ABSTRACT
The Senecio inaequidens – S. madagascariensis complex (Asteraceae: Senecioneae, subsequently “fireweed complex”) is a group of six southern African species of Senecio, three of which are considered invasive in various parts of the world: S. madagascariensis in South America, Japan, Australia, and the Hawaiian Islands, S. skirrhodon in New Zealand, and S. inaequidens in Mexico and Europe. Morphological plasticity and historically limited access to reliable information in the introduced ranges have led to taxonomic confusion in many countries, where the weedy populations have been classified under changing names and sometimes erroneously considered to be native species. This confusion has hampered research into management options such as biological control. Recently, uncertainty has arisen about whether only one or two species of the fireweed complex are present in New Zealand. To resolve this question and test the utility of multi-locus sequence capture data for the understanding of the complex, we produced a first dataset of hundreds of genes for four of its species with a focus on S. madagascariensis and S. skirrhodon. Both species were found to be present in the North Island of New Zealand, with S. madagascariensis widespread in the Northland region and S. skirrhodon present at both sites sampled in the southern half of North Island. Our results show that sequence capture data provide high levels of confidence and resolution even at shallow phylogenetic levels, which will enable a greater understanding of the fireweed complex and its invasion history as the dataset is expanded.
Introduction
The Senecio inaequidens – S. madagascariensis complex (Asteraceae: Senecioneae, subsequently “fireweed complex”) is a group of six southern African species generally assumed to be closely related and often described as being difficult to distinguish morphologically and potentially taxonomically unresolved (Marohasy Citation1993; Radford et al. Citation2000). They are S. inaequidens DC., S. madagascariensis Poir., S. skirrhodon DC., S. harveianus MacOwan, S. burchellii DC., and S. pellucidus DC. Representatives of this complex are invasive weeds in various parts of the world, including Mexico (Rzedowski et al. Citation2003), South America (López et al. Citation2008), Japan (Tsutsumi Citation2011), Australia (McFadyen and Morin Citation2012; Wijayabandara et al. Citation2022), the Hawaiian Islands (Le Roux et al. Citation2006), and New Zealand (Webb et al. Citation1988) (A).
Figure 1. A, Countries representing the native (black) and approximate introduced (dark grey) range of the fireweed complex. B, Sampling localities in KwaZulu-Natal, South Africa. C, Introduced range and provenance of specimens sequenced in Australia. D, Introduced range and sampling localities in New Zealand. Senecio inaequidens is marked brown, S. skirrhodon blue, and S. madagascariensis yellow. Points with black margin indicate sequenced samples, those without margin geocoded specimens in the Australasian Virtual Herbarium (accessed September 2022).
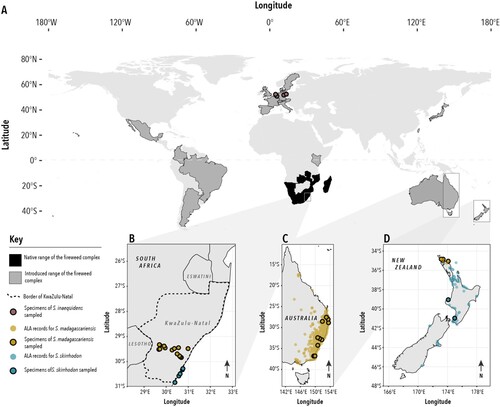
A monograph of the Asteraceae of KwaZulu-Natal, South Africa, (Hilliard Citation1977) has since its publication served as the authoritative taxonomic treatment of the fireweed complex, despite its restricted geographic scope, which also means that it does not provide descriptions of S. burchelli and S. pellucidus and only a rudimentary description of S. harveianus. According to Hilliard’s key and descriptions, S. skirrhodon is a coastal plant with fleshy leaves, S. madagascariensis an annual branching mostly in the upper part of the stem, and S. inaequidens and S. harveianus are perennials branching vigorously from the base. The latter two can be distinguished by their calycular bracts (few and short versus several rows and long). Senecio burchellii and S. pellucidus are easily distinguished from the other four species by only possessing c. 12 involucral bracts and 5–8 ray florets versus c. 20 and c. 13, respectively.
However, Hilliard’s work has some limitations, in particular if it is used without access to reference specimens identified by that author. Not all potentially useful characters from the descriptions are used in the key. For example, the couplet differentiating S. skirrhodon from other species requires the user to know if the species has fleshy leaves, which is difficult to determine from dried herbarium specimens, or if it would be growing on a beach in its natural habitat, which is not necessarily useful in invasive populations outside of its native range. In addition, some characters such as the number and dimensions of calycular bracts are not quantified.
Partly because of these limitations, partly because of the morphological plasticity of the species, especially with regard to growth form and leaf shape, taxonomists and weed researchers in the introduced range have struggled with the identification of invasive fireweed populations and relating them to South African taxa to study their invasion histories and source candidate biological control agents. European fireweed populations introduced with South African wool were variously named S. harveianus, S. reclinatus L.f., and S. vimineus DC., but are now generally named S. inaequidens (Jovet et al. Citation1975; Tutin et al. Citation1976). Argentinean fireweed was first described as a new species, then identified as S. burchelli (Cabrera and Ré Citation1965), and is now named S. madagascariensis. In Mexico, it was misidentified twice, until comparison with European S. inaequidens revealed the two as likely being the same species (Rzedowski et al. Citation2003). Australian fireweed was long considered part of the native S. pinnatifolius A. Rich. (formerly S. lautus Willd.) species complex. It was identified as S. madagascariensis in 1980 (Michael Citation1981) and only widely accepted as introduced after genetic confirmation (Scott et al. Citation1998).
Recently, uncertainty has arisen about the identity of fireweed populations in New Zealand, which have historically all been assigned to S. skirrhodon (Webb et al. Citation1988). Increased concern about the weediness of Senecio species in pastures in the Northland region of New Zealand prompted one of the authors (J.J.D.) to lodge specimens in the Allan Herbarium CHR in May 2017. They were tentatively identified as S. madagascariensis, with the New Zealand online flora listing the species as present (Schönberger et al. Citation2020). A recent review of the distribution of S. madagascariensis considered it to be absent from New Zealand but noted that most of it would be suitable habitat (Wijayabandara et al. Citation2022).
Previous attempts at clarifying the identity of invasive fireweed populations or pinpointing their provenances have used isozymes (Radford et al. Citation2000), chromosome counts and genome sizes (Lafuma et al. Citation2003), microsatellites (Dormontt et al. Citation2014), Amplified Fragment Length Polymorphism data, and ribosomal Internal Transcribed Spacer sequences (Le Roux et al. Citation2006; Lachmuth et al. Citation2010), all of which have limited power of resolution and frequently produce ambiguous results such as large phylogenetic polytomies. Today, high-throughput sequencing and the use of methods such as sequence capture allow the use of marker systems that provide considerably more sequence data and therefore more resolution and higher clade support in phylogenetic analyses (Koenen et al. Citation2020; Schmidt-Lebuhn and Bovill Citation2021).
In this study, we used sequence capture to produce data for hundreds of low-copy nuclear genes to test the hypothesis that two fireweed species occur in New Zealand. In addition, we sampled South African, European, and Australian fireweed occurrences to provide a phylogenetic context for the New Zealand samples and develop a first framework dataset that can be built upon for future research on the taxonomic identity and provenance of other fireweed populations globally.
Materials and methods
Sampling
Sampling for this study focused on populations identified as Senecio madagascariensis, S. skirrhodon, and S. inaequidens in South Africa, Australia, New Zealand, and Europe. South African specimens were collected through dedicated field work in the KwaZulu-Natal area (B), which was previously suggested as the likely area of origin of invasive S. madagascariensis in the Hawaiian Islands and Australia (Scott et al. Citation1998; Le Roux et al. Citation2006), covering multiple locations and morphological forms; vouchers are deposited at the Bews Herbarium, University of KwaZulu-Natal (NU). New Zealand specimens were collected in dedicated field work to represent the two forms of fireweed suggested to occur in the country from different parts of the North Island (D); vouchers are deposited at the Australian National Herbarium (CANB). These specimens are distinct from the three specimens previously deposited at CHR. Australian and European populations were sampled mostly from herbarium specimens at CANB, aiming for broad geographic coverage, including different European countries (A, C). One specimen was newly collected and is vouchered at the CSIRO European Laboratory. Voucher information and Sequence Read Archive accession numbers are summarised in Appendix 1.
To place the fireweed populations into a phylogenetic context, we sampled other representative species of Senecio from South Africa and added data of Asteraceae tribe Senecioneae available from the Plant and Fungal Tree of Life (PAFTOL) and Genomics for Australian Plants (GAP) consortia.
Lab procedures
Genomic DNA was extracted from 5–15 mg silica dried leaf tissue or herbarium material using Invisorb Spin Plant Mini Kit (Stratec, Berlin, Germany) following the manufacturer’s instructions. Libraries were built using the QIAseq FX DNA Library UDI-A Kit 96 (Qiagen, Clayton, Australia) that included a DNA digestion step to a fragment size of approximately 200 base pairs, and sequence capture was conducted on pools of sixteen libraries using the angiosperms353 (Johnson et al. Citation2019) MYbaits kit (Daicel Arbour Biosciences, Ann Arbour, MI, USA). Enriched libraries were sequenced on Illumina NovaSeq 6000 SP with v1.5 paired-end 2 × 150 cycle chemistry.
Bioinformatics
Reads were quality filtered and paired with TRIMMOMATIC (Bolger et al. Citation2014) and assembled using hybpiper-nf (Jackson et al. Citation2021), a Nextflow pipeline adapted from HybPiper (Johnson et al. Citation2016), against a target file designed for broad representation of Asteraceae by mining transcriptome data for angiosperms353 targets (McLay et al. Citation2021).
The results of HybPiper’s paralog finder were analysed with the Monophyletic Outgroups (MO) algorithm as implemented in resolve_paralogs-nf (Jackson et al. Citation2021), a Nextflow pipeline for the four gene-tree based paralogy resolution algorithms collated by Yang and Smith (Citation2014). We chose this algorithm because it returns at most one ortholog group for each locus, producing a more complete sample × gene matrix than alternative algorithms that return more ortholog groups with on average fewer sequences. After paralogy resolution, 326 ortholog groups were retained. Of these, eleven were discarded because they had data for less than ten terminals, leaving 315 that were analysed. Terminals were represented in 25–314 ortholog groups (mean 266), and ortholog groups had data for 10–209 terminals (mean 181).
Custom-written Python scripts were used to ensure that gene alignments were in frame and to concatenate them into a supermatrix. The concatenated dataset comprised 210 terminals and 165,504 characters, of which 63,814 were parsimony informative, 34,943 variable but uninformative, and 66,747 constant.
To explore the potential role of reticulate evolution in our data, we inferred locus heterzygosity and allele divergence for the samples of the fireweed complex using HybPhaser (Nauheimer et al. Citation2021).
Phylogenetic analysis
A phylogeny of the concatenated supermatrix was inferred with IQTREE 2.2.0.5 (Minh et al. Citation2020), under automatic partition- and model-testing (Lanfear et al. Citation2016), with codon positions as the starting partitions. The favoured model maintained the partition scheme with GTR + F + I + G4 as the substitution model in all partitions. UltraFast Bootstraps (UFB) were used to estimate branch support (Minh et al. Citation2013). The phylogeny was outgroup-rooted on the genus Abrotanella, which is often placed as sister to the remainder of Senecioneae (Zhang et al. Citation2021).
Results
Deeper relationships in the phylogeny were largely consistent with topologies previously inferred from nuclear ribosomal data (Supplementary Figure S1). The samples from the fireweed complex and South African Senecio leptophyllus DC. formed a clade with high support (UFB 100).
Within the complex, three main clades were resolved (): First, S. inaequidens from Europe, S. leptophyllus, and S. harveianus, with only weak support (UFB 61). Most internal relationships in this clade were also poorly supported, including the clade of S. inaequidens. Second, a very strongly supported clade (UFB 100) of South African S. skirrhodon and two New Zealand samples from the southeast of North Island.
Figure 2. Part of the phylogenetic tree of Senecioneae showing the fireweed complex. Three main clades are highlighted with boxes: Senecio inaequidens (brown), S. skirrhodon (blue), and S. madagascariensis (yellow). Silhouettes show representative leaf shapes of type specimens. Branch labels are UltraFast Bootstrap values. The complete phylogeny of tribe Senecioneae can be found in Supplementary Figure S1.
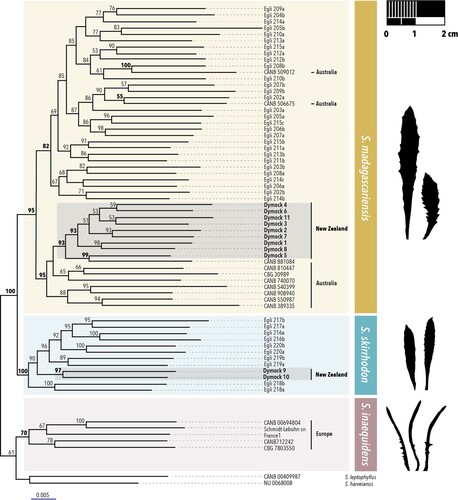
Third, a strongly supported (UFB 95), large clade of all South African and Australian specimens identified a priori as S. madagascariensis, several specimens of uncertain affiliation from South Africa, and nine New Zealand samples from Northland. Within S. madagascariensis, all Australian and New Zealand specimens except two formed a strongly supported (UFB 95) clade, and all South African specimens and two Australian specimens formed its sister clade, albeit with weak support (UFB 82).
Locus heterozygosity was 42–92% in Senecio inaequidens (median 84%), 23–86% in S. madagascariensis (median 70%), and 74–90% in S. skirrhodon (median 81%). Within-locus allele divergence was 1.0–3.6% in Senecio inaequidens (median 2.6%), 0.5–2.3% in S. madagascariensis (median 1.5%), and 1.9–2.7% in S. skirrhodon (median 2.3%).
To support our re-identification of specimens of doubtful affiliation, we compiled information from Hilliard’s descriptions and key and quantified some additional characters by examining photos of type specimens and specimens identified by Hilliard. summarises diagnostic characters of the five species that were historically and/or are currently considered introduced outside of southern Africa.
Table 1. Diagnostically useful morphological traits of the five fireweed species that have been considered or are currently considered to be invasive outside of southern Africa, based on keys and descriptions of Hilliard (Citation1977), where available, and examination of photos of type specimens or specimens identified by Hilliard.
Morphological re-examination of the South African specimens of originally uncertain identification in the S. madagascariensis clade against Hilliard’s specimens and descriptions made their placement in that clade plausible, as they showed typical branching patterns and leaf shapes. New Zealand specimens placed in S. skirrhodon showed leaf length to width ratios at the upper end of the range expected for this species ( L, N) and higher than other specimens of the species ( K, M), presumably because specimens of uncertain affiliation were preferentially collected for sequencing in this study. However, they also had calyculus bracts that were larger and in particular wider (c. 0.8–1.5 mm) than those placed in S. madagascariensis (c. 0.5–0.8 mm) ( A–D, G-J), in both cases matching the respective species’ type material (http://plants.jstor.org/stable/10.5555/al.ap.specimen.p0000355, http://plants.jstor.org/stable/10.5555/al.ap.specimen.mpu011695).
Figure 3. Comparison of calycular bracts and leaves of Senecio madagascariensis (A-F) and S. skirrhodon (G-N) in New Zealand. Calyculus images are all to same scale, as are leaf silhouettes. A, J.J. Dymock s.n. (CANB 953777), B, J.J. Dymock s.n. (CANB 953779), C, J.J. Dymock s.n. (CANB 9537780), D, J.J. Dymock s.n. (CANB 9537783), E, J.J. Dymock s.n. (CANB 953779), F, J.J. Dymock s.n. (CANB 953783), G, W. Symes s.n. (CHR 649039A), H, M. Gray 7108 (CANB 508525), I, J.J. Dymock s.n. (CANB 953781), J, J.J. Dymock s.n. (CANB 953782), K, W. Symes s.n. (CHR 645039B), L, J.J. Dymock s.n. (CANB 953782), M, W. Symes s.n. (CHR 645039A), N, J.J. Dymock s.n. (CANB 953781).
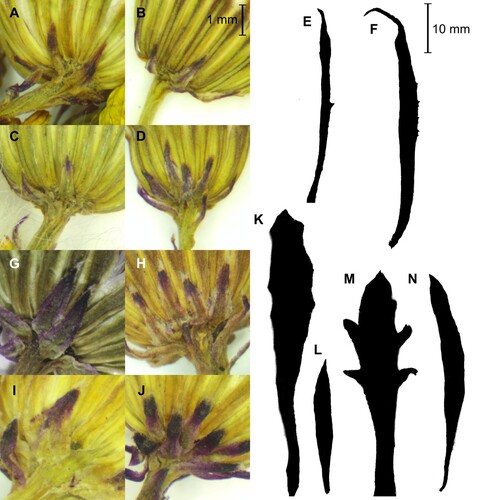
Field observations by one of the authors (J.J.D.) and collectors’ notes on herbarium specimens indicate that the two species tend to occupy different habitats in New Zealand. Senecio madagascariensis was found in grazed pasture, whereas S. skirrhodon occurs in beach habitats such as dunes but also variously on roadside gravel and lava tracks, along railway lines and streams, and on wasteland and building sites.
Discussion
Our data add to growing confidence that sequence capture data from hundreds of genes can provide phylogenetic or population genetic resolution even at the level of species complexes (Nicholls et al. Citation2015; Slimp et al. Citation2020; Schmidt-Lebuhn Citation2022). As results are based on larger numbers of sequence characters and gene regions, they can provide more confidence in species delimitation and relationships than historically used marker systems such as nuclear ribosomal spacer regions (Radford et al. Citation2000; Le Roux et al. Citation2006).
By sampling four of the six species of the fireweed complex, we resolved three main lineages. Two of them are individual species (S. madagascariensis, S. skirrhodon), and the third comprises three species (S. inaequidens, S. harveianus, S. leptophyllus). Most critically, New Zealand samples could be placed with very high confidence in two of these clades, Senecio madagascariensis and S. skirrhodon. The confirmation of the identity of S. madagascariensis in northern regions of New Zealand () supports recent field observations of the invasiveness of Senecio species in pasture habitats there. Senecio skirrhodon does not display this invasive behaviour in New Zealand.
Figure 4. Detailed map of the North Island of New Zealand showing geocoded specimens of Senecio madagascariensis (yellow) and S. skirrhodon (blue) from the Australasian Virtual Herbarium (accessed October 2022). One specimen with coordinates in the ocean has been removed.
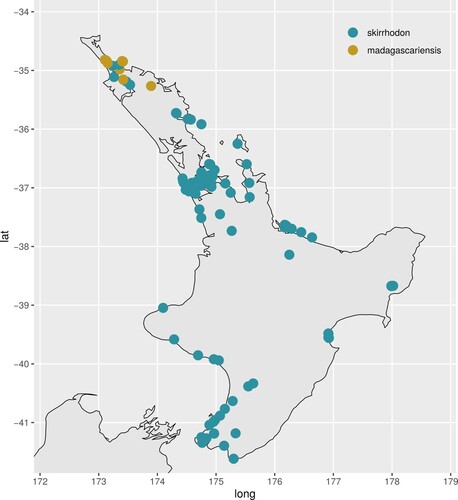
Within S. madagascariensis, two subclades were observed, albeit with one of them poorly supported. All New Zealand samples of the species and most Australian samples formed one clade, and South African samples and two Australian samples formed the second. This suggests three possible conclusions. First, the species may have been introduced to Australia at least twice, as suggested previously (Dormontt et al. Citation2014). Second, it is possible that New Zealand populations are derived from Australia, given that they are nested inside the latter. However, with our current geographic coverage, this conclusion must remain tentative.
This is because of the third conclusion, i.e. that KwaZulu-Natal, to which our South African sampling was limited, may not represent the area of origin of most introduced Australasian populations. Historically, that area has been considered the most likely area of origin of introduced S. madagascariensis based on isozyme data and ribosomal sequence similarity (Scott et al. Citation1998; Radford et al. Citation2000; Le Roux et al. Citation2006). However, the sister group relationship between samples from KwaZulu-Natal and nearly all introduced samples does not support this assumption, because we would in that case expect the latter to be phylogenetically nested inside the former. Further southern African sampling is needed to test the second and third conclusions and hence resolve the origins of New Zealand populations.
Although not the focus of our study, we included several European S. inaequidens in our sampling, and found them differentiated from S. harveianus and S. leptophyllus with only weak support. We did not sample South African S. inaequidens, so that no definite conclusions about their relationships can be drawn. However, S. inaequidens and S. harveianus are very similar in habit and leaf morphology and are primarily distinguished by their calycular bracts, which are few and short in S. inaequidens as compared to forming several ranks and being up to half as long as the involucral bracts in S. harveianus (Hilliard Citation1977).
When examining European specimens, we found that their calycular bracts match Hilliard’s description and the type of S. harveianus (http://plants.jstor.org/stable/10.5555/al.ap.specimen.e00239820), and indeed, although the European populations are now consistently classified as S. inaequidens (Lafuma et al. Citation2003; Lachmuth et al. Citation2010), they were historically treated as S. harveianus in some countries (Rzedowski et al. Citation2003). To verify the identity of European fireweed, increased sampling of these two species will be a priority in the future expansion of our dataset.
A caveat of our phylogenetic analysis is that it assumed that sequence variants divergent enough to be flagged as putative paralogs are indeed the result of gene duplication as opposed to reticulate evolution. The fireweed complex shows variation in ploidy level (López et al. Citation2008), and it has been suggested that crosses between sequence variants followed by polyploidisation have played a role in the origin of European Senecio inaequidens (Lafuma et al. Citation2003).
However, the three species of the complex sampled in this study showed similar patterns of sequence divergence. In all cases, locus heterozygosity was in line with values expected from ‘pure’ species (<90%) as opposed to allopolyploids (Lars Nauheimer, pers. comm.). On the other hand, within-locus allele divergence was considerably higher than the expected value of less than 1%. Taken together, these data suggest that the fireweed complex as a whole may be of hybridogenic origin. Broader and deeper sampling of South African populations and of close relatives of the complex will be required to resolve its evolutionary history in detail.
Supplementary figure S1 Full phylogeny
Download PDF (60.5 KB)Appendix 1 Voucher information and SRA accession numbers
Download MS Word (6.3 KB)Acknowledgements
We are grateful to Vincent Lesieur (CSIRO Health & Biosecurity) for providing a sample of Senecio inaequidens, to Ines Schönberger and Ilse Breitwieser (Allan Herbarium) for images of S. skirrhodon, to Lars Nauheimer for advice on within-locus sequence divergence, to Brendan Lepschi for comments on an early version of the manuscript, and to Rob Smissen and Ewen Cameron for refereeing the manuscript. We made use of the sequencing services of the Biomolecular Resource Facility of the Australian National University and of type specimen images on JSTOR Global Plants.
Data availability
Raw sequence reads generated for the study are available in the Sequence Read Archive under accession numbers SAMN30608566–SAMN30608673. The dataset includes samples from the Genomics for Australian Plants project that are available in the Bioplatforms Data Portal (https://data.bioplatforms.com) under sample numbers 80011, 80025, 80029, 80033, 80077, 80085, 80113, 80268, 80269, and 80271 and samples from the Plant And Fungal Tree Of Life project that are available at the International Nucleotide Sequence Database Collaboration under sequence IDs ERR3089139, ERR5033756, ERR6041605, ERR6041607, ERR6041608, ERR6041609, ERR6041610, ERR6041627, ERR6041634, ERR6041641, ERR6041649, ERR6041653, ERR6041654, ERR6041659, ERR6041660, ERR6041664, ERR6041665, ERR6041666, ERR6041667, ERR6041668, ERR6041669, ERR6041670, ERR6041671, ERR6041672, ERR6041673, ERR6041674, ERR6041675, ERR6041676, ERR6041677, ERR6041678, ERR6041679, ERR6041680, ERR6041681, ERR6041682, ERR6041683, ERR6041684, ERR7599340, ERR7599351, ERR7599353, ERR7599355, ERR7599386, ERR7618437, ERR7618438, ERR7618439, ERR7618440, ERR7618441, ERR7618442, ERR7618443, ERR7618444, ERR7618445, ERR7619528, ERR7619529, ERR7619530, ERR7619531, ERR7621148, ERR7621209, ERR7621230, ERR7621231, ERR7621242, ERR7621243, ERR7621246, ERR7621254, ERR7621263, ERR7621271, ERR7621273, ERR7621289, ERR7621293, ERR7621298, ERR7621309, ERR7621316, ERR7621319, ERR7621327, ERR7621332, ERR7621341, ERR7621345, ERR7621350, ERR7621647, ERR7621648, ERR7621691, ERR7621692, ERR7621694, ERR7621695, ERR7621698, ERR7621700, ERR7621701, ERR7621702, ERR7621703, ERR7621704, ERR7621905, SRR3995486, SRR9110571, and SRR9113366. Target file for assembly, data matrix, and phylogenetic tree are available in the CSIRO Data Access Portal at DOI:10.25919/4qbc-pb93.
Disclosure statement
No potential conflict of interest was reported by the author(s).
Additional information
Funding
References
- Bolger AM, Lohse M, Usadel B. 2014. Trimmomatic: a flexible trimmer for illumina sequence data. Bioinformatics. 30(15):2114–2120. doi:10.1093/bioinformatics/btu170.
- Cabrera AL, Ré RR. 1965. Sobre un Senecio adventicio en la Provincia de Buenos Aires. Revta Facultad de Agronomía de la Universidad Nacional de La Plata. 41:43–50.
- Dormontt EE, Gardner MG, Breed MF, Rodger JG, Prentis PJ, Lowe AJ. 2014. Genetic bottlenecks in time and space: reconstructing invasions from contemporary and historical collections. PLOS ONE. 9(9):e106874. doi:10.1371/journal.pone.0106874.
- Hilliard OM. 1977. Compositae in Natal. Pietermaritzburg: University of Natal Press.
- Jackson C, McLay T, Schmidt-Lebuhn AN. 2021. hybpiper-rbgv and yang-and-smith-rbgv: Containerization and additional options for assembly and paralog detection in target enrichment data. bioRxiv [Internet]. [accessed 2021 Nov 11]. doi:10.1101/2021.11.08.467817.
- Johnson MG, Gardner EM, Liu Y, Medina R, Goffinet B, Shaw AJ, Zerega NJC, Wickett NJ. 2016. HybPiper: extracting coding sequence and introns for phylogenetics from high-throughput sequencing reads using target enrichment. Applications in Plant Sciences. 4(7):1600016. doi:10.3732/apps.1600016.
- Johnson MG, Pokorny L, Dodsworth S, Botigué LR, Cowan RS, Devault A, Eiserhardt WL, Epitawalage N, Forest F, Kim JT, et al. 2019. A universal probe set for targeted sequencing of 353 nuclear genes from any flowering plant designed using k-medoids clustering. Systematic Biology. 68(4):594–606. doi:10.1093/sysbio/syy086.
- Jovet P, de Vilmorin R, Kerguelen M. 1975. Troisieme supplement de la Flore descriptive et illustree de la France par l’abbe H. Coste. [place unknown]: Albert Blanchard.
- Koenen EJM, Kidner C, de Souza ÉR, Simon MF, Iganci JR, Nicholls JA, Brown GK, de Queiroz LP, Luckow M, Lewis GP, et al. 2020. Hybrid capture of 964 nuclear genes resolves evolutionary relationships in the mimosoid legumes and reveals the polytomous origins of a large pantropical radiation. American Journal of Botany. 107(12):1710–1735. doi:10.1002/ajb2.1568.
- Lachmuth S, Durka W, Schurr FM. 2010. The making of a rapid plant invader: genetic diversity and differentiation in the native and invaded range of Senecio inaequidens. Molecular Ecology. 19(18):3952–3967. doi:10.1111/j.1365-294X.2010.04797.x.
- Lafuma L, Balkwill K, Imbert E, Verlaque R, Maurice S. 2003. Ploidy level and origin of the European invasive weed Senecio inaequidens (Asteraceae). Plant Systematics and Evolution. 243(1):59–72. doi:10.1007/s00606-003-0075-0.
- Lanfear R, Frandsen PB, Wright AM, Senfeld T, Calcott B. 2016. Partitionfinder 2: new methods for selecting partitioned models of evolution for molecular and morphological phylogenetic analyses. Molecular Biology and Evolution. 34(3):msw260–msw773. doi:10.1093/molbev/msw260.
- Le Roux JJ, Wieczorek AM, Ramadan MM, Tran CT. 2006. Resolving the native provenance of invasive fireweed (Senecio madagascariensis Poir.) in the Hawaiian Islands as inferred from phylogenetic analysis. Diversity Distributions. 12(6):694–702. doi:10.1111/j.1472-4642.2006.00271.x.
- López MG, Wulff AF, Poggio L, Xifreda CC. 2008. South African fireweed Senecio madagascariensis (Asteraceae) in Argentina: relevance of chromosome studies to its systematics. Botanical Journal of the Linnean Society. 158(4):613–620. doi:10.1111/j.1095-8339.2008.00865.x.
- Marohasy J. 1993. Are we justified in considering fireweed (Senecio madagascariensis) an exotic? Proceedings II of the 10th Australian Weeds Conference and 14th Asian Pacific Weed Science Society Conference, Brisbane, Australia, 6-10 September, 1993, 122–127.
- McFadyen R, Morin L. 2012. Senecio madagascariensis Poir.–fireweed. In: Julien M, McFayden R, Cullen JM, editor. Biological control of weeds in Australia. Clayton: CSIRO Publishing; p. 526–536.
- McLay TGB, Birch JL, Gunn BF, Ning W, Tate JA, Nauheimer L, Joyce EM, Simpson L, Schmidt-Lebuhn AN, Baker WJ, et al. 2021. New targets acquired: improving locus recovery from the Angiosperms353 probe set. Applications in Plant Sciences. 9:e11420. doi:10.1002/aps3.11420.
- Michael PW. 1981. Alien plants. In: Groves RH, editor. Australian vegetation. Cambridge: Cambridge University Press; p. 44–64.
- Minh BQ, Nguyen MAT, von Haeseler A. 2013. Ultrafast approximation for phylogenetic bootstrap. Molecular Biology and Evolution. 30(5):1188–1195. doi:10.1093/molbev/mst024.
- Minh BQ, Schmidt HA, Chernomor O, Schrempf D, Woodhams MD, von Haeseler A, Lanfear R. 2020. IQ-TREE 2: new models and efficient methods for phylogenetic inference in the genomic era. Molecular Biology and Evolution. 37(5):1530–1534. doi:10.1093/molbev/msaa015.
- Nauheimer L, Weigner N, Joyce E, Crayn D, Clarke C, Nargar K. 2021. HybPhaser: a workflow for the detection and phasing of hybrids in target capture data sets. Applications in Plant Sciences. 9(7):e11441. doi:10.1002/aps3.11441.
- Nicholls J, Pennington R, Koenen E, Hughes C, Hearn J, Bunnefeld L, Dexter K, Stone G, Kidner C. 2015. Using targeted enrichment of nuclear genes to increase phylogenetic resolution in the neotropical rain forest genus Inga (Leguminosae: Mimosoideae). Frontiers in Plant Science. [Internet]. [accessed 2022 Sep 2] 6. https://www.frontiersin.org/articles/10.3389fpls.2015.00710.
- Radford IJ, Muller P, Fiffer S, Michael PW. 2000. Genetic relationships between Australian fireweed and South African and Madagascan populations of Senecio madagascariensis Poir. and closely related Senecio species. Australian Systematic Botany. 13(3):409–423. doi:10.1071/SB98029.
- Rzedowski J, Vibrans H, Calderon de Rzedowski G. 2003. Senecio inaequidens (Compositae, Senecioneae), una maleza perjudicial introducida en Mexico. Acta Botanica Mexicana. 63:83–96.
- Schmidt-Lebuhn AN. 2022. Sequence capture data support the taxonomy of Pogonolepis (Asteraceae: Gnaphalieae) and show unexpected genetic structure. Australian Systematic Botany. 35(4):317–325. doi:10.1071/SB22010.
- Schmidt-Lebuhn AN, Bovill J. 2021. Phylogenomic data reveal four major clades of Australian Gnaphalieae (Asteraceae). TAXON. 70(5):1020–1034. doi:10.1002/tax.12510.
- Schönberger I, Wilton AD, Boardman KF, Breitwieser I, de Lange PJ, de Pauw B, Ford KA, Gibbs ES, Glenny DS, Heenan PB, et al. 2020. Checklist of the New Zealand flora - seed plants [Internet]. [accessed 2022 Oct 23]. doi:10.26065/sw4r-0w62.
- Scott LJ, Congdon BC, Playford J. 1998. Molecular evidence that fireweed (Senecio madagascariensis, Asteraceae) is of South African origin. Plant Systematics and Evolution. 213(3):251–257. doi:10.1007/BF00985204.
- Slimp M, Williams LD, Hale H, Johnson MG. 2020. On the potential of Angiosperms353 for population genomics. bioRxiv.:2020.10.11.335174. doi:10.1101/2020.10.11.335174.
- Tsutsumi M. 2011. Current and potential distribution of Senecio madagascariensis Poir. (fireweed), an invasive alien plant in Japan. Grassland Science. 57(3):150–157. doi:10.1111/j.1744-697X.2011.00222.x.
- Tutin TG, Heywood VH, Burges NA, Moore DM, Valentine DH, Walters SM, Webb DA. 1976. Flora europaea. New York: Cambridge University Press.
- Webb CJ, Sykes WR, Garnock-Jones PJ. 1988. Flora of New Zealand. Christchurch: DSIR.
- Wijayabandara K, Campbell S, Vitelli J, Shabbir A, Adkins S. 2022. Review of the biology, distribution, and management of the invasive fireweed (Senecio madagascariensis Poir). Plants. 11(1):107. doi:10.3390/plants11010107.
- Yang Y, Smith SA. 2014. Orthology inference in nonmodel organisms using transcriptomes and low-coverage genomes: improving accuracy and matrix occupancy for phylogenomics. Molecular Biology and Evolution. 31(11):3081–3092. doi:10.1093/molbev/msu245.
- Zhang C, Huang C-H, Liu M, Hu Y, Panero JL, Luebert F, Gao T, Ma H. 2021. Phylotranscriptomic insights into Asteraceae diversity, polyploidy, and morphological innovation. Journal of Integrative Plant Biology. 63(7):1273–1293. doi:10.1111/jipb.13078.