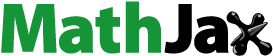
Abstract
The adenosine analogue GS-441524 has demonstrated efficacy in treatment of feline infectious peritonitis (FIP). With no commercially registered formulations of GS-441524 available, global focus shifted to its pro-drug remdesivir, as it became more accessible throughout the COVID-19 pandemic. This study developed and validated a simple liquid chromatography equipped with a fluorescence detector to quantify plasma concentrations of GS-441524 applicable for routine therapeutic monitoring of remdesivir or GS-441524 therapy for FIP infected cats. A Waters X-Bridge C18, 5 µm, 150 × 4.6 mm, column was used and mixtures of 20 mM ammonium acetate (pH 4.5) with acetonitrile of 5% and 70% were prepared for gradient mobile phase. With a simple protein precipitation using methanol to clean plasma sample, GS-441524 was monitored at excitation and emission wavelengths of 250 nm and 475 nm, respectively. Using an external standard, the lowest and highest limits of quantification were 19.5 ng/mL to 10,000 ng/mL, respectively. The intra- and inter day trueness of the quality controls (QCs) were within 10% of their nominal concentrations and intra- and inter day precision of the QCs (expressed as the coefficient of variation) ranged from 1.7 to 5.7%, This assay was able to quantify plasma trough levels of GS-441524 (23.7–190.1 ng/mL) after the administration of remdesivir (9.9–15.0 mg/kg BW, IV or SC) in FIP cats (n = 12). Accordingly, this study generated an alternative and cost-effective way to quantify GS-441524 in feline biological fluids at least up to 24 hr after administrations of remdesivir.
1. Introduction
Remdesivir (GS-5743) is a broad-spectrum antiviral nucleotide pro-drug which has potent in-vitro activity against RNA viruses including Ebola, MERS-CoV, SARS-CoV-1, and SARS-CoV-2 (Badgujar et al. Citation2020). It is one of few approved drugs, including ritonavir, bebtelovimab, and molnupiravir, for treating SARS-CoV-2 (COVID-19 Treatment Guidelines Panel Citation2019; Jorgensen et al. Citation2020) and has been reported to reduce the time of recovery from SARS-CoV-2 infection (Beigel et al. Citation2020). Remdesivir is proposed to inhibit viral replication intracellularly, by interfering with viral RNA-dependent RNA polymerase activity in the form of an active triphosphate (GS-443902) (Saha et al. Citation2020). Conversion from remdesivir into the active triphosphate requires multiple steps of hydrolysis (Kokic et al. Citation2021) ().
Figure 1. Biosynthesis of remdesivir in human (individual image sourced from PubChem. CID 121304016 (GS-5734), CID 121313150 (GS-704277), CID 44468216 (GS-441524), and CID 56832906 (GS-443902).

Existing pharmacokinetic knowledge in species including humans, mice and rhesus monkeys suggested remdesivir would undergo rapid hydrolysis in vivo, with a very short elimination half-life (t½) of 1–1.5 hr in human plasma (Warren et al. Citation2016; Li et al. Citation2022). Among several hydrolysed products, GS-441524, the parent nucleoside, offered more promise as a potential marker for therapeutic monitoring during remdesivir therapy, owing to its long t½ of 24 hr in human plasma (Deb et al. Citation2021).
Feline infectious peritonitis (FIP) is an immune-mediated disease caused by a virulent biotype of enteric feline corona virus (FCoV) (O’Reilly et al. Citation1979). Historically, FIP was associated with a near 100% mortality rate and no effective treatments (Pedersen Citation2009). An in-vivo study with experimentally induced FIP in cats, demonstrated daily subcutaneous injections of GS-441524 (5 mg/kg BW) were sufficient to provide an effective plasma concentration (AUC0-24hr 38.9 µmol/L*h) for 24 hr to inhibit viral replication (Murphy et al. Citation2018). A subsequent study demonstrated that GS-441524, when administered subcutaneously at a dosage of 4 mg/kg BW daily, produced long-term surviving cats with naturally occurring FIP (Pedersen et al. Citation2019). Despite potential therapeutic efficacy of GS-441524 to treat FIP, there are no registered veterinary formulations available. In 2020, Australian veterinarians gained legal access to compounded parenteral remdesivir and more recently, to oral GS-441524. Although remdesivir’s conversion to GS-441524 has been documented in other species, no information is available on the bioavailability of GS-441524 following remdesivir administration in cats.
Our research team is investigating the clinical efficacy of remdesivir for the treatment of naturally occurring FIP in client owned cats. This includes longitudinal monitoring of the GS-441524 plasma concentrations achieved in cats receiving treatment, to better elucidate optimal therapeutic levels required to achieve remission states. Here we report a novel analytical method, using high-performance liquid chromatography with fluorescence detection (HPLC-FLD), to quantify feline plasma concentrations with GS-441524 in cats with confirmed naturally occurring FIP. To our knowledge, currently available analytical methods to quantify GS-441524 in biological fluids are limited to LC-MS/MS methods (Avataneo et al. Citation2020; Alvarez et al. Citation2020; Habler et al. Citation2021; Du et al. Citation2021) requiring expensive systems that are not widely available to general laboratories. Therefore, the aim of this study was to develop and validate an alternative and cost-effective way to quantify GS-441524 in feline biological fluids applicable for routine therapeutic monitoring studies.
2. Methods
2.1. Chemicals
GS-441524 (purity 99.77%, CAS: 1191237-69-0), remdesivir (GS-5734, CAS 1809249-37-3), alanine (Ala) metabolite (GS-704277; CAS 1911579-04-8), and GS-441524 triphosphate (GS-443902; CAS 2725956-16-9) were purchased from Assay Matrix (Ivanhoe North, VIC, Australia), and ammonium acetate and glacial acetic acid were purchased from Sigma Aldrich (Castle Hill, NSW, Australia). HPLC grade acetonitrile (MeCN) and methanol (MeOH) were purchased from Thermo Fisher Scientific (Macquarie Park, NSW, Australia). Water was purified via Milli-Q water purification system (Merck Millipore, Burlington, MA, USA).
2.2. Working solutions, calibration standards, and quality controls
GS-441524 was prepared as a 0.5 mg/mL stock solution in 50% MeCN. Response of the instrumentation reading of the GS-441524 peak area of this stock solution was measured in triplicate by liquid chromatography (LC), and the mean peak area was used as the reference value. The stock solution was stored at −20 °C and prior to its use for preparing fresh working solutions, the GS-441524 peak area of the stock solution was remeasured by LC and checked with the reference value. If the stock solution peak area was > 5% difference than that of the reference value, then the stock solution was freshly reprepared. Working solutions of standards, ranging from 0.488 to 250 µg/mL, were freshly prepared by serially diluting the stock solution with MeOH. For preparation of the final plasma calibration standards, a volume of 2 µL of each of the working solutions was added to 48 µL of blank pooled feline plasma (total 50 µL) to give 10 calibration standards ranging from 19.5 to 10,000 ng/mL. Three concentrations of GS-441524 which served as quality controls (QCs) (35.2, 563, and 9,000 ng/mL) were also prepared in blank plasma and stored at −20 °C.
2.3. Sample preparation
Feline plasma samples (50 µL) underwent protein precipitation (PP) with the addition of 100 µL of MeOH after testing several other solutions (MeCN, MeOH, or mixture of MeCN/MeOH) with or without presence of acids (5% phosphoric acid, 5% acetic acid, or 10% trichloroacetic acid (TCA). The prepared sample was then vortexed, centrifuged at 14,000 × g for 10 min, the supernatant was transferred to the injection vial, and 10 µL was injected into the HPLC system. Between each sample injected into the HPLC system, the injection needle was rinsed with 40% MeOH.
2.4. Chromatographic condition
For detection and analysis of GS441524, a Shimadzu Nexera XR LC system (Rydalmere, NSW, Australia) was used. For the mobile phase, a mixture of 20 mM of ammonium acetate (adjusted to pH 4.5 with acetic acid) with solutions of either 5% of acetonitrile (A) or 70% of acetonitrile (B) prepared for following gradient: 0–1 min (0–5% B), 1–2 min (5% B), 2–5 min (5–50% B), 5–10 min (50–90% B), 10–11 min (90% B), and 11–12 min (90–0%) at a flow rate of 1.2 mL/min. A total run time was 15 min in order to equilibrate the condition. After testing several columns (Synergi 4 µm, 150 × 4.6 mm, Hypersil ODS 5 µm, 4.6 mm × 150 mm, and Discovery® C18, 5 µm, 4.6 mm × 250 mm), the Waters X-Bridge C18, 5 µm, 150 × 4.6 mm (Dundas, NSW, Australia) was selected as the stationary phase with the column temperature maintained at 35 °C. A fluorescence detector (RF-20A xs) (Shimadzu, Rydalmere, NSW, Australia) was used to monitor GS-441524 with excitation and emission wavelengths of 250 nm and 475 nm, respectively.
2.5. Validation of the method
Validation was performed on following parameters which in accordance with the biological method validation guidelines [International Council for Harmonisation of Technical Requirements for Pharmaceuticals for Human Use (ICH), 2022].
2.5.1. Selectivity
To ensure detection of GS-441524 was well differentiated from endogenous component(s) in the feline plasma matrix, different sources of individual plasma [including blank plasma from cats with FIP with or without administered other medications (n > 20) and drug-free plasma from clinically normal cats (n > 5)] were tested. During an initial optimising stage, the packed red blood cell fraction after separation from blank plasma was also analysed to assess interference from haemolysed red blood cells. Retention times of remdesivir and its other metabolites [Alanine metabolite (GS-704277) and GS-441524 triphosphate (GS-443902)]; as well as medications commonly used in feline practice for initial supportive care or sedation [such as dexamethasone (Dexasone®), maropitant (Cerenia®), medetomidine (Domitor®), midazolam (Hypnovel®), ondansetron (Ondaz®Zydis®), mirtazapine (AxitTM), amoxicillin-clavulanate (Amoxyclav 50TM) and gabapentin (Neurontin®)] were tested. To qualify that the analytical condition for GS-441524 was acceptable, the minimum interference was required to be < 20% of upper limit (ICH, 2022).
2.5.2. Calibration curves
Quantitation of GS-441524 was achieved by generating best fit regression line using peak areas of GS-441524 (external standard). Best fit regression was selected after equating to linear regression and applying different weighting factors (1/x and 1/x2). To qualify as a calibration curve, at least 75% of back calculated concentrations of calibrators are required to be ± 15% of the variation from the nominal concentrations [except for the lowest measurable concentration (a.k.a. lowest limit of quantification: LLOQ) which is ± 20%] (ICH, 2022).
2.5.3. Sensitivity
While LLOQ represents the lowest sensitivity, this was estimated using calibration curves with below equation, and bias and precision of LLOQ were tested for its suitability (ICH, 2022).
where σ is the standard deviation of y-intercepts and S is the mean of the calibration curve slopes (n = 3). The concentration of the LLOQ is required to have trueness range within 20% (80–120%) of the nominal concentration, the precision of samples is required to have a coefficient variation (CV) of ≤ 20%, and to have at least 10 times greater signal-noise level than a blank sample (ICH, 2022). Accordingly, 5 additional LLOQ samples, prepared independently from calibrators, were used to test for trueness and precision.
2.5.4. Trueness & precision
The intra- and inter day trueness and precision were analysed with triplicate QC samples (35.2, 563, and 9,000 ng/mL) assay within a day and on three consecutive days, respectively.
Trueness, expressed as a bias was calculated by [(estimated value - nominal value)/nominal value × 100]. For precision, relative difference of the observed concentrations, expressed as coefficient variation (CV %) was calculated by average of standard deviation/average estimated value × 100. For acceptance criteria for QCs, trueness within 15% and precision with 15% of QCs were required (ICH, 2022).
2.5.5. Analyte recovery
The efficiency of drug extraction was tested using an absolute recovery which compared variations of peak area between pre-spiked GS-441524 into plasma samples vs. GS-441524 in buffer solution (10,000 ng/mL, n = 9)
Additionally, recovery of pre-spiked samples was also investigated with post-spiked plasma samples (10,000 ng/mL, n = 2) and with pre-spiked buffer (n = 3).
2.5.6. Carry over
Carry over which tests the risk of potential interference from the previous analysis, was tested with injection of different blank plasma samples straight after running upper limit sample. It is required that the peak area in blank plasma should not exceed 20% of the peak area of LLOQ (ICH, 2022).
2.5.7. Dilution integrity
Dilution integrity was tested with plasma sample spiked with GS-441524 to make 13,500 ng/mL (n = 2) and diluted with blank plasma in 1:2 (v/v) ratio. To qualify the dilution integrity test, measured concentrations should be < 15% of variation to the nominal concentrations (ICH, 2022).
2.5.8. Stability
Processed QCs were left at room temperature (25 °C) for 12 hr and in an autosampler (4 °C) for 24 hr to check benchtop stability and autosampler stability, respectively using low and high QCs (35.2 and 9,000 ng/mL) in triplicates. Injection stability was done by repeated injection of processed samples. Three cycle and thaw stability tests for both were done over a 5-day period. Unprocessed QCs were stored for 1 month at −20 °C before processing to explore longer term stability.
2.6. Clinical application
Clinical samples were utilised from 12 cats with FIP, receiving remdesivir treatment as part of a broader clinical trial approved by The University of Sydney Animal Ethics Committee (2021/1874). For one cat, serial samples were collected following an initial dose of 15 mg/kg BW IV. For the remaining 11 cats, as single sample was used, taken 24 hrs following initial treatment with remdesivir at 9.87–15.0 mg/kg BW, IV or SC. GS-441524 plasma concentrations were quantified according to the above-described method to check its applicability.
2.7. Statistical analyses
Student’s t-tests and the Tukey-Kramer post hoc test, for multiple comparison, were applied using Graph Pad Prism version 9 for Windows (Graph Pad Software, USA). p-values < 0.05 were considered statistically significant.
3. Results
During initial screening, GS-441524 was observed to have an intrinsic fluorescent property (max λex: 241 nm: max λem: 405 nm) () that had a greater sensitivity than when detected with UV. However, within this fluorescence range, the plasma interfered with detecting GS-441524 in some of the feline plasma samples, that could not be corrected by modifying mobile phases or using other columns (). Consequently, the optimised fluorescence range was set to λex: 250 nm; λem: 475 nm to remove the plasma interference.
Figure 2. Chromatograms depicting fluorescence excitation (left: max λex: 241 nm) and emission spectrum (right: max λem 405 nm) of GS-441524 (100 ng/mL); y axis is absorbance; x axis is wavelength in nm.

Figure 3. Chromatograms of blank plasma monitored at λex: 245 nm/λem: 395 nm (red trace) and at λex: 250 nm/λem: 475 nm (black trace). Blank plasmas pre-spiked with mixtures of GS-441524 & remdesivir [78.1 (lower blue trace) and 313 ng/mL (higher blue trace), respectively] monitored at λex: 250 nm/λem: 475 nm.
![Figure 3. Chromatograms of blank plasma monitored at λex: 245 nm/λem: 395 nm (red trace) and at λex: 250 nm/λem: 475 nm (black trace). Blank plasmas pre-spiked with mixtures of GS-441524 & remdesivir [78.1 (lower blue trace) and 313 ng/mL (higher blue trace), respectively] monitored at λex: 250 nm/λem: 475 nm.](/cms/asset/cf7f2d84-e2a6-4a54-8937-9632973fce2b/tveq_a_2246553_f0003_c.jpg)
3.1. Validation
3.1.1. Selectivity
Chromatograms of blank plasmas pre-spiked with GS-441524, remdesivir, GS-443902, a mixture of GS-441524, remdesivir, the alanine metabolite, and a combination of therapeutic drugs (listed in method section) are shown in . The retention time (Rt) of GS-441524 was 4.78 min, well separated from remdesivir (Rt: 9.95 min), GS-443902 (Rt: 1.98 min) and alanine metabolite (Rt: 2.21 min). None of the tested formulations interfered with Rt of GS-441524 other than unknown peaks consistently observed at Rt 6.73 min and 7.21 min for tablet form of drugs which likely due to formulation excipient(s).
Figure 4. Typical chromatograms of blank plasma (black trace); blank plasma pre-spiked with a mixture of GS-441524, remdesivir, and GS-443902 (blue trace), a mixture of GS-441524, remdesivir, and alanine metabolite (red trace), and a mixture of medications often used in FIP patients for initial supportive care or sedation (see methods) (brown trace). No peaks are interfered with retention time (RT) of GS-441524 (RT: 4.78 min). Rt of remdesivir: 9.95 min; Rt: Alanine metabolite: 2.21 min; GS-443902 (1.98 min).

3.1.2. Calibration curve and LOQ
The representative average regression calibration curves (n = 3), with a weighting factor of 1/x), were y = 238.1 (± 2.4 SD) x + 1112.5 (± 248.8 SD) [coefficient of determination (r2) > 0.999] was selected as a best fit regression. The trueness of the calibration curves, which estimated through back calculation, were varied from −5.2 to 9.1% (excluding LLOQ) of the nominal concentration and the precision levels of prepared fortified calibrators were between 1.2–7.6% (CV). Based on the average slope and SD of y-intercept, theoretical LLOQ was 10.5 ng/mL. However, this study set the LLOQ as 19.5 ng/mL (equivalent to on-column amount of 0.20 pg) to ensure trueness and precision within acceptable criteria. Accordingly, estimated trueness and precision of LLOQ (n = 5) were within 17.4% of variation and CV of 4.7%, respectively.
3.1.3. Precision and trueness
The intra- and inter day trueness and precision results of the QCs (35.2, 563, and 9,000 ng/mL) are summarised in . Intra- and inter day trueness were within 10% of variation (ranged from −8.9 to 8.3%) and intra- and inter day precisions of QCs (expressed as CV) ranged from 1.7 to 5.7%, respectively.
Table 1. Intra- and inter day precision and trueness of QCs (GS-441524).
3.1.4. Carry over, dilution integrity, and recovery
No significant carry over was observed when blank plasma samples were analysed after the analysis of the upper limit concentration. The dilution integrity test with duplicated plasma samples (13,500 ng/mL), further diluted with blank plasma in 1: 2 (v/v) ratio, was within 1.28% (4,531.7 ± 36.3 ng/mL) of the nominal concentration. Average (±SD) of absolute recovery was 97.5 ± 3.3% (n = 9); whereas recoveries estimated using pre-spiked plasma vs. post-spiked plasma and pre-spiked plasma vs. pr-spiked buffer were 95.6 ± 8.9% (n = 2) and 94.5 ± 8.2% (n = 3), respectively.
3.1.5. Stability
Stability was studied with both low (35.2 ng/mL) and high QC concentrations (9,000 ng/mL). Injection repeatability (n = 3 times) of low and high QCs, expressed as CV, were 0.4% and 0.3%, respectively. Stabilities of QCs samples (n = 3, each QC) stored for 24 h in the autosampler (4 °C) and on the benchtop (room temperature) were within 1.6% and 7.0% of the initial concentrations, respectively. Three cycle freeze and thaw test were carried over the period of 5 days (). Compared with initial values, no significant differences (p-values > 0.16) were observed with low and high QCs.
Table 2. Three cycle freeze and thaw stability (GS-441524) (n = 3).
3.2. Clinical application
Chromatograms of clinical sample (t = 0 to 24 hr, n = 1) and plasma concentrations (expressed as a semi-logarithmic scale) vs. times (either up to 24 hr (n = 1) or trough levels (n = 11) are presented in and , respectively. Quantified plasma GS-441524 concentrations in the feline patients ranged from 23.7 ng/mL to 3,755.7 ng/mL which well fitted into the calibration range.
Figure 5. Chromatograms of plasma GS-441524 after single IV injection of remdesivir (15 mg/kg BW); t = 0 hr (black trace), 1 hr (pink trace), 2 hr (blue trace), 8 hr (brown trace), 20 hr (green trace), and 24 hr (dark blue trace).

Figure 6. * Semi-logarithmic plasma GS-441524 concentrations vs. time curve in a cat with FIP following IV injection of remdesivir 15 mg/kg BW (red curve; n = 1); trough plasma GS-441524 concentrations after the administrations of remdesivir (9.87–15 mg/kg BW, IV or SC) in cats with FIP (n = 11); trough GS-441524 range from 23.7–190.1 ng/mL (84.9 ± 61.9 ng/mL, avg ± SD).

4. Discussion
While currently available analytical methods to quantify plasma levels of GS-441524 are based on the use of expensive mass spectrometry (); this study described an alternative and cost-effective method (HPLC-FLD) to quantify plasma GS-441524 concentrations applicable for routine drug monitoring during remdesivir treatment in cats with FIP. Compared to ultraviolet (UV) detection, fluorescence detection is considered more sensitive and specific as it uses excitation and emission wavelengths to monitor fluoresce of the analyte (Lipka and Vaccher Citation2015) especially in bio-samples that have numerous endogenous compounds, such as blood and plasma. Although other studies reported HPLC-FLD (λex: 245 nm; λem: 390 nm) to quantify remdesivir in pharmaceutical formulations and in human plasma (Hamdy et al. Citation2021; Moneim et al. Citation2021), to the best of our knowledge, there are no studies that report on quantification of GS-441524 in plasma using HPLC-FLD.
Table 3. Analytical methods to quantify GS-441524 in biological fluid (plasma).
This study identified GS-441524 was the major circulating plasma metabolite in cats with FIP, following remdesivir administration () which was similar to that reported in humans (Deb et al. Citation2021). It is interesting that the plasma half-life of remdesivir in humans is 1–1.30 hrs (Deb et al. Citation2021), but our other preliminary studies (not reported here) show that remdesivir is rapidly degraded in cat plasma to GS-441524 within minutes (Coggins et al. Citation2022). Therefore, GS-441524 is an essential biomarker for monitoring both remdesivir and GS-441524 treatment in FIP cats.
It has been determined that GS-441524 at concentrations of 1 µM (equivalent to 291 ng/mL) is the effective concentration (e.g. EC50) to inhibit the viral replication in-vitro (Murphy et al. Citation2018). The recommended daily dose rate of remdesivir in the treatment of FIP is not yet standardized but can be based on individual’s severity of symptoms. For example, the dosage can range from 10–15 mg/kg BW or 20 mg/kg BW every 12 h based on the presence of neurological symptoms (Taylor Citation2022; Malik Citation2022). This HPLC-FLD method was able to detect both EC50 and plasma GS-441524 concentrations that ranged from 23.7–190.1 ng/mL (n = 12), 24 h after various remdesivir dosages were administered (e.g. ranging from 9.87–15 mg/kg BW; IV, SC) to cats with FIP (). Compared to methods based on mass spectrometry, this HPLC-FLD method demonstrated a comparable LLOQ (19.5 ng/mL vs. 1.0–22.2 ng/mL), with a wider assay concentration range (e.g. up to 10,000 ng/mL vs. 187–2,500 ng/mL) ().
As FIP is a systemic disease accompanied with diverse and often severe clinical signs (Pedersen Citation2009), treatment with these antiviral agents is often accompanied with other medications (e.g. for initial supportive care or for sedation). This method (fluorescence detection range optimised to λex: 250 nm; λem: 475 nm) demonstrated excellent selectivity of GS-441524 against common medications utilised by clinicians managing cats with FIP in a clinical setting. This method was also highly selective for remdesivir and its other metabolites against interference from endogenous plasma compounds (including hemolysed samples) ( and ). While this method provided excellent selectivity of GS-441524, one of the limitations was that this study used external calibration, as an appropriate internal standard (IS) could not be identified that could be detected by the fluorescent spectrum for GS-441524. Generally, use of IS helps to improve uncertainty of measurement, such as instrument stability or loss of sample volume during extraction, especially when multistep extractions are required (Imre et al. Citation2019). However, if the method has a simple sample procedure and the method demonstrates good trueness and precision, then the superior benefit of the IS, is somewhat negated (Dolan Citation2012). Accordingly, while injection stability of our system was precise (0.3–0.4%, CV), simple one step protein precipitation technique was applied to purify the plasma sample (with average absolute recovery of 97.5%) in this study to overcome this issue.
5. Conclusion
This study developed and validated simple HPLC method utilising fluorescence detection to quantify plasma concentrations of GS-441524 applicable to monitor plasma concentrations of GS-441524 at least up to 24 hr after administrations of remdesivir (10 to 15 mg/kg BW, IV or SC); suitable for therapeutic monitoring (e.g. measuring plasma trough level of GS-441524) or for further pharmacokinetic studies of remdesivir administration and or GS-441524 in FIP affected cats.
Animal welfare and ethic statement
This study is a part of a broader clinical trial approved by The University of Sydney Animal Ethics Committee (2021/1874).
Disclosure statement
The authors declare no conflict of interest.
Data availability statement
The data supporting the findings of this study are available from Dr. Benjamin Kimble ([email protected]) upon request.
Correction Statement
This article has been republished with minor changes. These changes do not impact the academic content of the article.
Additional information
Funding
References
- Avataneo V, de Nicolò A, Cusato J, Antonucci M, Manca A, Palermiti A, Waitt C, Walimbwa S, Lamorde M, di Perri G, et al. 2020. Development and validation of a UHPLC-MS/MS method for quantification of the prodrug remdesivir and its metabolite GS-441524: a tool for clinical pharmacokinetics of SARS-CoV-2/COVID-19 and Ebola virus disease. J Antimicrob Chemother. 75(7):1772–1777. doi: 10.1093/jac/dkaa152.
- Alvarez JC, Moine P, Etting I, Annane D, Larabi IA. 2020. Quantification of plasma remdesivir and its metabolite GS-441524 using liquid chromatography coupled to tandem mass spectrometry. Application to a Covid-19 treated patient. Clin Chem Lab Med. 58(9):1461–1468. doi: 10.1515/cclm-2020-0612.
- Badgujar KC, Ram AH, Zanznay R, Kadam H, Badgujar VC. 2020. Remdesivir for COVID-19: a review of pharmacology, mechanism of action, in-vitro activity and clinical use based on available case studies. J Drug Delivery Ther. 10(4-s):264–270. doi: 10.22270/jddt.v10i4-s.4313.
- Beigel JH, Tomashek KM, Dodd LE, Mehta AK, Zingman BS, Kalil AC, Hohmann E, Chu HY, Luetkemeyer A, Kline S, ACTT-1 Study Group Members., et al. 2020. Remdesivir for the treatment of Covid-19. N Engl J Med. 383(19):1813–1826. doi: 10.1056/NEJMoa2007764.
- Coggins S, Kimble B, Norris MJ, Thompson FM, Malik R, Govendir M. 2022. Stability of remdesivir and in-vitro formation of GS-441524 using feline hepatic microsomes, blood and plasma. International Society for Companion Animal Infectious Diseases; Glasgow, Scotland.
- COVID-19 Treatment Guidelines Panel. 2019. Coronavirus Disease. (COVID-19) Treatment Guidelines. National Institutes of Health. [accessed 2022 Aug 29]. https://www.covid19treatmentguidelines.nih.gov/.
- Deb S, Reeves AA, Hopefl R, Bejusca R. 2021. ADME and pharmacokinetic properties of remdesivir: its drug interaction potential. Pharmaceuticals. 14(7):655. doi: 10.3390/ph14070655.
- Dolan JW. 2012. When should an internal standard be used? LCGC North America. 30:474–480.
- Du P, Wang G, Yang S, Li P, Liu L. 2021. Quantitative HPLC-MS/MS determination of Nuc, the active metabolite of remdesivir, and its pharmacokinetics in rat. Anal Bioanal Chem. 413(23):5811–5820. doi: 10.1007/s00216-021-03561-8.
- ICH Harmonised Guideline. 2022. Bioanalytical method validation and study sample analysis M10. ICH Harmonised Guideline: Geneva, Switzerland.
- Habler K, Brügel M, Teupser D, Liebchen U, Scharf C, Schönermarck U, Vogeser M, Paal M. 2021. Simultaneous quantification of seven repurposed COVID-19 drugs remdesivir (plus metabolite GS-441524), chloroquine, hydroxychloroquine, lopinavir, ritonavir, favipiravir and azithromycin by a two-dimensional isotope dilution LC–MS/MS method in human serum. J Pharm Biomed Anal. 196:113935. doi: 10.1016/j.jpba.2021.113935.
- Hamdy MM, Abdel Moneim MM, Kamal MF. 2021. Accelerated stability study of the ester prodrug remdesivir: recently FDA-approved Covid-19 antiviral using reversed-phase-HPLC with fluorimetric and diode array detection. Biomed Chromatogr. 35(12):e5212. doi: 10.1002/bmc.5212.
- Imre S, Tero-Vescan A, Dogaru MT, Kelemen L, Muntean D-L, Curticapean A, Szegedi N, Vari C-E. 2019. With or without internal standard in HPLC bioanalysis. A case studies. J Chromatogr Sci. 57(3):243–248. doi: 10.1093/chromsci/bmy106.
- Jorgensen SC, Kebriaei R, Dresser LD. 2020. Remdesivir: review of pharmacology, pre-clinical data, and emerging clinical experience for COVID-19. Pharmacotherapy: J Human Pharmacol Drug Therapy. 40(7):659–671. doi: 10.1002/phar.2429.
- Kokic G, Hillen HS, Tegunov D, Dienemann C, Seitz F, Schmitzova J, Farnung L, Siewert A, Höbartner C, Cramer P. 2021. Mechanism of SARS-CoV-2 polymerase stalling by remdesivir. Nat Commun. 12(1):279. doi: 10.1038/s41467-020-20542-0.
- Li Y, Cao L, Li G, Cong F, Li Y, Sun J, Luo Y, Chen G, Li G, Wang P, et al. 2022. Remdesivir metabolite GS-441524 effectively inhibits SARS-CoV-2 infection in mouse models. J Med Chem. 65(4):2785–2793. doi: 10.1021/acs.jmedchem.0c01929.
- Lipka E, Vaccher C. 2015. Quantitative analysis of drugs in biological matrices by HPLC hyphenated to fluorescence detection. Bioanalysis. 7(6):743–762. doi: 10.4155/bio.15.20.
- Malik R. 2022. Treatment of FIP in cats with subcutaneous remdesivir followed by oral GS-441524 tablets. [accessed 10 October]. https://sockfip.org/wp-content/uploads/2021/11/Remdesivir-Rx-from-Australia.pdf.
- Moneim MMA, Kamal MF, Hamdy MM. 2021. Rapid sensitive bioscreening of remdesivir in COVID-19 medication: selective drug determination in the presence of six co-administered therapeutics. Rev Analytical Chem. 40(1):323–333. doi: 10.1515/revac-2021-0141.
- Murphy BG, Perron M, Murakami E, Bauer K, Park Y, Eckstrand C, Liepnieks M, Pedersen NC. 2018. The nucleoside analog GS-441524 strongly inhibits feline infectious peritonitis (FIP) virus in tissue culture and experimental cat infection studies. Vet Microbiol. 219:226–233. doi: 10.1016/j.vetmic.2018.04.026.
- O’Reilly KJ, Fishman B, Hitchcock LM. 1979. Feline infectious peritonitis: isolation of a coronavirus. Vet Rec. 104(15):348. doi: 10.1136/vr.104.15.348.
- Pedersen NC. 2009. A review of feline infectious peritonitis virus infection: 1963–2008. J Feline Med Surg. 11(4):225–258. doi: 10.1016/j.jfms.2008.09.008.
- Pedersen NC, Perron M, Bannasch M, Montgomery E, Murakami E, Liepnieks M, Liu H. 2019. Efficacy and safety of the nucleoside analog GS-441524 for treatment of cats with naturally occurring feline infectious peritonitis. J Feline Med Surg. 21(4):271–281. doi: 10.1177/1098612X19825701.
- Saha A, Sharma AR, Bhattacharya M, Sharma G, Lee SS, Chakraborty C. 2020. Probable molecular mechanism of remdesivir for the treatment of COVID-19: need to know more. Arch Med Res. 51(6):585–586. doi: 10.1016/j.arcmed.2020.05.001.
- Taylor. 2022. An update on treatment of FIP in the UK, international society of feline medicine. [accessed 2022 October 10]. https://icatcare.org/app/uploads/2022/05/An-update-on-treatment-of-FIP-in-the-UKv2.pdf.
- Warren TK, Jordan R, Lo MK, Ray AS, Mackman RL, Soloveva V, Siegel D, Perron M, Bannister R, Hui HC, et al. 2016. Therapeutic efficacy of the small molecule GS-5734 against Ebola virus in rhesus monkeys. Nature. 531(7594):381–385. doi: 10.1038/nature17180.