we selected Open LJ and evaluated the shear strength when moisture deterioration of the adhesive was accelerated. The effect of surface treatment on the Mode 1 bonding strength of deteriorated adhesive was examined using Open DCB while observing the fracture surface, as presented in Section 3-2. Furthermore, in Section 3-3, we discussed why laser treatment improves the adhesive strength through detailed observation of the adherend surface and adhesive interface using SEM/EDS. The salt spray cycle test results are presented in Section 3-4. Finally, the main conclusions of the study are summarized in Section 4.
2. Specimen and experimental procedure
2.1. Materials of adhesive and adherend
The selected adhesive was an epoxy thermosetting adhesive (Not commercial; Cemedine Co., Ltd., Japan), which is the chemical composition is listed in . Carboxyl-terminated butadiene acrylonitrile rubber (CTBN) was added to improve the elongation and toughness. The mechanical properties of the cured adhesive are also listed in the table.[Citation5, Citation7] A6061-T6 supplied by UACJ Co. Ltd. Japan was used as the adherend, and the tensile strength, proof stress, and elongation were 295 MPa, 245 MPa, and 10 %, respectively. The adhesive was mixed in two stages to achieve evenness. First, dicyane diamide and 3-(3,4-dichlorophenyl)-1,1’-dimethylurea were stirred and mixed into 1/3 of the epoxy resin. The remaining epoxy resin was then divided into two batches and stirred and mixed until uneven to create a pre-paste. Other ingredients were stirred and mixed continuously, and the pre-paste was stirred and mixed with the mixture while controlling the temperature to below 40 °C.
Table 1. Chemical composition of adhesive (Mass%) and mechanical property of adhesive bulk.
2.2. Four types of adherend surface treatment
To examine the effects of surface treatments on the adhesive strength, we performed four types of surface treatments, as described above. The adherends surface-treated with laser, acid, blast, and wipe were prepared. A laser scanning system (MPS-502, Keyence Co., Japan) equipped with a nanosecond pulse (λ = 1098 nm) was used for the laser surface treatment. In a previous study, we showed that the overlap rate of the laser spot on the surface has a significant effect on the adhesion strength.[Citation23] lists the surface treatment conditions for the laser, sandblasting, and acid treatment used in this study.
Table 2. Specifications of surface treatment (laser, blast and acid).
The laser scan was processed under trace conditions that created an overlap of 44% of the laser spot diameter in both the X and Y directions. In addition, all the specimens were processed by enlarging the adhesive area by 10 –15 % to avoid edge effects.
2.3. Specime
show the DCB specimen, which is according to the ISO standard,[Citation25] used for measuring the energy release rate (G1C) and the LJ specimen used for measuring the shear strength, respectively. The thickness of the adhesive layer was controlled at 0.3 mm, however, the thickness of the adhesive layer of the Open Face specimen was set to 0.15 mm because of one side water absorption. Because adhesive layer thickness significantly affects G1C and the deterioration rate, accurate thickness control is required. shows the procedure for controlling the adhesive layer thickness of Open Face specimens. To guarantee the adhesive layer thickness, poly-tetra-fluoro-ethylene (PTFE) sheets with t = 0.15 mm were placed on the adherend together with the adhesive and cured while applying a load. The curing temperature was maintained at 180°C for 1 h in electro furnace.
2.4. Experimental equipment and procedure
A universal test machine (AGS-X10kN, AUTOGRAPH, Shimadzu Co., Japan) and DCB dedicated tester (2.5kN Zwicki, ZwickiLine, ZwickRoell Co., Germany) were used for the tensile shear tests of LJ and G1C measurements of DCB, respectively. A salt spray tester (CYP-90, Suga Test Instruments Co., Japan) was used for the salt spray cycle test.
The degradation acceleration process of the Open LJ/DCB used in this study is shown in . An adhesive with a 0.15 mm thickness was deposited on the adherend. The adhesive was cured on the adherend and then immersed in water at 63 °C. We chose 63 °C, which can damage both the adhesive and the adherend.[Citation7] After removal from water, the specimens were bonded using a secondary cyanoacrylate adhesive (EXTRA 4000, TOAGOSEI CO., LTD. Japan). To measure the adhesive strength in the water-absorbed state, we used a secondary adhesive to ensure that it hardens in a short time at room temperature.[Citation26] The samples were then cured for 2 h.
2.5. SEM observation
To visualize the surface and cross section of surface treatment obtained using laser, acid, and blast, field-emission scanning electron microscopes (FE-SEM: ZEISS Ultra55 and Hitachi SU5000) were used at a low acceleration voltage of 1–3 kV. SEM observations are usually performed at a higher acceleration voltage of 5–15 kV because a higher spatial resolution should be obtained owing to the sharper electron beam. In contrast, incident electrons with higher energy would interact deeper and wider with the specimen, decreasing the actual spatial resolution. It is estimated that the immersion depth and interaction radius were 1200 nm and 300 nm at 10 kV, and 15 nm and 5 nm at 1 kV, respectively. This means that a fine surface structure in the order of 100 nm cannot be obtained at a higher acceleration voltage. Currently, many spatial FE-SEMs operate at a lower voltage of 1–3 kV. In addition to observing the surface morphology, we performed elemental mapping using an energy dispersive X-ray spectroscopy (EDS) system (Bruker QUANTAX EDS attached to SU5000).
The original specimen was cut to a size of 10 × 20 mm2 using an electric saw and we smoothened the cut section using the adhesive and the treated plane using an abrasive paper. To prepare the specimens for cross-sectional observation using SEM, we performed ion milling using a Hitachi IM4000 instrument. The acceleration voltage was 6 kV and the ion voltage was 3 kV at an Ar gas flow of 10 mm3/min for 1–3 h.
3. Results and discussion
3.1. Accelerated deterioration test of Open LJ
The effects of three different surface treatment methods (laser, blast, and acid) on the shear strength of the deteriorated adhesives were investigated. The shear strength and statistical dispersion of the undeteriorated Closed LJ were first examined. shows the Weibull plots of the strengths of the laser, blast, and acid treated specimens. The average strength of the laser was the highest (24.1 MPa), which is approximately 10% higher than those of the blast and acid treated specimens. The shape parameter m, which indicates the statistical dispersion, was sufficiently high in all cases and exhibited minimal variations. In this study, the nominal shear strength (fracture load/adhesion area) was used for the shear strength.
Previous study shows the relationship between high temperature immersion and adhesive degradation using Fourier Transform Infrared Spectroscopy (FTIR) measurements.[Citation6,Citation27] shows the relationship between the immersion time of Open LJ in a 63 °C bath and shear strength. The horizontal axis shows the specimens immersed for up to 100 days. Because the initial strength differs with the surface treatment, the vertical axis shows “strength reduction rate (%) = strength after immersion/strength before immersion × 100”. The shear strength continued to decrease until the 10th day of immersion and thereafter remained constant at 20 – 30 % of the initial strength. The trend of strength reduction was the same regardless of the surface treatment. show photographs of the fracture surfaces of the immersed Open LJ. For comparison, the upper row shows photographs of the fracture surface of the not-immersed (initial state) Closed LJ. In the initial state, the adhesive was sound and strong, therefore all the specimens exhibited thin-layer cohesive failure. As deterioration progressed, the strength of the adhesive decreased and the fractured surface moved into the deep adhesive. Cohesive fracture or thin layer cohesive fracture occurred in all the test specimens. In this case, the cohesive fracture indicates the adhesive fracture instead of the adherend fracture. Therefore, no specimen was fractured in the secondary cyanoacrylate adhesive. It was considered that the adhesive strength had more effect on the shear strength than interfacial strength. Therefore, it appears that the trends of strength deterioration were similar. It can be inferred that the fracture of immersed LJ specimens is more related to the degradation of the adhesive than the degradation of the interface.
3.2. Accelerated deterioration test of open DCB
The effects of four different surface treatments (laser, blast, acid, and wipe) on the energy release rate G1C of the deteriorated adhesives were examined. shows the relationship between the 63°C bath immersion time of Open DCB and G1C. In this study, G1C was calculated using the corrected beam theory (CBT) method, as expressed in EquationEquation (1)(1)
(1) , in accordance with the ISO 25,217.[Citation25]
Figure 5. Accelerated deterioration test of Open DCB, (a) relationship between immersion time at 63°C and G1C, (b) strength and elongation of adhesive bulk specimen as a function of immersion time.
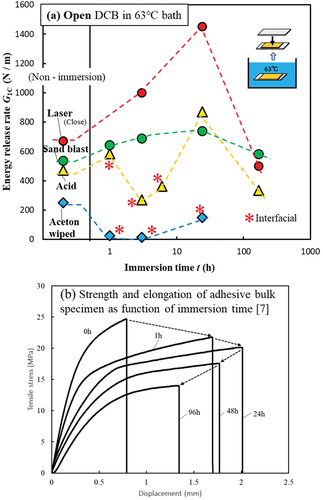
where P is the load, B is the width of the specimen, a is the crack length, ∆ is the crack length correction, δ is the displacement of the beam, F is the large displacement correction, and N is the load block correction. For comparison, the G1C values before immersion are shown in the left column. Before immersion, the laser-treated specimens exhibited the highest G1C values. According to the shear strength of LJ shown in , the strength of the laser specimens was approximately 10% higher than those of the blast and acid specimens; however, the G1C of the laser specimens in the non-immersed DCB test improved by 20–30% compared with those of blast and acid-treated specimens. This is because mode 1 tests (DCB) tend to better represent adhesive strength, including interfacial strength, than mode 2 tests (LJ).[Citation7] The right column of shows the relationship between the immersion times of Open DCB and G1C. The asterisks indicate that the specimens fractured at the interface. The specimens without asterisks are cohesive fractures or thin-layer cohesive fractures. In the case of blast and laser treatments, interface failure did not occur until the end, and no significant decrease in G1C was observed. However, acetone wipe treatment specimen yielded interfacial failure soon after immersion, and G1C decreased significantly. In the case of Open Face specimen test, no specimens fractured on the parting surface (second bond), including case of LJ.
Acid treatment specimen yielded interfacial fracture after a short immersion time; however, it returned to cohesive fracture, and G1C also improved after 24 h. Blast, acid, and laser treatments yielded the same cohesive fracture after 24 h of immersion; however, the G1C of laser-treated specimens significantly improved. The G1C of acid-treated specimens significantly decreased after 3 h of immersion. When high-temperature moisture reaches the adherend, the aluminum surface becomes temporarily unstable.[Citation7,Citation28] Furthermore, the high G1C after 24 h of immersion is due to improved elongation of the adhesive. is the result of previous study[Citation7] shown the relationship between elongation and strength of the adhesive bulk, which has 2 times thickness of the Open DCB. As mentioned in Section 2-3, the Open DCB adhesive absorbs moisture from one side, whereas the adhesive bulk absorbs from both sides. Therefore, the thickness of the bulk was twice that of the DCB adhesive layer to equalize the moisture absorption rate. The adhesive bulk also exhibits maximum elongation at 24 h, after which the elongation and strength decreased.[Citation7] Because of the highest energy required to break the specimen immersed for 24 h, G1C improved.
3.3. Two types of anchor effects by laser processing
shows the fracture surface of the not-immersed and immersed DCB specimens at 63 °C for 24 h. All the not-immersed specimens are shown in the middle row exhibit cohesive fractures. However, in the case of acid and wiped-treated srfaces (polarized images at upper row), a portion of the base material (black area) was exposed, and the interface was locally fractured. In contrast, the adhesive (white area) remained on the entire surface of the laser- and blast-treated surfaces. The photographs in the bottom row of the figure show the ductile fracture surfaces after 24 h of immersion at 63°C. Plasticization of the adhesive progressed. In particular, the fracture surface of laser-treated specimen was far from the interface, which was considered to exhibit a high G1C.
Figure 6. Photographs of fracture surfaces of Open DCB with four types of surface treatments before and after immersion. Upper and middle rows show the fracture surface photographs of the not-immersed specimen and the polarized micrograph of the thin-layer cohesive failure area, respectively. Bottom row shows the fracture surface photograph of specimens after immersion.
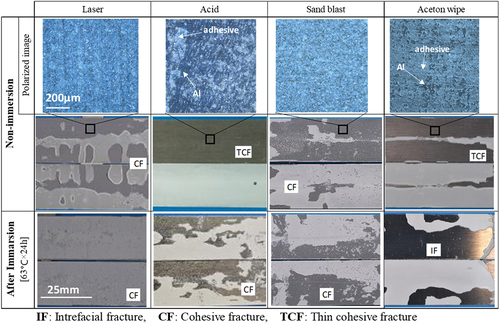
show the SEM images of the adherend surface, surface roughness (Ra), and surface area ratio (surface area/measured area). The surface roughness and surface area ratio were measured using the white confocal of OPTELICS (Lasertec Co., Ltd., Japan). In , the SEM image of the laser treated adherend shows significant unevenness on the surface. When an intense laser beam is shot onto an aluminum surface, the aluminum melts and reagglomerates to form a 3-dimensional shape. When the unevenness area was magnified, dense filament-like structures with diameters of several tens of nanometers were observed on the surface. The atoms were scattered (ablation) by the laser beam and oxidized to form filament-like microaggregates upon redeposition. shows that the SEM image of the blast-treated specimen also has significant unevenness area. The surface roughness of the laser treated specimen was approximately 10 and 3 times those of the acid and blast treated specimens, respectively. The surface area ratio of the laser and blast treated specimens was also larger than that of the acid treated specimen. However, an optical microscope cannot measure surface microfilaments because of its low resolution. In addition, the back side of unevenness could not be measured, resulting in a significantly smaller value than the actual surface area. The surface of the acid specimen in appears smooth, and the surface roughness is very low.
Figure 7. SEM images of adherend surface treated by (a) laser, (b) acid, (c) sandblast, and surface roughness and surface area ratio.
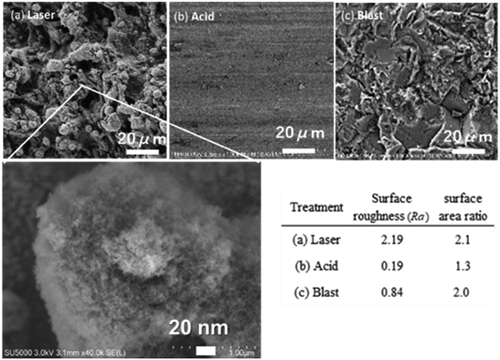
shows the SEM-EDS images of the adhesive interface of the not-immersed DCB. The samples were prepared as explained in Section 2-5. shows the adhesive interfaces of the laser-treated specimens. It is known that surface modification of laser treatment products a hard and dense oxide layer on the surface.[Citation29,Citation30] A thin oxide layer covered the surface and fine filament-like oxides were observed on top of the oxide layer. The EDS image shows that the adhesive (the main component is carbon (yellow) penetrates the filament-like aluminum oxide (red + blue) and cures well. The microanchor effect is expected to create a tough adhesive interface. show the adhesive interfaces of the acid and blast treated specimens, respectively. In both cases, there was no filament-like oxide and the interface was composed of a thin oxide layer and an adhesive. These results suggest that the two adhesive anchoring effects of macro-unevenness and microfilaments contributed significantly to the high G1C of laser specimen.
3.4. Salt spray cycle test
As explained above,[Citation18–21] the results of the salt spray tests demonstrate that laser treatment of metal adherends improves their corrosion resistance. In this study, salt spray tests were conducted for 500 cycles (167 h) in accordance with the JIS Z2371,[Citation31] that is, 1 cycle (8 h) = (salt spray at 35°C for 2 h) + (drying at 60°C for 4 h) + (humid air at 50°C 95% for 2 h). Tensile tests were conducted on 10 or 3 LJ specimens. shows the relationship between the number of cycles and shear strength for blast-, laser-, and acid-treated LJ.
Figure 9. Results of salt spray cycle test, (a) relationship between salt spray cycle number and shear strength of Closed LJ, and fracture surfaces, (b) relationship between cycle number and standard deviation of strength.
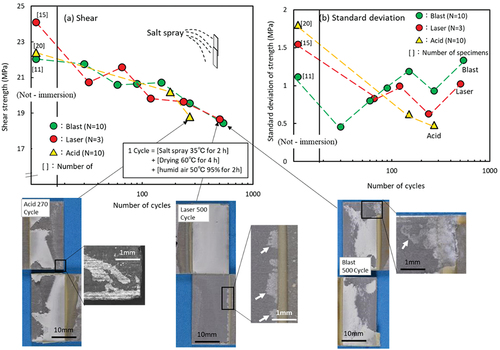
The strength of all the specimens decreased with the number of cycles. Furthermore, all the fracture surfaces exhibited cohesive failure. In the case of the fracture surfaces of “Acid 270Cycle” and “Blast 500Cycle” in the photographs, the adhesive exhibited ductile fracture, and plasticization was observed, whereas for “Laser 500Cycle”, the deterioration of the adhesive was unknown. However, the adherend on the fracture surface showed that corrosion first occurred at 500 cycles for laser- and blast-treated specimens, whereas corrosion of the adherend was confirmed at 270 cycles for acid-treated specimens. Therefore, laser- and blast-treated specimens were slightly more corrosion resistant than acid-treated specimens. As mentioned in 3–3, since the adherend surface was sufficiently oxidized, it seems to act as a protective film and improve corrosion resistance. Anodizing treatment is effective for improving the corrosion resistance of aluminum. However, it has been reported that this technique has a negative effect on strength owing to the initiation and growth of surface cracks.[Citation32] In contrast, laser treatment under certain conditions produces a layer with corrosion resistance.
The dispersion of the deteriorated specimen strength was compared using standard deviation. shows the number of cycles and the standard deviation of intensity. The dispersion became smaller at the start of the test. The adhesive deterioration increased with an increase in the number of cycles, and the dispersion gradually increased. For the blast-treated specimen, plasticization of the adhesive was estimated from the fracture surface observation, and the standard deviation was larger than the initial value.
4. Conclusions
The effect of laser surface treatment on the mechanical strength of adhesion was investigated and compared with that of acid, blast, and wipe treatments. The following conclusions are drawn based on the findings the study.
The average shear strength of laser-treated LJ was 10 % higher than those of the other treatments, however, G1C of DCB was 30 % higher than those of the other specimens. The dispersion was similar for all the treatment conditions, and the Weibull function shape parameter ranged from 13 to 18.
When Open LJ was tested to accelerate degradation by immersion at 63 °C, the laser-treated specimens exhibited the same shear strength reduction behavior as those of acid-and blast-treated specimens.
When Open DCB adhesives were degraded in water at 63 °C, the laser-treated specimens did not exhibit interfacial fracture in the long term, however, the acid- and acetone-treated specimens exhibited interfacial fracture at the early stage. The G1C value of laser-treated specimen was higher than those of acid, blast, and wipe.
In the salt spray cyclic test, the strength of all the specimens decreased with time; however, the laser- and blast-treated adherends exhibited slower corrosion progression.
The effect of surface treatment on strength was more improved in the mode I-based DCB than in the mode II-based LJ.
SEM observation of the interface of the fractured surface revealed that the laser exhibited dense microfillaments on the surface, which held the adhesive well.
The adhesive strength of the laser-treated specimen was enhanced by the two anchor effects of macro unevenness and microfilaments on the adherend surface.
Disclosure statement
No potential conflict of interest was reported by the author(s).
Additional information
Funding
References
- Da Silva, L. F. M.; Öchsner, A.; Adams, R. Handbook of Adhesion Technology. Springer. 2011. ISBN 9778-3-642-01168-9. DOI: 10.1007/978-3-642-01169-6.
- Horiuchi, S.; Terasaki, N.; Miyamae, T. Interfacial Phenomena in Adhesion and Adhesive Bonding, 1st ed.; 2024; Singapore: Edition Springer, 2023.
- Silva, L. F. M.; Sato, C. Design of Adhesive Joints Under Humid Conditions; Springer: Berlin, Heidelberg, 2013; pp. 1–19. DOI: 10.1007/978-3-642-37614-6.
- Machado, J. J. M.; Hayashi, A.; Nunes, P. D. P.; Marques, E. A. S.; Carbas, R. J. C.; Sato, C.; da Silva, L. F. M. Strain Rate Dependence of a Crash Resistant Adhesive as a Function of Temperature for the Automotive Industry. Proc. Inst. Mech. Eng. Part L. 2019, 233(11), 2189–2203. DOI: 10.1177/1464420719836914.
- Houjou, K.; Shimamoto, K.; Akiyama, H.; Sato, C. Effect of Cyclic Moisture Absorption/Desorption on the Strength of Epoxy Adhesive Joints and Moisture Diffusion Coefficient. J. Adhes. 2021, 98(11), 1535–1551. DOI: 10.1080/00218464.2021.1926242.
- Houjou, K.; Shimamoto, K.; Akiyama, H.; Sato, C. Effect of Test Temperature on the Shear and Fatigue Strengths of Epoxy Adhesive Joints. J. Adhes. 2022, 98(16), 2599–2617. DOI: 10.1080/00218464.2021.1982707.
- Houjou, K.; Sekiguchi, Y.; Shimamoto, K.; Akiyama, H.; Sato, C. Energy Release Rate and Crack Propagation Rate Behaviour of Moisture-Deteriorated Epoxy Adhesives Through the Double Cantilever Beam Method. J. Adhes. 2023, 99(6), 1016–1030. DOI: 10.1080/00218464.2022.2074295.
- Houjou, K.; Shimamoto, K.; Akiyama, H.; Sato, C. Dependence of Fatigue Limit on Stress Ratio and Influence of Cyclic Stress on Shear Strength for an Adhesive Lap Joint. J. Adhes. 2021, 97(12), 1153–1165. DOI: 10.1080/00218464.2020.1738934.
- Houjou, K.; Akiyama, C.; Sato, H.; Sato, C. Experimental Investigations on the Effect of a Wide Range of Strain Rates on Mechanical Properties of Epoxy Adhesives, and Prediction of Creep and Impact Strengths. J. Adhes. 2020, 98(5), 449–463. DOI: 10.1016/j.polymertesting.2022.107821.
- Houjou, K.; Akiyama, H.; Sato, C. Fatigue Fracture Behavior of Cured Epoxy Adhesive Containing a Surface Crack. Polym. Tast. 2023, 117, 107821. DOI: 10.1016/j.polymertesting.2022.107821.
- Arikan, E.; Holtmannspötter, J.; Zimmer, F.; Hofmann, T.; Gudladt, H.-J. The Role of Chemical Surface Modification for Structural Adhesive Bonding on Polymers-Washability of Chemical Functionalization without Reducing Adhesion. Int. J. Adhes. Adhes. 2019, 95, 102409. DOI: 10.1016/j.ijadhadh.2019.102409.
- Sato, T.; Ise, S.; Horiuchi, S.; Akiyama, H.; Miyamae, T. Influences of Low Temperature Ambient Pressure N 2 Plasma and Flame Treatments on Polypropylene Surfaces. Int. J. Adhes. Adhes. 2019, 102322(93), 76–82. DOI: 10.1016/j.ijadhadh.2019.01.016.
- Rhee, K. Y.; Yang, J.-H. A Study on the Peel and Shear Strength of Aluminum/CFRP Composites Surface Treated by Plasma and Ion Assisted Reaction Method. Comp. Sci. Technol. 2003, 63-1(1), 33–40. DOI: 10.1016/S0266-3538(02)00145-8.
- Elmarakbi, A. Advanced Composite Materials for Automotive Applications: Structural Integrity and Crashworthiness; John Wiley & Sons: Ltd; 2013. ISBN:9781118423868. DOI: 10.1002/9781118535288.
- Roesner, A.; Scheik, S.; Olowinsky, A.; Gillner, A.; Reisgen, U.; Schleser, M. Laser Assisted Joining of Plastic Metal Hybrids. Physics Procedia. 2011, 12(Part B), 370–377. DOI: 10.1016/j.phpro.2011.03.146.
- Rodríguez-Vidal, E.; Sanz, C.; Soriano, C.; Leunda, J.; Verhaeghe, G. Effect of Metal Micro-Structuring on the Mechanical Behavior of Polymer–Metal Laser T-Joints. J. Mater. Pro. Technol. 2016, 229, 668–677. DOI: 10.1016/j.jmatprotec.2015.10.026.
- Harder, S.; Schmutzler, H.; Hergoss, F. D.; Jetter, F.; Holtmannspötter, J.; Fiedler, B. Effect of Infrared Laser Surface Treatment on the Morphology and Adhesive Properties of Scarfed CFRP Surfaces. Compos. Part A. 2019, 121, 299–307. DOI: 10.1016/j.compositesa.2019.02.025.
- Rechner, R.; Jansen, I.; Beyer, E. Influence on the Strength and Aging Resistance of Aluminium Joints by Laser Pre-Treatment and Surface Modification. Int. J. Adhes. Adhes. 2010, 30, 595–601. DOI: 10.1016/j.ijadhadh.2010.05.009.
- Lippky, K.; Mund, M.; Blass, D.; Dilger, K. Investigation of Hybrid Fusion Bonds Under Varying Manufacturing and Operating Procedures. Compos. Struct. 2018, 202, 275–282. DOI: 10.1016/j.compstruct.2018.01.078.
- Wan, H.; Lin, J.; Min, J. Effect of Laser Ablation Treatment on Corrosion Resistance of Adhesive-Bonded Al Alloy Joints. Surf. Coat. Technol. 2018, 345, 13–21. DOI: 10.1016/j.surfcoat.2018.03.087.
- Min, J.; Wan, H.; Carlson, B. E.; Lin, J.; Sun, C. Chengcheng Sun, Application of Laser Ablation in Adhesive Bonding of Metallic Materials: A Review. Optics & Laser Technol. 2020, 128, 106188. DOI: 10.1016/j.optlastec.2020.106188.
- Wu, Y.; Lin, J.; Carlson, B. E.; Lu, P.; Balogh, M. P.; Irish, N. P.; Mei, Y. Effect of Laser Ablation Surface Treatment on Performance of Adhesive-Bonded Aluminum Alloys. Surf. Coat. Technol. 2016, 304, 340–347. DOI: 10.1016/j.surfcoat.2016.04.051.
- Terasaki, N.; Fujio, Y.; Sakata, Y.; Houjou, K.; Shimamoto, K.; Akiyama, H.; Yase, K.; Horiuchi, S.; Hartwig, S.; Steinberg, J., et al. Effect of Laser Pre-Treatment on Adhesive Joint Performance and Evaluation of Interfacial Strain Distribution Using Mechanoluminescence. J. Adhes. (Accept). DOI: 10.1080/00218464.2024.2313103.
- Wylde, J. W.; Spelt, J. K. Measurement of Adhesive Joint Fracture Properties As a Function of Environmental Degradation. Int. J. Adhes. Adhes. 1998, 18, 237–246. DOI: 10.1016/S0143-7496(98)00028-1.
- ISO. ISO 25217: Adhesives - Determination of the Mode 1 Adhesive Fracture Energy of Structural Adhesive Joints Using Double Cantilever Beam and Tapered Double Cantilever Beam Specimens. 2009.
- ISO 7972: Adhesives - Absorption of Water into an Adhesive Layer Using an Open-Faced Specimen and Determination of Shear Strength by Secondary Bonding. 2023
- Houjou, K.; Shimamoto, K.; Akiyama, H.; Terasaki, N.; Sato, C. Calculation of the Moisture Diffusion Coefficient at the Adhesive Interface of Double Cantilever Beam Specimens by Studying the Fracture Surfaces. Polym. Test. 2023, 125, 108141. DOI: 10.1016/j.polymertesting.2023.108141.
- Eric, X. C.; Jean-François, M.; Che, L. M.; Che, M. Transformations of γ-Alumina in Aqueous Suspensions: 1. Alumina Chemical Weathering Studied As a Function of pH. J. Colloid Inter. Sci. 2007, 308(2), 429–437. DOI: 10.1016/j.jcis.2006.12.074.
- Gnanamuthu, D. S. Laser Surface Treatment. Opt. Eng. 1980, 19(5), 783–788. DOI: 10.1117/12.7972604.
- Takahhi, H.; Fujiwara, K.; SEO, M. The Cathodic Polarization of Aluminum Covered with Anodic Oxide Films in a Neutral Borate Solution–II. Film Breakdown and Pit Formation. Corros. Sci. 1994, 36(4), 689–705. DOI: 10.1016/0010-938X(94)90074-4.
- JIS. JIS Z 2371: Methods of Salt Spray Testing. (Japanese Industrial Standards). 2015.
- Paz Martínez-Viademonte, M.; Abrahami, S. T.; Hack, T.; Burchardt, M.; Terryn, H. A Review on Anodizing of Aerospace Aluminum Alloys for Corrosion Protection. Coatings. 2020, 10(11), 1106. DOI: 10.3390/coatings10111106.