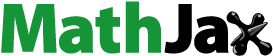
Abstract
Methylcyclohexane (MCH) dehydrogenation is essential for the practical utilization of organic hydrides as hydrogen carriers. Selenium (Se) modification on Pt/TiO2 catalysts has been found to be effective for MCH dehydrogenation in our previous research. To determine the effects of Se modification on the reaction, reaction kinetics analysis was performed. The Se modification onto rutile-type TiO2 (STR, STR-100N) before Pt loading (Pt/Se/STR) showed the highest activity, and even a small amount of Se significantly improved the activity. Although the kinetic analysis of Pt/STR showed that the reaction rate of MCH dehydrogenation decreased at high MCH partial pressures, the reaction rates of Pt/Se/STR and Se/Pt/STR remained the same even at high MCH partial pressures. We found that the inhibition of MCH adsorption onto the catalyst could be prevented by Se modification. This behavior was confirmed by adsorption/desorption evaluations.
1. Introduction
Hydrogen has gained worldwide recognition as a clean and CO2-free secondary energy source. The primary challenge in hydrogen utilization is the development of efficient methods for transporting large quantities of hydrogen because of its low density (Alhumaidan et al. Citation2011; Hassan et al. Citation2021; Sekine & Higo, Citation2021). To transport hydrogen more easily, investigations have been conducted on the storage of hydrogen in alloys (Kojima, Citation2019; Marques et al. Citation2021), ammonia (Lamb et al. Citation2019), and organic chemical hydrides (Modisha et al. Citation2019; Oda et al. Citation2010; Okada et al. Citation2006; Suzuki et al. Citation2021). Among these hydrogen carriers, organic chemical hydrides have emerged as particularly promising owing to their affordability and manageability. These compounds can store and release hydrogen through hydrogenation-dehydrogenation cycles. Toluene (TOL) and methylcyclohexane (MCH), which exhibit liquid phases across a wide temperature range (–95 to 100 °C), are particularly suitable for this application (Sugiura et al. Citation2017). MCH dehydrogenation is an endothermic reaction, as shown in EquationEq. (1)(1)
(1) , which typically requires temperatures of 300–400 °C and a Pt catalyst (Boufaden et al. Citation2016; Sugiura et al. Citation2017). Although Pt-supporting catalysts are effective for dehydrogenation, they are prone to deactivation because of the adsorption of MCH, TOL, and reaction intermediates, as well as carbon deposition (Zhang et al. Citation2011). Therefore, it is crucial to improve the catalytic activity for MCH dehydrogenation.
(1)
(1)
Many studies on catalyst supports and promoters for MCH hydrogenation have reported improvements in catalytic activity (Ouma et al. Citation2021; Shen et al. Citation2017; Wu et al. Citation2021). Although Pt supported on Al2O3 (Pt/Al2O3) showed high activity for MCH dehydrogenation (Alhumaidan et al., Citation2018; Nakano et al. Citation2017), Pt/Al2O3 tended to have low selectivity and a short lifetime. The use of TiO2 instead of Al2O3 could improve the catalytic stability owing to the strong metal-support interaction (SMSI) effect, which suppresses TOL adsorption (Nagatake et al. Citation2016; Yang et al. Citation2020). However, the addition of a sulfur promoter to the catalyst improved its activity for more than 6000 h (Chen et al. Citation2021). In addition, the lifetime of Pt catalysts can be extended by alloying them with Fe and Zn, where Fe facilitates the hydrogenation of hydrocarbons (decoking) and Zn promotes TOL desorption (Nakaya et al. Citation2020). Based on these reports, controlling the electronic state of the Pt catalyst using a support and promoter is a promising way to improve the activity and stability of MCH dehydrogenation.
In our previous study (Ito et al. Citation2022), to control the electronic state of a Pt catalyst, the modification of Se, a chalcogen, with a Pt/TiO2 catalyst was investigated. This modification improved the stability and activity of MCH dehydrogenation, even with a small amount of Se. Although the electron donation effect of Se on the Pt catalyst prevented the inhibition of hydrocarbon adsorption, the complete mechanism for this improvement has not been elucidated.
In this study, a reaction kinetics analysis of MCH dehydrogenation over a Se-modified Pt/TiO2 catalyst was performed to determine the effect of Se addition to the catalyst on MCH dehydrogenation. Therefore, it is essential to establish reaction equations for commercial systems. In addition to kinetic analysis, the effect of Se modification was clarified by measuring the adsorption-desorption characteristics.
2. Experimental
2.1. Catalyst Preparation
As catalyst support, TiO2 (STR-100N, Sakai Chemical Industry Co., Ltd.) was used and is denoted as STR in this study. The structure of STR was rutile (space group: P42/mnm), and its specific surface area was approximately 100 m2 g−1, according to our previous study (Ito et al. Citation2022). Platinum and selenium were loaded onto the support via a sequential impregnation method using Pt(NH3)4(NO3)2 and Na2SeO4 (Fujifilm Wako Pure Chemical Corp.) as precursors. After evaporation, the gel was oven-dried overnight. Calcination was carried out at 450 °C for 120 min under 10% H2/N2 flow. The Pt content was 1 wt% in all catalysts, and the amount of Se was adjusted according to the molar ratio of the Pt content. The Pt catalyst loaded onto Se/TiO2 by the sequential impregnation method is denoted as Pt/Se/STR, and the Pt catalyst before Se impregnation is denoted as Se/Pt/STR.
2.2. Activity test
The catalytic activity tests were performed in a fixed-bed flow reactor. The 30 mg catalyst, which was mixed with 60 mg of SiO2 (CARiACT Q-50, Fuji Silysia Chemical Ltd.) as a dilutant, was charged into the reactor. The catalyst was reduced at 350 °C for 60 min under a 17 vol% H2/N2 flow. The activity test was carried out at 280 °C, and the feed was supplied at MCH/N2 = 0.6/39.4 mL min−1 by bubbling. In Section 3.2, the catalyst weight was changed from 15 mg to 50 mg, and the MCH partial pressure was changed from 1.5 vol% to 20 vol% for kinetic analysis. The product was analyzed using a gas chromatograph equipped with a flame ionization detector (GC-FID) and a thermal conductive detector (GC-TCD).
2.3. Characterization
The diameters of the Pt particles were evaluated by the H2 pulse method using a BELCAT instrument (MicrotracBEL). The catalyst was pre-heated at 10 °C min−1 increments to 250 °C in a diluted H2 flow. When the temperature reached 250 °C, the gas was purged with Ar flow for 60 min. After the temperature was lowered to 50 °C, the H2 pulse method was carried out. The products were detected by a thermal conductivity detector (TCD) using a BELCAT instrument.
The desorption of MCH from the catalyst was evaluated by the temperature-programmed desorption (TPD) technique using a BELCAT instrument. The pre-treatment and the activity test were carried out at 350 °C for 60 min under H2 flow, and then the temperature was lowered to 50 °C. After MCH was supplied by bubbling at 50 °C, the temperature was increased by 10 °C min−1 increments to 350 °C.
3. Results and Discussion
3.1. Effect of the Se Modification on the Pt/STR Catalyst
shows the TOL yield from MCH dehydrogenation over the Se-modified Pt/STR catalysts. Se modification before Pt loading (Pt/Se/STR) significantly improved the catalytic activity. The effect of Se modification on MCH dehydrogenation was also confirmed in our previous study using anatase-type TiO2 (P25) (Ito et al. Citation2022). Because STR is known as rutile-type TiO2, it was confirmed that Se modification was also effective for rutile-type TiO2. summarizes the Pt particle sizes and catalyst activities. Because Se is highly soluble in water, Pt/Se/STR required more Se than Se/Pt/STR. In our previous study, it was confirmed that the actual Se content in the Pt/Se/STR (Se/Pt = 0.2) was almost the same as that in the Se/Pt/STR (Se/Pt = 0.01) due to high Se solubility (Ito et al. Citation2022). Because the Pt particle sizes of Pt/STR, Pt/Se/STR (Se/Pt = 0.04), and Pt/Se/STR (Se/Pt = 0.2) were almost the same, as shown in , the improvement in the catalytic activity by Se modification was caused by the Pt-Se interaction rather than the change in the Pt particle size. Compared to these results, the catalytic activity of Pt/Se/STR (Se/Pt = 1.0), which contained an excess amount of Se, greatly decreased. It is presumed that the Pt-TiO2 interaction was weakened because an excess amount of Se covered the TiO2 support. Actually, Se was highly dispersed on the support according to our previous study (Ito et al. Citation2022). Alternatively, excess Se in the TiO2 pores may have increased the Pt particle size. These considerations suggest that an excess amount of Se decreases the catalytic activity for MCH dehydrogenation. In addition, the catalytic activity of Se/Pt/STR, which was modified by Se on Pt/STR, was lower than that of Pt/STR, regardless of the Se content of Pt/Se/STR (Se/Pt = 0.2). The results showed that the order of Se modification was also important for catalytic activity.
Table 1. Pt diameter in Se-modified Pt/SRT catalysts.
3.2. Kinetics analysis
shows the dependence of the catalytic activity on the MCH partial pressure for the dehydrogenation reaction. The rate of TOL formation on Pt/STR was proportional to MCH partial pressure at 0.1 atm or less, while the formation rate relatively decreased at high MCH partial pressures at 0.1 atm or more. Adsorption inhibits the catalytic performance of MCH dehydrogenation (Murata et al. Citation2021). It was inferred that the inhibition of the adsorption of hydrocarbons, such as MCH or TOL, decreased the reaction rate at high MCH partial pressures on Pt/STR. In contrast, the TOL formation rate remained proportional even at high partial pressures on the Se-modified Pt/STR catalysts. Although the TOL formation rate decreased in the Se/Pt/STR, a proportional relationship was maintained, as shown by the blue square plot in . The results showed that the Se modification maintained the catalytic performance even at high MCH partial pressures.
Figure 2. Dependency of toluene (TOL) formation rate on MCH partial pressure over Se-modified Pt/STR catalysts.

To discuss the kinetic behavior, the reaction rate equation was derived from the elementary reactions of MCH dehydrogenation.
(2)
(2)
(3)
(3)
(4)
(4)
(5)
(5)
Then, the following reaction rate equation was obtained using a quasi-equilibrium approximation that took into account the MCH conversion, with the surface reaction (3) as the rate-determining step:
(6)
(6)
where K represents the adsorption equilibrium constant, and k represents the reaction rate constant. Subscripts M, T, and H represent MCH, TOL, and hydrogen, respectively. Note that the rate equation was formulated assuming the surface reaction (3) as the rate-determining step due to the general model, although the desorption of hydrocarbon became an inhibitor in the MCH dehydrogenation (Akram et al. Citation2020). In addition, although a few reports performed kinetics analysis using a dual-site model (Usman et al., Citation2012), the paper demonstrated kinetics analysis using a single-site model because the report demonstrated that the single-site model worked as well. To evaluate the reaction, a design equation for a plug-flow reactor was used for curve fitting. The results of the analysis are presented in and . As shown in , the MCH conversion, xM, could be fitted well using EquationEq. (6)
(6)
(6) . Although no significant changes were observed in the constants related to TOL and hydrogen in , the adsorption equilibrium constants of MCH and KM and the reaction rate constant, kr, changed for the Se-modified Pt/STR catalysts. As mentioned previously, the Se modification maintained catalytic performance even at a high MCH partial pressure. Kinetic analysis revealed that Se modification (Se/Pt/STR) decreased MCH adsorption, resulting in high catalytic performance even at high MCH partial pressures. In addition, kinetic analysis revealed that Se modification (Pt/Se/STR) promoted the catalytic reaction because the rate constant improved.
Table 2. Kinetic parameters of MCH dehydrogenation derived from .
To validate the kinetic analysis, a similar curve fitting was performed by changing the catalyst weight. The results of the analysis are presented in and . Similarly, Se/Pt/STR exhibited a decrease in the adsorption equilibrium constant of MCH, and Pt/Se/STR exhibited an increase in the reaction rate constant, as shown in . The experiments and analyses supported the adsorption inhibition behavior. In addition, because the reaction rate constant of Pt/Se/STR was improved by Se modification, Se modification contributed to the improvement of the catalytic activity owing to the electron donation effect (Ito et al. Citation2022).
Table 3. Kinetic parameters of MCH dehydrogenation derived from .
3.3. Adsorption and desorption behavior of MCH over Se-Modified Pt/STR catalysts
To evaluate the MCH adsorption and desorption behavior, surface observations were performed using Fourier transform infrared spectroscopy (FT-IR). However, no peaks were observed because the strongly adsorbed MCH did not exhibit IR activity. As an alternative for evaluating the surface state, IR measurements were performed using CO as the probe molecule. shows the IR spectra of CO adsorbed on the Se-modified Pt/STR catalysts. All catalysts showed linear CO adsorption at 2090 cm−1 and bridged CO adsorption at 1800 cm−1 (Ito et al. Citation2022). Although the spectra were similar, the Se modification slightly changed the peak area between the liner and bridge peak. The ratio of the liner and bridge adsorption of Pt/Se/STR, Se/Pt/STR, and Pt/STR was 4.9, 3.4, and 4.5, respectively. Because the ratio of Se/Pt/STR was changed by the Se modification, selenium loaded on Pt/STR covered the Pt surface. On the other hand, the ratio of Pt/Se/STR, which showed high activity, was not changed by the Se modification. The result showed no morphological effect by the Se modification.
The MCH desorption behavior was evaluated using temperature-programmed desorption (TPD). shows the TPD profile of the MCH obtained from the Pt/Se/STR. As no chemisorption peak of MCH on the STR was observed around 300 °C, it was found that MCH was adsorbed on the Pt catalyst. Although the desorption peak of MCH from Pt/STR appeared at approximately 270 °C, that from Pt/Se/STR decreased to approximately 230 °C. The results showed that MCH adsorption on the Pt catalyst was weakened by Se modification. Our previous report revealed that the electronic state of the Pt catalyst changed because of the electron donation effect (Ito et al. Citation2022). It was inferred that the change in the Pt state weakened MCH adsorption, showing high activity even at high MCH partial pressures. This result is consistent with the kinetic analysis, which also confirmed that Se modification alleviated the inhibition of MCH adsorption.
4. Conclusions
To determine the promotional effect of Se modification on Pt/STR, kinetic analysis of MCH dehydrogenation was performed. The adsorption equilibrium constant of MCH was weakened by the addition of Se (Se/Pt/STR), and the rate constant increased (Pt/Se/STR). The change in adsorption behavior was also confirmed by TPD measurements of MCH. The electron donation effect of Se changed the electronic state of the Pt catalyst, resulting in the Se-modified Pt catalyst showing high activity, even at a high MCH partial pressure.
Disclosure statement
No potential conflict of interest was reported by the author(s).
Additional information
Funding
References
- Akram MS, Aslam R, Alhumaidan FS, Usman MR. 2020. An exclusive kinetic model for the methylcyclohexane dehydrogenation over alumina-supported Pt catalysts. Int J Chem Kinet. 52:415–449. doi:10.1002/kin.21360.
- Alhumaidan F, Cresswell D, Garforth A. 2018. Long-term deactivation of supported Pt catalysts in the dehydrogenation of methylcyclohexane to toluene. Ind Eng Chem Res. 49:9764–9770. doi:10.1021/ie1013025.
- Alhumaidan F, Cresswell D, Garforth A. 2011. Hydrogen storage in liquid organic hydride: Producing hydrogen catalytically from methylcyclohexane. Energy Fuels. 25:4217–4234. doi:10.1021/ef200829x.
- Boufaden N, Akkari R, Pawelec B, Fierro JLG, Zina MS, Ghorbel A. 2016. Dehydrogenation of methylcyclohexane to toluene over partially reduced silica-supported Pt-Mo catalysts. J. Mol. Catal. A Chem. 420:96–106. doi:10.1016/j.molcata.2016.04.011.
- Chen X, Gierlich CH, Schötz S, Blaumeiser D, Bauer T, Libuda J, Palkovits R. 2021. Hydrogen production based on liquid organic hydrogen carriers through sulfur doped platinum catalysts supported on TiO2. ACS Sustainable Chem Eng. 9:6561–6573. doi:10.1021/acssuschemeng.0c09048.
- Hassan IA, Ramadan HS, Saleh MA, Hissel D. 2021. Hydrogen storage technologies for stationary and mobile applications: Review, analysis, and perspectives. Renew Sustain Energy Rev. 149:111311. doi:10.1016/j.rser.2021.111311.
- Ito H, Oshima K, Yamamoto T, Ting KW, Toyao T, Sugiyama T, Kato Y, Morita K, Ohashi A, Kishida M. 2022. Improved catalytic stability of Pt/TiO2 catalysts for methylcyclohexane dehydrogenation via selenium addition. Int J Hydrog Energy. 47:38635–38643. doi:10.1016/j.ijhydene.2022.09.055.
- Kojima Y. 2019. Hydrogen storage materials for hydrogen and energy carriers. Int. J. Hydrog. Energy. 44:18179–18192. doi:10.1016/j.ijhydene.2019.05.119.
- Lamb KE, Dolan MD, Kennedy DF. 2019. Ammonia for hydrogen storage; A review of catalytic ammonia decomposition and hydrogen separation and purification. Int J Hydrog Energy. 44:3580–3593. doi:10.1016/j.ijhydene.2018.12.024.
- Marques F, Balcerzak M, Winkelmann F, Zepon G, Felderhoff M. 2021. Review and outlook on high-entropy alloys for hydrogen storage. Energy Environ Sci. 14:5191–5227. doi:10.1039/D1EE01543E.
- Modisha PM, Ouma CNM, Garidzirai R, Wasserscheid P, Bessarabov D. 2019. The prospect of hydrogen storage using liquid organic hydrogen carriers. Energy Fuels. 33:2778–2796. doi:10.1021/acs.energyfuels.9b00296.
- Murata K, Kurimoto N, Yamamoto Y, Oda A, Ohyama J, Satsuma A. 2021. Structure–property relationships of Pt–Sn nanoparticles supported on Al2O3 for the dehydrogenation of methylcyclohexane. ACS Appl Nano Mater. 4:4532–4541. doi:10.1021/acsanm.1c00128.
- Nakano A, Manabe S, Higo T, Seki H, Nagatake S, Yabe T, Ogo S, Nagatsuka T, Sugiura Y, Iki H, et al. 2017. Effects of Mn addition on dehydrogenation of methylcyclohexane over Pt/Al2O3 catalyst. Appl Catal A Gen. 543:75–81. doi:10.1016/j.apcata.2017.06.017.
- Nakaya Y, Miyazaki M, Yamazoe S, Shimizu K, Furukawa S. 2020. Active, selective, and durable catalyst for alkane dehydrogenation based on a well-designed trimetallic alloy. ACS Catal. 10:5163–5172. doi:10.1021/acscatal.0c00151.
- Nagatake S, Higo T, Ogo S, Sugiura Y, Watanabe R, Fukuhara C, Sekine Y. 2016. Dehydrogenation of methylcyclohexane over Pt/TiO2 catalyst. Catal Lett. 146:54–60. doi:10.1007/s10562-015-1623-3.
- Oda K, Akamatsu K, Sugawara T, Kikuchi R, Segawa A, Nakao S. 2010. Dehydrogenation of methylcyclohexane to produce high-purity hydrogen using membrane reactors with amorphous silica membranes. Ind Eng Chem Res. 49:11287–11293. doi:10.1021/ie101210x.
- Okada Y, Sasaki E, Watanabe E, Hyodo S, Nishijima H. 2006. Development of dehydrogenation catalyst for hydrogen generation in organic chemical hydride method. Int J Hydrog Energy. 31:1348–1356. doi:10.1016/j.ijhydene.2005.11.014.
- Ouma CNM, Obodo KO, Modisha PM, Rhyman L, Ramasami P, Bessarabov D. 2021. Effect of chalcogen (S, Se and Te) surface additives on the dehydrogenation of a liquid organic hydrogen carrier system, octahydroindole–indole, on a Pt(111) surface. Appl Surf Sci. 566:150636. doi:10.1016/j.apsusc.2021.150636.
- Sekine Y, Higo T. 2021. Recent trends on the dehydrogenation catalysis of liquid organic hydrogen carrier (LOHC): A review. Top Catal. 64:470–480. doi:10.1007/s11244-021-01452-x.
- Shen LL, Xia K, Lang WZ, Chu LF, Yan X, Guo YJ. 2017. The effects of calcination temperature of support on PtIn/Mg(Al)O catalysts for propane dehydrogenation reaction. Chem Eng J. 324:336–346. doi:10.1016/j.cej.2017.05.058.
- Sugiura Y, Nagatsuka T, Kubo K, Hirano Y, Nakamura A, Miyazawa K, Iizuka Y, Furuta S, Iki H, Higo T, et al. 2017. Dehydrogenation of methylcyclohexane over Pt/TiO2-Al2O3 catalysts. Chem Lett. 46:1601–1604. doi:10.1246/cl.170722.
- Suzuki T, Izato Y, Miyake A. 2021. Identification of accident scenarios caused by internal factors using HAZOP to assess an organic hydride hydrogen refueling station involving methylcyclohexane. J Loss Prev Process Ind. 71:104479. doi:10.1016/j.jlp.2021.104479.
- Usman M, Cresswell D, Garforth A. 2012. Detailed reaction kinetics for the dehydrogenation of methylcyclohexane over Pt catalyst. Ind Eng Chem Res. 51:158–170. doi:10.1021/ie201539v.
- Wu K, Chen F, Wang F, Huang Y, Shen Z, Wang W, Yang Y. 2021. Preparation of Pt supported on mesoporous Mg–Al oxide catalysts for efficient dehydrogenation of methylcyclohexane. Int J Hydrog Energy. 46:25513–25519. doi:10.1016/j.ijhydene.2021.05.056.
- Yang X, Song Y, Cao T, Wang L, Song H, Lin W. 2020. The double tuning effect of TiO2 on Pt catalyzed dehydrogenation of methylcyclohexane. Mol Catal. 492:110971. doi:10.1016/j.mcat.2020.110971.
- Zhang C, Liang X, Liu S. 2011. Hydrogen production by catalytic dehydrogenation of methylcyclohexane over Pt catalysts supported on pyrolytic waste tire char. Int J Hydrog Energy. 36:8902–8907. doi:10.1016/j.ijhydene.2011.04.175.