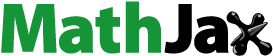
Abstract
Supplementing poultry feed with polyunsaturated fatty acids (PUFAs) can enhance the immunity of poultry and produce poultry products enriched with omega-3 fatty acids (n-3FAs) that are beneficial for consumer health. Thraustochytrids, such as Aurantiochytrium, are known to produce PUFAs. This study aimed to investigate the effect of adding a biomass mixture produced from Hiroshimana old pickle (HOP) and rice vinegar residue (RVR) by Aurantiochytrium sp. strain L3W into the diet of Hirodai-dori chicken, a new meat-type chicken breed established by Hiroshima University, to produce poultry products containing n-3FAs, docosahexaenoic acid (DHA) and eicosapentaenoic acid (EPA). A biomass mixture containing DHA and EPA was produced by culturing the strain L3W, using the solid food wastes, HOP and RVR. Adding this biomass mixture to the poultry diet at 10% significantly increased the DHA content in the liver after the 7th day of the feeding experiment, and the final DHA content increased by 70%. Adding the biomass mixture also maintained the DHA content in the thigh and breast meat, whereas DHA was not present in poultry products in the control experiment with a diet containing no biomass mixture. The ratio of n-6/n-3 fatty acids in the liver was also reduced by adding the biomass to the poultry diet, which is desirable as a healthy source of fatty acids. These findings have demonstrated that a biomass mixture containing Aurantiochytrium sp. strain L3W could be used as a functional eco-feed to increase and maintain the content of n-3FAs in chicken meat.
1. Introduction
Polyunsaturated fatty acids (PUFAs) are classified as n-3 or n-6 based on the location of the last double bond relative to the methyl end (Schmitz and Ecker Citation2008). Flaxseed oil, seafood, and supplemental nutrients such as fish oil, are rich in n-3 fatty acids (n-3FAs) such as docosahexaenoic acid (DHA) and eicosapentaenoic acid (EPA) (Priyadarsini et al. Citation2022). The consumption of sources of n-3FAs has increased owing to their health benefits, such as improving fetal development and cardiovascular function, preventing Alzheimer’s disease, and playing an essential role in regulating immunity (Dinicolantonio and O’Keefe Citation2018; Ibrahim et al. Citation2018; Swanson et al. Citation2012). Recent studies have shown that supplementing poultry feed with n-3FAs can enhance the immunity of poultry (Alagawany et al. Citation2019) and produce poultry products enriched with n-3FAs such as eggs and meat that are beneficial for consumer health (Ló Pez-Ferrer et al. Citation2001; Sefer et al. Citation2011; Yan and Kim Citation2013). Incorporating fish oil into poultry feed has also been found to reduce the n-6/n-3 FAs ratio in breast meat by enrichment of the n-3FAs (Farhoomand and Checaniazer Citation2009). This has been linked to reductions in obesity and inflammation disorders (Zárate et al. Citation2017), indicating the importance of n-3FAs in poultry-feeding systems.
Fish oil is known as a commercial source of n-3FAs for enriching n-3FAs in poultry products (Ló Pez-Ferrer et al. Citation2001). However, fish oil production is highly dependent on marine raw materials. The Food and Agriculture Organization (FAO) (FAO Citation2020) reported that as much as 18 million tons were used to produce fish oil and fish meal. Recent studies have also reported that natural resources and ecosystems are diminishing as the demand for fish increases (Bartek et al. Citation2021; FAO Citation2020). FAO also reported that 34% of fish stocks were over-fished in 2017. Therefore, the dependence on fish oil must be reduced to ensure food security and to achieve a sustainable market. For these reasons, we have focused on using thraustochytrid as a source of n-3FAs.
Thraustochytrids are halophilic and heterotrophic microorganisms that can produce n-3FAs such as DHA and EPA (Nakai et al. Citation2021). Several studies have reported on the effects of using dried pure biomass of thraustochytrids as supplements in poultry diets. A previous study have shown that feeding the biomass of a thraustochytrid, Aurantiochytrium limacinum, to ISA Brown laying hens at a rate of 2.5% resulted in a significant increase in DHA to a total of 410 mg DHA/100 g in their eggs (Moran et al. Citation2020). Other studies have also demonstrated that the biomass of thraustochytrids effectively increases the DHA and EPA contents of the tissues and organs of broiler chickens together with decreasing the n-6/n-3 FAs ratio (Keegan et al. Citation2019a; Keegan et al. Citation2019b; Long et al. Citation2018). For example, adding Aurantiochytrium limacinum biomass to the broiler diet at 1% significantly increased the DHA content to 233, 55, and 86 mg DHA/100 g in the liver, breast, and thigh meat, respectively (Keegan et al. Citation2019b). These results suggest that thraustochytrid biomass can be a viable alternative source to fish oil for n-3FAs for enriching poultry products, although using artificial media for thraustochytrids cultivation may be costly (Rashid et al. Citation2019). Owing to their heterotrophic nature, cultivating thraustochytrids using food wastewater and liquid waste has been widely studied. For example, Humaidah et al., reported that wastewater discharged from washing the bean boiling wastewater could be used to cultivate Aurantiochytrium sp. strain L3W to produce DHA and EPA (Humaidah et al. Citation2020). Waste syrup (Iwasaka et al. Citation2013) and waste sauce (Humaidah et al. Citation2022) were also found to be useful for cultivating thraustochytrids. However, their high viscosity may cause a negative impact on growth (Humaidah et al. Citation2022). Certain types of solid food waste can also be used as a substrate for thraustochytrids. Patel et al., demonstrated that Aurantiochytrium sp. could grow on hydrolyzed post-consumption food waste (Patel et al. Citation2020). More recently, a mixture of Hiroshimana old pickle and sake lees was used for cultivating the strain L3W without using hydrolysis and sterilization treatments, leading to a biomass mixture containing 12.6 mg/g of DHA and 0.217 mg/g of EPA (Suenaga et al. Citation2023). Although the DHA and EPA contents in the biomass mixture were less than that in the fish oil (Ward et al. Citation2022; Yi et al. Citation2014), the DHA and EPA contents were respectively 1500 and 37 times higher than that in commercial poultry feed (Suenaga et al. Citation2023). This biomass mixture was also fed to White Leghorn laying hens, increasing the DHA and EPA contents of the eggs and the DHA content in the breast meat (Asao et al. Citation2023). The transfer efficiency of DHA to the eggs was also greater than that into the meat. However, no study has yet tested the effects of feeding the biomass mixture to meat poultry.
Hirodai-dori is a new breed of meat-type chicken established by Hiroshima University in Japan (Tsudzuki and Nakamura Citation2020). The breed was produced by crossing two native Japanese chicken breeds in the Japanese Avian Bioresource Project Research Center of Hiroshima University. For branding purposes, the content of PUFAs in Hirodai-dori meat may produce an additional value but no study has yet examined using thraustochytrids as a PUFA source in the diet of Hirodai-dori chickens. The present study aimed to investigate the effect of a biomass mixture containing n-3FAs produced by culturing Aurantiochytrium strain L3W using the solid food wastes, Hiroshimana old pickle (HOP) and rice vinegar residue (RVR), on enriching the DHA and EPA contents of the Hirodai-dori chicken meat. The accumulation dynamics of DHA and EPA in the thigh meat, breast meat, and liver will also be investigated to demonstrate the effect of the biomass mixture as a source of PUFAs.
2. Experiment
2.1. Thraustochytrids mass cultivation
We had checked the ability of the thraustochytrid, Aurantiochytrium sp. strain L3W, to grow on solid food waste under unsterile conditions to produce the PUFAs, DHA and EPA (Suenaga et al. Citation2023). The solid waste comprised the substrates, Hiroshimana old pickle (HOP) and rice vinegar residue (RVR), obtained from pickle and seasoning factories, respectively, in Hiroshima, Japan. The components of HOP and RVR are summarized in Table S1. The reason for using HOP and RVR is their difficulty in recycling for other purposes because of their high salinity, and because a previous study confirmed that they can be used as substrates to cultivate the strain L3W (Suenaga et al. Citation2023). One kg (dry weight) of each food waste was crushed using a blender (V1200i, Vitamix, Cleveland, OH, USA) into particles of less than 10 mm, then dispersed in 200 L of artificial seawater prepared by adding a crude salt (Namisho, Diasalt Corp., Tokyo, Japan) to tap water at 30 g/L in a 200-L mass cultivation tank equipped with mixing paddles (Suenaga et al. Citation2023). The strain L3W was then inoculated at 3 × 104 cells/mL into the tank and incubated for 6 d at room temperature. After cultivation, the culture solution was filtered through a filter cloth with a 1-µm mesh to obtain the biomass mixture. The mass cultivation was repeated 6 times, and the biomass mixture was frozen before use. The DHA and EPA contents of each batch of biomass mixture are summarized in .
Table 1. DHA and EPA contents of the biomass mixtures added to the poultry feed.
2.2. Feeding test
The animal protocol for all experiments in the present study was approved by the Hiroshima University Animal Research Committee (Approval No. C21-25). Fifteen Hirodai-dori roosters aged 152 d were purchased from Gallus Japan Ltd. (Hiroshima, Japan). To adapt to the experimental conditions, each rooster was housed in a stainless cage with ad libitum access to a commercial diet (Rayer 17, Chubushiryo Co. Ltd., Nagoya, Japan) and water for 14 d before the feeding test at room temperature with a 14:10-h light: dark cycle. After the adaptation period, the roosters were weighed individually then divided into five groups with a similar body weight. Previous studies investigating the enrichment of n-3FAs in poultry products have used 3–16 hens/group (Keegan et al. Citation2019b). Although a higher replication number would have been better, in the present study the feeding tests were based on 3 individuals/group because of the limited amount of biomass mixture available. Two of the five groups were used to determine the initial and final body weights and fatty acid contents in the control experiment during the 28 d of the experimental period, namely d0/d0C and d28C. The notation d0/d0C stands for chickens from the biomass mixture/control dietary group sacrificed on day 0, and d28C for the control dietary group sacrificed on the 28th day of the feeding experiment. In the control experiment, only the commercial diet was fed, whereas the remaining 3 groups were fed a diet in which the biomass mixture had been added to the commercial diet at 10% dry basis as the DHA and EPA source. The DHA and EPA contents in the commercial diet were 0.0038 and 0.0031 mg/g, respectively. Based on the weighted average DHA and EPA contents in the biomass mixtures (), the DHA and EPA contents in the diet mixture were 2.3 mg DHA/g feed and 0.073 mg EPA/g feed, respectively. This DHA content was comparable with that of diets fed to broiler chickens (0.85–4.26 mg DHA/g feed) (Moran et al. Citation2018), where the dried biomass of Aurantiochytrium limacinum was used as the DHA source ().
Table 2. Comparison of n-3FAs enrichment using thraustochytrids.
During the 28 d of the feeding experiment, the roosters had ad libitum access to the commercial diet or diet mixture and water. Every morning, the remaining feed was weighed to estimate the dietary consumption then replaced with fresh feed. To measure the fatty acid contents, the roosters were periodically euthanized under anesthesia with CO2 gas. After dissection, the right-side thigh (M. biceps femoris) and breast (superficial pectoral muscle) meats without skin, and liver were stored at -80 °C for further analysis of DHA and EPA. The DHA transfer efficiency (%) was then estimated using one chicken per group (n = 3) as follows: Efficiency = (mg DHA/g thigh or breast meat × right-side thigh or breast meat weight × 2) (mg DHA in feed × average daily feed intake × 28 days) × 100.
2.3. Analysis
During the 28 d of the feeding experiment, the environmental temperature and humidity was monitored using a temperature and humidity logger (LR5001, Hioki E.E. Corp., Nagano, Japan). The fatty acid contents of the biomass mixture and commercial diet samples were analyzed using undecanoic acid (Tokyo Chemical Industry, Tokyo, Japan) in n-hexane at 1000 mg/L as an internal standard. After lyophilizing the samples, the internal standard was added at 10 µl/200 mg then the biomass pellet was methylated and purified using the fatty acids methylation-purification kit (Nacalai-Tesque, Kyoto, Japan). Finally, the derivatized sample was analyzed using a gas chromatograph with a flame ionization detector (7820 A GC/FID, Agilent Technologies Inc., Santa Clara, CA, USA), with the Supelco 37-component FAME mix (Sigma-Aldrich, St Louis, MO, USA) being used as the standards of methylated DHA and EPA. The DHA and EPA in the thigh and breast meat, and liver samples were also analyzed by Bureau Veritas FEC Co. Ltd. (Shimane, Japan) in accordance with the Food Labeling Standards (Consumer Affairs Agency 2020). For the statistical analyses of the feed intake and FA contents in the samples of thigh and breast meats, and liver, a one-way analysis of variance (ANOVA) followed by Tukey’s test was conducted to determine the significance of the effects of feeding the biomass mixture using Microsoft Excel with p < 0.05 as significant.
3. Results and Discussion
3.1. Feed intake and body weight performance
During the feeding experiment, the temperature and relative humidity were between 12 and 25 °C and between 60% and 91%, respectively (Figure S1), and no health problems occurred. shows that there was no significant difference in the feed intake between the dietary treatments (p > 0.05). The weight of the whole body, thigh, breast, and liver tissues were not affected by adding the biomass mixture to the diet (, p > 0.05), thereby confirming that the mixture of RVR, HOP, and biomass of the L3W strain had not inhibited the dietary intake and growth of the chickens. However, the DHA and EPA intake of the Hirodai-dori chickens respectively increased 605 times and 25 times by adding the biomass mixture (, p < 0.05). This might be due to the much higher DHA and EPA contents in the biomass mixture () compared with that in the commercial feed containing the fish powder (0.0038 mg DHA/g and 0.0031 mg EPA/g).
Figure 1. Weights of (a) body and (b) body parts of Hirodai-dori chickens during the feeding experiment. Bars indicate standard deviation (n = 3).
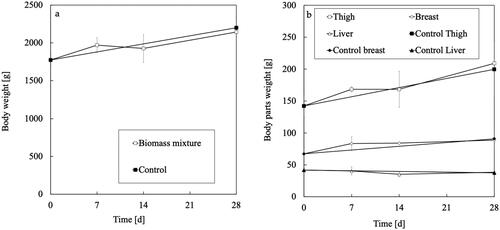
Figure 2. Intake of (a) DHA and (b) EPA by the Hirodai-dori chickens during the 28 d of the feeding experiment. Bars indicate standard deviation (n = 3).
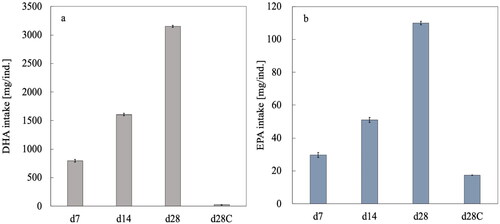
Table 3. Average feed intake of the Hirodai-dori chickens during the 28 d of the feeding experiment.
3.2. Enrichment of polyunsaturated fatty acids in the meat of Hirodai-dori chickens
shows the DHA content in the thigh and breast meat and the liver. In the control experiment, no DHA was detected (< 0.005 g DHA/g) in the thigh and breast meat, and the DHA content of the liver also decreased by the end of the feeding experiment. During the 28 d of the experimental period, the average body weight of the Hirodai-dori chickens increased from 1800 to 2200 g in the control group and to 2143 g in the added biomass group (), but the difference was not significant. This is in contrast with the increase in body weight of more than 1.2 times, and the difference in the average feed intake in the added biomass group between the first 7 d (102 g/d) and the last 14 d (106 g/d) of less than 4%. Although the change in the feed intake of the control group was not measured, the increase in the amount diet ingested was negligible compared with that in the body weight of the Hirodai-dori chickens. This suggests that Hirodai-dori chickens require a higher intake of DHA and its precursors to maintain the DHA level in their thigh and breast meat and liver as they grow. In contrast, supplementing the feed with a biomass mixture containing 2.3 mg DHA/g led to a gradual increase in the DHA content in the liver tissue, where a significant difference appeared on the 7th day (p < 0.05), although the DHA content was maintained rather than increased in the thigh and breast meat in the added biomass group. The DHA content in the liver also finally increased by approximately 70% compared with the initial value. Because of the large error bars, the difference in DHA content between the 14th and 28th days was not significant. This phenomenon might be caused by the lipid metabolism in poultry varying between individuals due to genetic differences (Hermier Citation1997). However, these results showed that adding the biomass mixture to the poultry diet at 10% did increase the DHA content of the chicken livers and maintain the DHA content in the thigh and breast meat.
Figure 3. Changes in the DHA content in the thigh and breast meat, and liver of the Hirodai-dori chicken during the 28 d of the feeding experiment. Bars indicate standard deviation (n = 3).
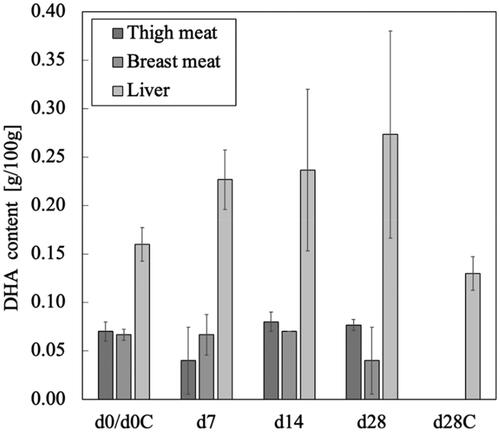
The DHA content in the liver exceeded those in the thigh and breast meat throughout the experimental period in both the control and the added biomass dietary groups. This result agrees with previous studies investigating DHA enrichment by adding thraustochytrid biomass to the poultry diet (Keegan et al. Citation2019b; Moran et al. Citation2018). The higher content of DHA in the liver may be due to it playing an essential role in lipid metabolism, where it is the primary site of de novo lipogenesis before distribution through all the body parts (Neijat et al. Citation2017).
However, no EPA was detected in any body part samples. The EPA intake by the Hirodai-dori chickens was much lower than that of DHA (). Because of this low EPA intake, the eicosanoid metabolism where arachidonic acid (n-6) and EPA (n-3) are combined with the glycerol backbone of phospholipids or glycerides (Schmitz and Ecker Citation2008) might dominate so that EPA does not accumulate. It was also expected that EPA might be metabolized into longer-chain metabolites, such as DHA, via the PUFA metabolic pathway (Lee et al. Citation2019; Schmitz and Ecker Citation2008). In fact, no EPA was detected in the liver (), where de novo lipogenesis occurred before the lipids were distributed to the other body parts. These might also be the reasons why EPA has not been confirmed to be present in the body tissue of Hirodai-dori chickens.
In previous studies, broilers have been fed thraustochytrid biomass such as A. limacinum (Keegan et al. Citation2019a; Keegan et al. Citation2019b; Moran et al. Citation2018) and Schizochytrium limacinum (Long et al. Citation2018). Regarding the DHA deposition patterns, shows that the DHA content of the thigh meat of Hirodai-dori chickens (76.67 mg/100 g) where DHA was fed at 2.3 mg/g diet for 28 d was comparable with the contents of 86.0 mg/100 g and 46.40 mg/100 g of DHA in the thigh meat in ROSS 308 broilers fed A. limacinum biomass at 1.48 mg DHA/g feed for 42 d (Keegan et al. Citation2019b) and at 1.50 mg DHA/g feed for 21 d (Keegan et al. Citation2019a), respectively. However, the Arbor Acres broiler has exhibited the deposition of DHA in its thigh meat at a higher level by ingesting S. limacinum biomass for 42 d. In the present study, as the feeding experiment progressed, the quantity of fatty acids found in the breast meat of the Hirodai-dori chickens decreased, suggesting that a greater intake of DHA leads to a lower overall fatty acid content in the breast meat. These findings agree with previous research which demonstrated that ROSS 308 broiler chickens fed a diet with high levels of DHA will have reduced levels of total fatty acids in their breast meat, but a higher concentration of DHA (Keegan et al. Citation2019a; Keegan et al. Citation2019b). This implies that the quantity of DHA present in the meat is not solely determined by the total fatty acid content, but also by the amount of DHA that is ingested through the diet. However, in the present study, the DHA content of the Hirodai-dori breast meat did not change during the feeding experiment, indicating that it might be less sensitive to DHA compared with ROSS 308 broiler chickens: the DHA content of the Hirodai-dori breast meat (40 mg/100 g) was lower than that of the ROSS 308 broiler (50.1 and 42.11 mg/100 g) fed a diet with a lower DHA content (1.50 mg DHA/g feed) for a shorter period (21 d) with almost the same daily feed intake (103–115 g/day) (Keegan et al. Citation2019a; Keegan et al. Citation2019b). The DHA deposition in the Hirodai-dori liver was similar to that of ROSS 308 broiler chicken liver, indicating that the Hirodai-dori liver may be more sensitive to DHA or require less DHA compared with the livers of ROSS 308 broiler chickens. The DHA transfer efficiency from the diet to the thigh meat of Hirodai-dori chickens (5.1%) was higher than that to its breast meat (1.1%), whereas the difference in efficiency between the thigh and breast meat of Ross 308 broilers (Keegan et al. Citation2019b) was smaller ().
shows that the EPA content of the thigh and breast meat of the ROSS 308 broilers increased with an increasing content of thraustochytrid biomass (Keegan et al. Citation2019a; Keegan et al. Citation2019b), whereas no EPA was detected in the Hirodai-dori chickens. The differences in the EPA deposition behaviors cannot be discussed because the EPA content of the diets fed to the ROSS 308 broilers was not reported. However, our results suggest that the Hirodai-dori chicken is less sensitive to EPA or has a higher requirement for EPA but easily metabolizes it as no EPA was present in the liver.
3.3. FA composition
provides an overview of the effect of adding the biomass mixture on the FA composition in the breast and thigh meat, and liver. FAs are classified into four groups, saturated fatty acids (SFA), monounsaturated fatty acids (MUFA), n-6FAs, and n-3FAs. Commercial feed mainly provided SFAs with stearic acid accounting for 33% and a MUFA, oleic acid, accounting for 23%, followed by linoleic acid, an n-6FA accounting for 20%. The fourth highest component was the n-3FA, α-linolenic acid, accounting for 3%, while no DHA or EPA was detected in the commercial feed. The biomass mixture had a different FA composition: oleic acid (47%), followed by palmitic acid (24%) and DHA (10.9%).
Figure 4. Composition of fatty acids in commercial feed, biomass mixture, thigh and breast meat and liver of Hirodai-dori chickens. SFA = saturated fatty acid; MUFA = monounsaturated fatty acid; Omega-6 FA= n-6 fatty acid; Omega-3 FA = n-3 fatty acid.
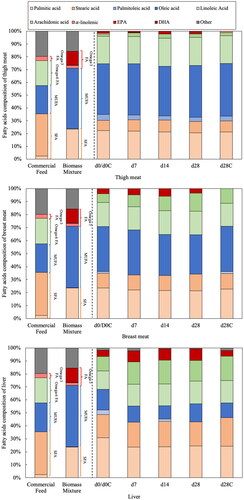
The FAs in the thigh and breast meat in the control samples (d0/d0C and d28C) were mostly made up of MUFAs consisting of oleic and palmitoleic acids, with the second group being the SFAs, palmitic and stearic acids. Omega-6FAs were the third group consisting of linoleic and arachidonic acids, while the important n-3FAs, α-linolenic acid and DHA, accounted for less than 3%. However, by feeding the biomass mixture, the contribution of n-3FAs in the thigh and breast meat was maintained at 2% and 4%, respectively. These values were higher than that in the control, whereas the MUFAs, SFAs and n-6FAs compositions did not change. In contrast, in the liver, SFAs were the most dominant in both experimental groups, and n-6FAs and MUFAs were the second and third groups, respectively. DHA was the major component in the n-3FAs in both experimental groups. Although the contribution of DHA did not change in the control (d0/d0C and d28C), feeding the biomass mixture resulted in an increase in the DHA contribution from 4% to 9% (p < 0.05). Similar to the thigh and breast meat, the compositions of SFAs, MUFAs and n-6FAs in the liver were not affected by feeding the biomass mixture. This resulting comparative reduction in the ratio of n-6FAs to n-3FAs might be beneficial to human health (Fernandes et al. Citation2014; Zárate et al. Citation2017).
An increase in DHA and decrease in SFA can be beneficial for human health. For example, a higher intake of DHA along with a reduction in SFA intake has been reported to improve cardiovascular function (Astrup et al. Citation2020; Dinicolantonio and O’Keefe Citation2018). It has also been recommended that the ratio of n-6FAs to n-3FAs in food should be less than 5:1 for optimal health (Fernandes et al. Citation2014). Increasing this ratio is known to contribute to the development of various diseases (Simopoulos Citation2016; Simopoulos Citation2004; Simopoulos Citation2002; Zárate et al. Citation2017). Unfortunately, modern diets have shifted from an ideal 1–4:1 ratio in prehistoric times to an unhealthy 20:1 or even higher due to the increased consumption of n-6FAs in industrialized societies (Simopoulos Citation2016). summarizes the n-6/n-3 ratio in the meat and liver samples in the present study. The n-6/n-3 ratios in the thigh meat and liver were reduced (p < 0.05) by feeding the biomass mixture, whereas it could not be calculated for the breast meat because no n-3FAs were detected in the control group. In contrast, a reduction in DHA content in all the body part samples occurred in the control group where only the commercial feed was fed. Adding the biomass mixture also reduced the n-6/n-3 ratio to a desirable level in the liver tissue, from 8.83:1 to 4.03:1. In addition, the d22esirable n-6/n-3 ratio was given for the breast meat by feeding the biomass mixture. Furthermore, feeding the biomass mixture decreased the n-6/n-3 ratio in the thigh meat. These results have shown that adding the biomass mixture to the diet can reduce the n-6/n-3 ratio in poultry products. In particular, the ratio, 4.03:1, in the liver tissue makes it desirable as a source of healthy fatty acids. A previous study suggested that adding Schizochytrium limacinum at a rate of 2% to the diet of Arbor Acres broiler chickens decreased the n-6/n-3 ratio in the thigh meat from 10.9 to 1.92 (Long et al. Citation2018). Supplementing a broiler diet with A. limacinum biomass at a rate of 1% significantly reduced the n-6/n-3 ratio in the thigh meat and liver of broilers, from 12.7 to 7.7 and from 11.1 to 3.9, respectively (Keegan et al. Citation2019b).
Table 4. Omega-6/omega-3 ratios in the meat and liver samples of Hirodai-dori chickens after 28 d of the feeding experiment.
4. Conclusion
This study used RVR and HOP as substrates to cultivate the Aurantiochytrium sp. strain L3W. The resulting biomass mixture, containing DHA and EPA, was then added to a commercial diet at a rate of 10% and fed to 152-day-old Hirodai-dori chickens for 28 d. Adding the biomass mixture to the poultry diet significantly increased the DHA content in the liver by the 7th day of the feeding experiment and by the end of feeding experiment had enhanced it by 70.83%. Adding the biomass mixture also maintained the DHA levels in both the thigh and breast meat, whereas in the control group fed only the commercial diet, the DHA content in all the body part samples decreased. This might be due to the negligible increase in the amount of diet ingested compared with the increase in the body weight of the Hirodai-dori chicken. Adding the biomass mixture also reduced the n-6/n-3 ratio in the liver tissue to a desirable value (from 8.83:1 to 4.03:1). In addition, the desirable n-6/n-3 ratio was given for the breast meat by feeding the biomass mixture. Furthermore, feeding the biomass mixture decreased the n-6/n-3 ratio in the thigh meat and positively affected the breast meat. These findings have demonstrated that a thraustochytrid biomass mixture could be used as a functional eco-feed to raise or maintain the content of n-3FA in chicken meat. However, no EPA was found in the thigh and breast meat, and liver. This indicated that the Hirodai-dori chicken was less sensitive to EPA enrichment than other types of meat chicken, such as the ROSS 308 broiler. The effects of DHA and EPA in the Hirodai-dori chicken body after ingestion need to be better understood to reduce the n-6/n-3 ratio in chicken meat to healthier levels and should be considered in future studies.
Supplemental Material
Download MS Word (121.3 KB)Acknowledgments
This work was supported by Japan Science and Technology Agency (JST) as part of SICORP, Grant Number JPMJSC21E8. We thank Philip Creed, PhD, from Edanz (https://jp.edanz.com/ac) for editing a draft of this manuscript.
Disclosure statement
All authors declare no conflicts of interest.
References
- Alagawany M, Elnesr SS, Farag MR, Abd El-Hack ME, Khafaga AF, Taha AE, Tiwari R, Yatoo MI, Bhatt P, Khurana SK, et al. 2019. Omega-3 and omega-6 fatty acids in poultry nutrition: Effect on production performance and health. Animal. 9:573–592. doi:10.3390/ani9080573.
- Asao W, Nii T, Nishijima W, Gotoh T, Humaidah N, Nakai S. 2023. Application of an eco-feed produced by culturing Aurantiochytrium sp. strain L3W using solid food wastes for production of w-3 fatty acids-enriched poultry products running title: eco-feed for w-3 fatty acids-enriched products. J Mater Cycles Waste Manag. 25:3346–3354. doi:10.1007/s10163-023-01757-x.
- Astrup A, Magkos F, Bier DM, Brenna JT, de Oliveira Otto MC, Hill JO, King JC, Mente A, Ordovas JM, Volek JS, et al. 2020. Saturated fats and health: a reassessment and proposal for food-based recommendations: JACC state-of-the-art review. J Am Coll Cardiol. 76:844–857. doi:10.1016/j.jacc.2020.05.077.
- Bartek L, Strid I, Henryson K, Junne S, Rasi S, Eriksson M. 2021. Life cycle assessment of fish oil substitute produced by microalgae using food waste. Sustain Prod Consum. 27:2002–2021. doi:10.1016/j.spc.2021.04.033.
- Consumer Affairs Agency. J.: Food labeling guideline, https://www.caa.go.jp/policies/policy/food_labeling/food_labeling_act/pdf/food_labeling_cms101_200327_11.pdf.
- Dinicolantonio JJ, O’Keefe JH. 2018. Importance of maintaining a low omega-6/omega-3 ratio for reducing inflammation. Open Heart. 5:e000946. doi:10.1136/openhrt-2018-000946.
- FAO. 2020. The state of world fisheries and aquaculture. doi:10.4060/ca9229en.,
- Farhoomand P, Checaniazer S. 2009. Effects of graded levels of dietary fish oil on the yield and fatty acid composition of breast meat in broiler chickens. J Appl Poult Res. 18:508–513. doi:10.3382/japr.2008-00137.
- Fernandes CE, Vasconcelos MADS, De Almeida Ribeiro M, Sarubbo LA, Andrade SAC, Filho ABDM. 2014. Nutritional and lipid profiles in marine fish species from Brazil. Food Chem. 160:67–71. doi:10.1016/j.foodchem.2014.03.055.
- Hermier D. 1997. Conference: avian lipoprotein metabolism: an update lipoprotein metabolism and fattening in poultry 1. J Nutr. 127:805–808.
- Humaidah N, Nakai S, Nishijima W, Gotoh T. 2022. Utilization of saline and viscous food-processing liquid waste for cultivation of thraustochytrid for production of polyunsaturated fatty acids. Clean Technol Environ Policy. 24:2739–2748. doi:10.1007/s10098-022-02348-4.
- Humaidah N, Nakai S, Nishijima W, Gotoh T, Furuta M. 2020. Application of Aurantiochytrium sp. L3W for food-processing wastewater treatment in combination with polyunsaturated fatty acids production for fish aquaculture. Sci Total Environ. 743:140735. doi:10.1016/j.scitotenv.2020.140735.
- Ibrahim D, El-Sayed R, Khater SI, Said EN, El-Mandrawy SAM. 2018. Changing dietary n-6:n-3 ratio using different oil sources affects performance, behavior, cytokines mRNA expression and meat fatty acid profile of broiler chickens. Anim Nutr. 4:44–51. doi:10.1016/j.aninu.2017.08.003.
- Iwasaka H, Aki T, Adachi H, Watanabe K, Kawamoto S, Ono K. 2013. Utilization of waste syrup for production of polyunsaturated fatty acids and Xanthophylls by Aurantiochytrium. J Oleo Sci. 62:729–736. doi:10.5650/jos.62.729.
- Keegan JD, Currie D, Knox A, Moran CA. 2019. Redressing the balance: including DHA-rich Aurantiochytrium limacinum in broiler diets increases tissue omega-3 fatty acid content and lowers the n-6:n-3 ratio. Br Poult Sci. 60:414–422. (a) doi:10.1080/00071668.2019.1605153.
- Keegan JD, Fusconi G, Morlacchini M, Moran CA. 2019. Whole-life or fattening period only broiler feeding strategies achieve similar levels of omega-3 fatty acid enrichment using the DHA-rich protist, Aurantiochytrium limacinum. Animals. 9:327. (b) doi:10.3390/ani9060327.
- Lee SA, Whenham N, Bedford MR. 2019. Review on docosahexaenoic acid in poultry and swine nutrition: Consequence of enriched animal products on performance and health characteristics. Anim Nutr. 5:11–21. doi:10.1016/j.aninu.2018.09.001.
- Ló Pez-Ferrer S, Baucells MD, Barroeta AC, Grashorn MA. 2001. n-3 Enrichment of Chicken Meat. 1. Use of Very Long-Chain Fatty Acids in Chicken Diets and Their Influence on Meat Quality: Fish Oil. Poult Sci. 80:741–752. doi:10.1093/ps/80.6.741.
- Long SF, Kang S, Wang QQ, Xu YT, Pan L, Hu JX, Li M, Piao XS. 2018. Dietary supplementation with DHA-rich microalgae improves performance, serum composition, carcass trait, antioxidant status, and fatty acid profile of broilers. Poult Sci. 97:1881–1890. doi:10.3382/ps/pey027.
- Moran CA, Keegan JD, Vienola K, Apajalahti J. 2018. Broiler tissue enrichment with docosahexaenoic acid (DHA) through dietary supplementation with Aurantiochytrium limacinum Algae. FNS. 09:1160–1173. doi:10.4236/fns.2018.910084.
- Moran CA, Morlacchini M, Keegan JD, Rutz F, Fusconi G. 2020. Docosahexaenoic acid enrichment of layer hen tissues and eggs through dietary supplementation with heterotrophically grown Aurantiochytrium limacinum. J Appl Poult Res. 29:152–161. doi:10.1016/j.japr.2019.10.002.
- Nakai S, Das A, Maeda Y, Humaidah N, Ohno M, Nishijima W, Gotoh T, Okuda T. 2021. A novel strain of Aurantiochytrium sp. strain L3W and its characteristics of biomass and lipid production including valuable fatty acids. J Wat Environ Tech. 19:24–34. doi:10.2965/jwet.20-087.
- Neijat M, Eck P, House JD. 2017. Impact of dietary precursor ALA versus preformed DHA on fatty acid profiles of eggs, liver and adipose tissue and expression of genes associated with hepatic lipid metabolism in laying hens. Prostaglandins Leukot Essent Fatty Acids. 119:1–17. doi:10.1016/j.plefa.2017.01.010.
- Patel A, Rova U, Christakopoulos P, Matsakas L. 2020. Mining of squalene as a value-added byproduct from DHA producing marine thraustochytrid cultivated on food waste hydrolysate. Sci Total Environ. 736:139691. doi:10.1016/j.scitotenv.2020.139691.
- Priyadarsini M, Raichel Nivetha X, Mathimani T, Anto S, Hareesh Krishnan H, Glivin G, Premalatha M, Mariappan V, Sekhar J. 2022. Omega-3-fatty acids from algae for health benefits. Mater Today Proc. 66:1514–1518. doi:10.1016/j.matpr.2022.07.177.
- Rashid N, Selvaratnam T, Park WK. 2019. Resource recovery from waste streams using microalgae: Opportunities and threats. In A. Yousuf, editor. Microalgae Cult Biofuels Prod. Cambridge (MA): Academic Press. p. 337–351.
- Schmitz G, Ecker J. 2008. The opposing effects of n-3 and n-6 fatty acids. Prog Lipid Res. 47:147–155. doi:10.1016/j.plipres.2007.12.004.
- Sefer D, Andonov A, Sobajic S, Markovic R, Radulovic S, Jakic-Dimic D, Petrujkic B. 2011. Effects of feeding laying hens diets supplemented with omega 3 fatty acids on the egg fatty acid profile. Bio Anim Husb. 27:679–686. doi:10.2298/BAH1103679S.
- Simopoulos AP. 2002. The importance of the ratio of omega-6/omega-3 essential fatty acids. Biomed Pharmacother. 56:365–379. doi:10.1016/s0753-3322(02)00253-6.
- Simopoulos AP. 2004. Omega-6/omega-3 essential fatty acid ratio and chronic diseases. Food Rev Int. 20:77–90. doi:10.1081/FRI-120028831.
- Simopoulos AP. 2016. An increase in the Omega-6/Omega-3 fatty acid ratio increases the risk for obesity. Nutrients. 8:128. doi:10.3390/nu8030128.
- Suenaga T, Nakai S, Umehara A, Nishijima W, Gotoh T, Humaidah N. 2023. Valorization of solid food waste as a source of polyunsaturated fatty acids using Aurantiochytrium sp. L3W. Waste Biomass Valor. 14:2945–2956. doi:10.1007/s12649-023-02072-0.
- Swanson D, Block R, Mousa SA. 2012. Omega-3 fatty acids EPA and DHA: health benefits throughout life. Adv Nutr. 3:1–7. doi:10.3945/an.111.000893.
- Tsudzuki M, Nakamura T. 2020. Nihondori no kachito burandokeisei -Hirodaidori no torikumi- (Values of the Japanese chicken breed and branding -challenges of Horodai-dori-). Nougyoukyoudoukumiai Keieijitsumu. 75:123–133.
- Ward ED, Thomasson K, Fischer KR. 2022. Analysis of omega-3 fatty acid content in fish oil products. J Pharm Pract. 35:870–873. doi:10.1177/08830738211015051.
- Yan L, Kim IH. 2013. Effects of dietary ω -3 fatty acid-enriched microalgae supplementation on growth performance, blood profiles, meat quality, and fatty acid composition of meat in broilers. J. Appl Anim Res. 41:392–397. doi:10.1080/09712119.2013.787361.
- Yi T, Li SM, Fan JY, Fan LL, Zhang ZF, Luo P, Zhang XJ, Wang JG, Zhu L, Zhao ZZ, et al. 2014. Comparative analysis of EPA and DHA in fish oil nutritional capsules by GC-MS. Lipids Health Dis. 13:190. doi:10.1186/1476-511X-13-190.
- Zárate R, Jaber‐Vazdekis N, Tejera N, Pérez JA, Rodríguez C. 2017. Significance of long chain polyunsaturated fatty acids in human health. Clin Transl Med. 6:25. doi:10.1186/s40169-017-0153-6.