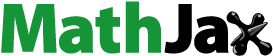
Abstract
Canine individual identification and parentage testing are essential in various fields, including forensics and breeding programs. This study aimed to develop and validate the Canine 25 A kit, a multiplex polymerase chain reaction (PCR) system designed to address these critical requirements. This novel system enables the simultaneous amplification of 24 canine autosomal short tandem repeat (STR) loci and one sex-determining marker. Validation of the Canine 25 A kit was conducted following the Scientific Working Group on DNA Analysis Methods (SWGDAM) guidelines, demonstrating significant sensitivity, high inhibitor tolerance, canine specificity within a mixture, species specificity, and precision in genotype determination. The Canine 25 A kit was crucial in resolving several forensic cases, such as casework samples from a dog attack incident and parentage determination. Its effectiveness in genotyping these samples highlights its significance in forensic applications. Population genetic parameter analysis revealed a high discriminatory power, as indicated by the calculated combined discrimination power (CDP) values for each breed exceeding 0.999 999 999 999, while the combined power of exclusion (CPE) surpassed 0.9999. Overall, the Canine 25 A kit offers a precise and dependable tool for canine individual identification and parentage determination.
1. Introduction
Dogs (Canis familiaris) are the most common household pets worldwide. In China, the number of domestic dogs in urban households has exceeded 54 million in 2021 (pethadoop.com), not including rural households. This large population makes accurate individual identification and parentage testing crucial (Linacre et al. Citation2011), which is also necessary to detect evidence types (e.g. hair, feces) in criminal cases involving canines. People consider dogs constant companions and share a close relationship with them. Therefore, the canine DNA in biological materials, such as saliva, blood, hair, or feces, which are abundant in daily surroundings, may sometimes be associated with criminal cases (Kun et al. Citation2013). Canine DNA can represent criminal evidence, whether a dog is directly involved or not (Pádár et al. Citation2001; Citation2002; Eichmann et al. Citation2004; Halverson and Basten Citation2005; Kanthaswamy et al. Citation2009; Tom et al. Citation2010). To exploit the potential information available from criminal evidence, a reliable method for typing canine DNA samples is required (Eichmann et al. Citation2005).
In 2008, we proposed the Canine 11 A STR kit as one of the first commercially available canine STR genotyping tools in China (Du Citation2007; Du et al. Citation2008). The kit consisted of 11 canine autosomal short tandem repeat (STR) loci (PEZ1, PEZ2, PEZ3, PEZ5, PEZ6, PEZ8, PEZ12, FH2010, FH2054, FH2132, and FH2611). In 2011, four novel STR loci (PEZ15, PEZ20, PEZ21 and FH2079) with high polymorphism and one canine sex-determined marker, DAmel, were selected and added to the kit. This construction generated the new canine multiplex STR amplification system, the Canine 17 A STR kit, using five fluorescent dyes for labeling (Ye et al. Citation2011; Qian et al. Citation2016). This nomenclature system has been applied in over 536 canines from 15 breeds, with a combined power of discrimination (CPD) of 0.999 999 992 and a combined power of exclusion (CPE) of 0.995 026. In forensic cases involving limited DNA samples, implementing a multiplex polymerase chain reaction (PCR) targeting highly polymorphic marker sites has enhanced efficiency and gives superior quality outcomes (Dayton et al. Citation2009). This implies that panels with more genetic markers can achieve significant validity in forensic practice cases, particularly given the higher likelihood of excluding potential matches due to the anticipated inbreeding prevalent across various dog breeds.
This study aimed to develop a higher-quality canine STR amplification kit with improved system detection efficiency. Recently, hundreds of canine STR markers have been discovered due to many research efforts (Zenke et al. Citation2011; Ogden et al. Citation2012), like the Canine Genotypes™ Panel 1.1 Kit (catalog number: F860S) and the Canine Genotypes™ Panel 2.1 Kit (catalog number: F864S) (Dayton et al. Citation2009; Kanthaswamy et al. Citation2018; Lee et al. Citation2021). Manufactured by Thermo Fisher Scientific, these two commercial STR amplification kits, consisting of 19 canine STRs, have been successfully used in fields such as canine breed identification, genetic analysis, and kinship analysis. Specific loci from these kits were used as references in developing a novel system. More specifically, we have updated the previous system by supplementing eight additional loci (FH2328, VGL3112, PEZ17, FH3313, FH2088, FH2001, FH2017, and FH2107) and integrated them into a novel system, Canine 25 A kit.
The Canine 25 A kit is characterized by more novel markers and improved identification capability, making it a powerful tool for canine individual identification and paternity tests. Multiple validation studies were conducted following the Scientific Working Group on DNA Analysis Methods (SWGDAM) guidelines to assess the performance of this new system.
2. Materials and methods
2.1. Marker selection and primer design
Twenty-four STR loci located dispersedly on different chromosomes with a sex determination marker were selected and assembled into a single system. Except for locus PEZ3, all the STR markers contained tetranucleotide repeat motifs. presents information about repeat motifs, size ranges, and located chromosomes of these markers.
Table 1. The information and primer details of Canine 25 A kit.
Pairs of primers were designed with amplicon sizes between 80–500 bp based on the same parameters using Primer 5.0. The primer specificity was verified by the Basic Local Alignment Search Tool (BLAST) function of GenBank on the National Center for Biotechnology Information (NCBI). Several pairs of primers were designed for each locus, but finally, only one pair exhibiting high efficiency and clean profiles with few pseudo peaks was identified as a candidate. To facilitate their arrangement in the multiplex, according to their amplicon size, primers of 24 STR loci and a sex-determining locus amelogenin were divided into five groups, and one primer of each pair was labeled by a fluorescent dye such as FAM, HEX, TAMRA, ROX, or PUR. Internal standard segments for size analysis, Maker SIZ-500 (AGCU ScienTech, Wuxi, China), were labeled with VIG. shows the markers and dye configuration.
2.2. DNA samples
Different types of biological canine samples, including blood, buccal swabs, and feces, were selected for testing. Remnants of samples (blood, buccal swabs, feces) following veterinary examination were provided by Jiangxi Provincial Key Laboratory of Police Dog Breeding and Behavior Science. Samples were obtained from 500 dogs representing 16 breeds widespread in China with the owner’s consent. Samples used for species specificity studies from chickens, cattle, fish, mice, pigs, rabbits, sheep, Escherichia coli (E. coli), and humans were collected from Homy Genetics Inc. (China). The blood spot samples were punched to 0.5 mm in diameter using a hole puncher for direct PCR amplification. The other biological samples that could not be amplified directly were extracted by the Chelex-100 or TIANamp Genomic DNA kit protocol (TIANGEN BIOTECH, Beijing, China).
2.3. Positive controls
A single canine sample with good amplification and a complete STR genotype was chosen as the standard positive control to ensure the accuracy and reliability of canine profiling. The DNA of positive control was extracted with the TIANamp Genomic DNA kit, and the extracted DNA concentration was quantified using a NanoDrop Spectrophotometer (Thermo Fisher Scientific, Waltham, USA) per the protocols.
2.4. Multiplex PCR amplification
Coamplification of 24 canine STR markers and one sex-related marker was performed on a Mastercycler® nexus thermal cycler (Eppendorf Corporate, Hamburg, Germany) with the following standard thermal cycling conditions: 2 min initial denaturation at 95 °C; 30 cycles for 30 s at 94 °C, 1 min at 60.5 °C, and 50 s at 72 °C; followed by a final extension for 60 min at 60 °C. The total reaction was optimized to 10 μL of volume, including 0.5–2 ng of DNA template, 2.0 μL 5× primer set (0.04–0.43 μM of each primer), 0.2 μL heat-activated C-Taq polymerase (5 U/μL), and 4.0 μL 2.5 × PCR mix (containing 125 mM Tris-HCl buffer, 125 mM KCl, 7.5 mM dNTPs, 5.0 mM MgCl2). The volume was adjusted to 10 μL using ddH2O. The C-Taq polymerase and PCR mix were from AGCU ScienTech, Wuxi, China.
2.5. Electrophoresis and analysis
Samples for fragment separation were prepared as follows: PCR product was first blended with a 10.0 μL mixture consisting of 9.5 μL deionized Hi-Di Formamide and 0.5 μL Maker SIZ-500 size standard (AGCU ScienTech, Wuxi, China). The samples were denatured at 95 °C for 3 min and then immediately chilled on ice for 3 min. Electrophoresis was performed on an Applied Biosystem 3130 Genetic Analyzer using the POP4 Polymer (Thermo Fisher Scientific, Waltham, USA), with the spectral calibration file established using the 6 Dye Matrix Standards (Du et al. Citation2019). Fragment sizes and genotyping were analyzed by GeneMapper ID-X software (Thermo Fisher Scientific, Waltham, USA).
2.6. Construction of allelic ladders
2.6.1. Monocloning of the target al.leles
A single-plex PCR procedure was performed using non-fluorescently labeled primers to obtain the frequent alleles of each marker for allelic ladders. The resulting PCR products were separated using 1.7% agarose gel electrophoresis and visualized by ethylene bromide staining. Subsequently, the target products were retrieved from the gel and purified.
The isolated target fragments were cloned into the pMD18-T vector and transformed into E. coli DH5α, followed by overnight incubation in Luria-Bertani medium (Chen et al. Citation2019). The recombinants were screened using the blue-white method. Recombinant colonies were identified using the blue-white screening method. After plasmid extraction and gene sequencing, the recombinant plasmids were preserved, and the bacterial cultures were stored in glycerol.
2.6.2. Allelic fragment amplification and one-single ladder preparation
Each allelic fragment from the same locus was amplified using the recombinant plasmid as a template, resulting in 151 allelic fragments. These selected fragments were then individually combined to create 25 single-locus ladders, ensuring a balanced concentration based on the peak height ratio with a target peak height above 70% (Zhou et al. Citation2014). The ladders of each single locus were extracted and purified using chloroform and were subsequently stored in the dark at −20 °C.
2.6.3. Assembling of allelic ladders
The allelic ladders of all 25 Canine loci were combined and adjusted based on the average peak height ratio of the single-locus ladder to achieve an average peak height value of over 400 relative fluorescence units (RFUs). Additionally, the average peak height ratio between loci marked with the same fluorescence dye was ensured to be above 60%, while the average peak height ratio between loci marked with different fluorescence dyes exceeded 60%. This careful adjustment and mixing of the allelic ladders ensured optimal performance and accurate analysis in subsequent experiments.
2.7. Developmental validation
2.7.1. PCR conditions/procedures
PCR conditions were optimized for the Canine 25 A kit by tests for ranges of reaction components, annealing temperature, or cycle number. Control DNA was used as the template and maintained at 0.5 ng in each reaction system. Conversely, reaction components, including primers, reaction mix, and C-Taq polymerase, were used in a series of concentrations, namely, 0.5×, 0.75×, 1×, 1.25×, and 1.5× (1× corresponds to the concentrations described in section 2.4). Furthermore, amplification was performed at different annealing temperatures (59, 59.5, 60.1, 60.5, 61.2, 61.7, and 62.5 °C) and cycle numbers (29, 30, and 31).
2.7.2. Sensitivity
Assay sensitivity was evaluated using serial dilutions of control DNA. One microliter of each DNA dilution was amplified in triplicate with the following template amounts: 2 ng, 1 ng, 500 pg, 250 pg, 125 pg, and 62.5 pg.
2.7.3. Species specificity
Species studies were performed using DNA samples from E. coli, humans, and different animal species (e.g. chickens, cattle, fish, mice, pigs, rabbits, and sheep). A DNA input amount of 1 ng was amplified with a Canine 25 A kit.
2.7.4. Mixture study
Mixture analysis experiments were conducted using well-characterized canine DNA samples. The mixtures were prepared at specific ratios of 1:1, 1:2, 1:5, 1:10, and 1:20 to evaluate the impact of DNA mixtures on the analysis, with the total amount of DNA constant at 1 ng. Additionally, mixtures combining dog saliva and human blood at the ratios mentioned above were designed to demonstrate the applicability of multiplex assay in forensic casework involving bites by domestic dogs. The human DNA was detected within these mixtures using the AGCU Expressmarker 16CS Kit (AGCU ScienTech, Wuxi, China).
2.7.5. Inhibitor study
To evaluate the anti-interference capacity of Canine 25 A kit, six common forensic inhibitors, hematin, hemoglobin, indigo, humic acid, calcium ion, and ethylene diamine tetra acetic acid (EDTA), were added to PCR systems at the following concentrations: 25, 50, 75, 100, or 150 μmol/L hematin; 50, 75, 100, 150, or 200 μmol/L hemoglobin; 4, 8, 12, 16, or 20 mmol/L indigo; 10, 15, 20, 25, or 30 mg/L humic acid; 0.4, 0.8, 1.2, 1.6, or 2.0 mmol/L calcium ion; and 0.3, 0.6, 0.9, 1.2, or 1.5 mmol/L EDTA (Chen et al. Citation2021). The amount of control DNA was maintained at 0.5 ng (Chen et al. Citation2019).
2.7.6. Reproducibility
Thirty randomly selected samples from the population study were analyzed independently by three separate laboratories, and the data were compared to determine the reproducibility. Furthermore, control DNA was detected to validate the consistency of genotype results using an Applied Biosystems 3130XL Genetic Analyzer and a 3500XL Genetic Analyzer.
2.7.7. Size precision
The size precision and accuracy of the Canine 25 A kit were evaluated by collecting fragment size data from three full injections of allelic ladder on the Applied Biosystems 3130XL Genetic Analyzer. The mean size was calculated for each allele, and the standard deviation was determined for the common alleles at each locus.
2.7.8. Balance of peak height
Eighty different dog samples from the population study were used to calculate the peak height balance for allele pairs at each locus. Homozygous and heterozygous peaks were first normalized by averaging the RFU value for each allele of heterozygous genotypes and dividing the RFU value of each homozygous peak by 2.
2.7.9. Stutter analysis
This study utilized a subset of 100 unrelated canine individual blood samples for stutter analysis. Stutter peaks were identified with one repeat smaller or larger than the true allele. The peak height of each stutter peak was divided by the peak height of the corresponding true allele to calculate the stutter ratio. The analytical threshold for the minimum stutter peak height was set at 20 RFUs to ensure the inclusion of all stutter peaks.
The average stutter value, standard deviation, and stutter threshold were determined for each analyzed sample. These parameters provide insights into the characteristics of stutter peaks within the canine DNA profiles to understand their variability and distribution; it is essential to accurately interpret and compare genetic profiles in forensic and genetic research applications.
2.7.10. Case sample study
Five casework samples were extracted with Chelex-100 resin and amplified using a Canine 25 A kit. DNA was obtained from bloodstains taken from three reference dogs, Rottweiler, German Shepherd Dog, and Poodle breed, and from saliva swabs from a piece of shirt and pants showing dog bite marks. The Likelihood Ratio (LR) was utilized in individual identification through DNA evidence. This entails evaluating the probability of observing the DNA evidence under two hypotheses: LR = P(E | S)/P(E | NS), where P(E | S) is the probability of observing the DNA evidence given that the suspect is the true source of DNA sample, and P(E | NS) is the probability of observing the DNA evidence given that the suspect is not the true source of DNA sample (Buckleton et al. Citation2007; Sands Citation2007). In this particular case, the DNA evidence was derived from stains on clothing, and the LR can be construed as follows: P(E | S) denotes the likelihood that the stains on the clothing were left by the suspect’s dog, whereas P(E | NS) represents the probability that the stains on the clothing were left by random dogs unrelated to the case. The LR measures the strength of evidence for individual identification, with higher LR values indicating more robust evidence supporting one hypothesis over the other.
Bloodstains from one duo family belonging to the Shiba breed were tested. The parentage index (PI) of each locus and combined paternity index (CPI) were calculated. STR frequency involved in LR and PI calculation came from the statistics of population genetic analysis in this study.
2.7.11. Population studies
A total of 500 canine samples from Nanchang Police Dog Base in different sample types, including 432 blood stains, 63 buccal swabs, and 5 fecal samples, were profiled using a Canine 25 A kit. Population statistical parameters such as allele frequencies, observed heterozygosity (Hobs), polymorphic information content (PIC), discrimination power (PD), power of exclusion (PE), typical paternity index (TPI), and the p values of Hardy-Weinberg equilibrium were analyzed by STRAF (Gouy and Zieger Citation2017; Khacha-Ananda and Mahawong Citation2021). Additionally, the combined discrimination power (CDP) and the CPE were calculated as (Pi represents the ith value of PD or PE) (Chen et al. Citation2019).
3. Results and discussion
3.1. Allelic ladders
By adjusting the mixed volume ratio of the allele ladder, the peak height of each allele exceeded 400 RFUs, and the average peak height ratio between loci exceeded 50%; presents the electropherogram displaying the allelic ladder.
3.2. PCR conditions/procedures
The recommended optimal reaction volume for the Canine 25 A kit is described in Section 2.4; however, some laboratories decrease the total reaction volume to reduce costs. Such a reduction in the reaction system may decrease the success rate of sample detection or even the detection failure. The primers, reaction mix, and Taq polymerase are critical for multiplex PCR assays. Herein, we conducted optimization and adjustments on these three components to maximize the robustness and performance of the Canine 25 A kit.
illustrates the detection results of DNA profiles at different concentrations of PCR conditions. Complete profiles were obtained at all concentrations of C-Taq DNA polymerase. However, when the reaction mix concentration was reduced to 0.5×, only 32% of the loci were detected. Regarding primers, the percentage of detected loci was 0% and 56% when the concentration was reduced to 0.5× and 0.75×, respectively. Consistently increasing primer concentrations above 1.25× resulted in the gradual generation of allelic impurity peaks. displays the electrophoresis profiles corresponding to the different component concentrations.
Figure 3. The detection rate of alleles with the Canine 25 A kit at different concentrations of primers, reaction mix and C-Taq DNA polymerase.

Figure 4. Electropherogram of different PCR reaction components in Canine 25 A kit: A) 0.75× reaction mix, B) 1× primers; C) 0.5× C-Taq DNA polymerase.

For a 10.0 μL reaction volume system, the recommended optimal conditions are as follows: 4 μL of PCR mix, 0.4 μL of C-Taq DNA polymerase, and 2 μL of primers. Implementing these conditions resulted in the efficient acquisition of DNA profiles, ensuring the robustness and performance of the novel system.
presents the electrophoresis profiles corresponding to the annealing temperature and cycle number. The greatest product yield occurred at an annealing temperature between 59–60.1 °C, with accurate products obtained between 61.2– 62.5 °C. When the annealing temperature decreased to 60.1 °C, unwanted impurity allelic peaks appeared. Nevertheless, when the annealing temperature was increased to 62.5 °C, the peak heights of some loci decreased, and PEZ1/2 and DAmel showed allelic dropout. Therefore, profiles were efficiently and accurately obtained at an annealing temperature of 60.5 °C, which was the optimal and recommended annealing temperature.
Figure 5. DNA profiles obtained from Canine 25 A kit at different PCR conditions: A) annealing temperatures and B) cycle numbers.

A total of 0.5 ng of control DNA was amplified at 29, 30, and 31 cycles on an Eppendorf Mastercycler Nexus Thermal Cycler. Full profiles were unfailingly obtained at all three cycle numbers, while the peak heights increased as the number of cycles increased. A cycle number of 30 were indicated to be optimal for the novel developed Canine 25 A kit because this cycle number maximized reagent sensitivity and minimized the occurrence of impurity peaks.
3.3. Sensitivity
For the sensitivity test of the Canine 25 A kit, a series of concentrations of control DNA, 2.0, 1.0, 0.5, 0.25, 0.125, and 0.0625 ng, were used as the template and added to the reaction volume of 25 μL (). Full profiles of 24 loci and a sex-determining locus amelogenin were consistently obtained with the reaction containing 0.125 ng of DNA (). When the DNA was less than 0.125 ng, allelic dropouts or amplification failure occasionally occurred, indicating insufficient template DNA. Consequently, in the 25 μL reaction system, the Canine 25 A kit sensitivity was determined to be 0.125 ng/25 μL.
Figure 6. Heatmap summarizing the sensitivity results of six serial dilutions (from 2.0 ng to 0.0625 ng) of control DNA with three biological replicates (-1, -2, -3), using a threshold set at 50 RFU. Green represents peak height ratio >50% within the same locus. Yellow represents peak height ratio <50% and >30% within the same locus. Red represents allele loss.

3.4. Species specificity
The novel-developed Canine 25 A kit was tested using DNA samples from different animal species to determine any cross-reactivity (Liu et al. Citation2019). No amplification products were detected for samples from chickens, cattle, fish, mice, pigs, rabbits, sheep, E. coli, and humans (), which indicated that the system was robust and unlikely to be affected by the presence of genetic material from these animal species.
3.5. Mixture study
A mixture study was conducted to evaluate the ability of the Canine 25 A kit to identify the minor contributor (Ensenberger et al. Citation2010). In the dog-dog mixed DNA samples, all alleles were distinguishable in the 1:1, 1:2, and 1:5 mixtures; displays the DNA profiles of mixture samples with a mixing ratio of 1:5. However, in the 1:10 mixture, some alleles of the minor contributor were indistinguishable from stutter products, resulting in a loss of information. Notably, when the mixture ratio increased to 1:20, no profiles of the minor contributor could be obtained.
Figure 9. DNA profile analysis of mixture study: A) dog-dog mixture (1:5), B) human-dog mixture (1:5), and C) human genotype detection from mixture DNA using AGCU Expressmarker 16CS Kit.

In the mixtures of dog saliva and human blood, all alleles were successfully identified in 1:1, 1:2, and 1:5 (with dog saliva DNA as the minor contributor). However, when the mixing ratio reached 1:10, more than half of the peaks started diminishing. As expected, complete human DNA profiles were obtained in all five ratios using the AGCU Expressmarker 16CS Kit; illustrates the genotyping of the mixture of dog saliva and human blood at a ratio of 1:10. These findings suggest that the Canine 25 A kit exhibits promising performance in achieving complete genotyping for dog-dog mixed samples and dog saliva-human blood mixed samples, even when the contribution of dog DNA is as low as 20%.
3.6. Inhibitor study
As DNA samples from crime scenes usually contain inhibitors that may interfere with PCR amplification and sometimes even cause complete amplification failure, it is important to validate the robustness and anti-interference quality of the novel developed system (Green et al. Citation2013; Zhu et al. Citation2015). shows that complete profiles were obtained with concentrations up to 25 μmol/L hematin, 50 μmol/L hemoglobin, 4 mmol/L indigo, 10 mg/L humic acid, 0.8 mmol/L calcium ion, and 0.6 mmol/L EDTA. When the concentrations exceeded these levels, obvious inhibition of the system occurred, reflected as allelic dropouts at some loci.
3.7. Reproducibility
A reproducibility study was performed to validate the reliability and accuracy of the developed Canine 25 A kit by testing 30 samples in different laboratories. The results showed that allele calls of all samples were identical across different analyses. To demonstrate the suitability of the system for use on different capillary electrophoresis (CE) platforms, control DNA was examined using both ABI 3130XL and ABI 3730XL. The genotype of the same locus obtained revealed perfect consistency between the two CE methods ().
3.8. Size precision study
The size precision results indicated that very little variation at each locus was observed in the size of the Canine 25 A allelic ladder, and most allele deviations were nearly 0.05 bases. The maximum standard deviation was close to 0.19 bases at locus VGL3112 (). These results demonstrate that the Canine 25 A kit has sufficient ability to ensure accurate genotyping of samples.
3.9. Balance of peak height
Two different types of peak balance were evaluated to assess the overall Canine 25 A STR kit performance. The intracolor balance was calculated to assess the peak balance of loci marked with the same fluorescent dye (Zhou et al. Citation2014). illustrates that the peak height ratios between different loci within the same dye channel were 59.23% for the blue dye, 46.04% for the green dye, 41.37% for the yellow dye, 54.09% for the red dye, and 55.57% for the purple dye. displays the average RFU values of each group of loci. The average RFU value for the blue dye was approximately 4071 for the blue dye, 3976 for the green dye, 2750 for the yellow dye, 3578 for the red dye, and 5459 for the purple dye. Therefore, the lowest value of peak height ratio between loci labeled with different fluorescent dyes was the minimum RFU at 2750 divided by the maximum at 5459, equal to approximately 50.4%. These results showed that the Canine 25 A kit has a good balance.
3.10. Stutter analysis
summarizes the relevant parameters for assessing stutter in the Canine 25 A kit, including the minimum, maximum, and average stutter values, standard deviation, and stutter threshold for each locus. Notably, the FH2107 locus exhibited the highest values across all parameters, indicating a higher propensity for stuttering at this locus. The average stutter percentage for FH2107 was determined to be 19.40%. Accordingly, the recommended stutter filter for GeneMapper IDX software in the Canine 25 A kit was defined as the average value plus three standard deviations.
Table 2. Stutter values for the 25 canine STRs included in the Canine 25 A kit.
3.11. Case samples study
Canine STR profiling was used to test and analyze the performance in a dog attack incident using the Canine 25 A kit. All three bloodstains from different suspected dogs yielded a full canine-specific STR profile. Meanwhile, saliva stains on the shirt and pants only provided a partial profile (); reveals that the Rottweiler sample shared 15 identical loci with the shirt and 20 with the pants. The combined discrimination power (CPD) for these 15 and 20-locus sets was calculated to be 0.999 999 999 983 876 and 0.999 999 999 999 982, respectively, satisfying the system efficiency criteria of individual identification. The likelihood ratios were determined to be 1.9620 × 1022 and 5.9361 × 1028, supporting that the samples taken from the shirt, pants, and Rottweiler originated from one individual.
Figure 14. DNA profiles obtained from case samples related to a dog attack incident using the Canine 25 A kit: A) Rottweiler, B) Poodle, C) German Shepherd Dog, D) shirt with dog bite marks, and E) pants with dog bite marks.

Table 3. The genotype of casework samples.
Full profiles were obtained in the father-son duo paternity case, and no locus showed genetic incompatibilities (). The paternity index value per marker was calculated, and the final cumulative paternity index was 4.8568 × 108, confirming the parenthood of the tested family.
Table 4. The parentage index of Canine 24 STR loci for a case involving a father-son duo.
3.12. Population studies
Full DNA profiles were successfully obtained from different sample types of bloodstains, buccal swabs, and feces. depicts the forensic parameters of 24 canine STR loci in 16 canine breeds, revealing insignificant deviations from Hardy-Weinberg expectations after Bonferroni corrections (p > 0.05/24 = 0.0021), except for the FH2079 locus in Schnauzer, FH2088 in Rottweiler, PEZ15 in Chinese Kunming dog, FH2328 in Golden Retriever, PEZ2 in Poodle and PEZ6 and PEZ8 in Labrador Retriever.
Figure 15. Forensic parameters of 24 canine STR loci in 16 canine breeds. The parameters provided include polymorphism information content (PIC), power of discrimination (PD), observed heterozygosity (Hobs), power of exclusion (PE), typical paternity index (TPI), and p value of Hardy-Weinberg’s equilibrium (p-HW). Each breed is represented by a distinct letter label. The breeds included in the figure are: A) Italian cane corso, B) bloodhound, C) Schnauzer, D) Rottweiler, E) Chinese Kunming dog, F) Welsh Corgi Pembroke, G) Golden Retriever, H) Shiba, I) German spitz, J) Bichon Frise, K) German Shepherd dog, L) French bulldog, M) Poodle, N) Labrador Retriever, O) Belgian Shepherd dog, and P) English Springer Spaniel.

The PIC indicated that the marker can establish polymorphisms in populations, and the results showed that FH2107 and FH3313 gave a higher genetic polymorphism over 0.6 compared with the PICs in each canine breed (Khacha-Ananda and Mahawong Citation2021). illustrates detailed information on these breeds and their cumulative paternity indices. The CDP values for each breed were exceeded 0.999 999 999 999, and the CPE was over 0.9999. These results suggest that the Canine 25 A kit is suitable for individual identification and paternity testing, providing high accuracy and reliability.
Table 5. Specific information and cumulative paternity index of 16 Canine breeds.
4. Conclusion
The Canine 25 A kit was developed to enhance canine individual identification and parentage determination. This multiplex system offers an in-depth examination of 25 distinct canine STR loci, allowing for full genotyping even when working with minimal DNA template quantities as low as 0.125 ng. Several tests were conducted to validate the analytical performance of this kit, including PCR conditions, cross-reactivity, and stability studies, yielding consistently reliable profiles. The validation study has firmly established that the Canine 25 A kit exhibits significant sensitivity, high inhibitor tolerance, canine specificity within a mixture, species specificity, and precision in genotype determination.
In canine population analysis, the Canine 25 A kit demonstrated its effectiveness by generating complete and distinct STR profiles for 500 individuals sourced from various sample types, representing 16 different breeds. It demonstrated a high level of accuracy and reliability with CDP values surpassing 0.999 999 999 999 for each breed and a CPE exceeding 0.9999. These results indicate that the Canine 25 A kit is suitable for precise and dependable individual identification and paternity testing. Furthermore, the practical applications of this kit extended to successfully identifying casework samples involved in real cases, including one related to a dog attack incident and another concerning a father-son duo. The genetic evidence derived from the Canine 25 A kit significantly contributed to resolving these cases by furnishing critical investigative insights.
The Canine 25 A kit has proven durability and efficacy in canine forensic testing. Its significant potential in forensic applications contributed to the progression of canine forensic genetics.
Disclosure statement
No potential conflict of interest was reported by the authors.
Additional information
Funding
References
- Buckleton JS, Curran JM, Gill P. 2007. Towards understanding the effect of uncertainty in the number of contributors to DNA stains. Forensic Sci Int Genet. 1(1):20–28. doi: 10.1016/j.fsigen.2006.09.002.
- Chen L, Du W, Wu W, Yu A, Pan X, Feng P, Feng C, Li C, Xu L, Liu C. 2019. Developmental validation of a novel six-dye typing system with 47 A-InDels and 2 Y-InDels. Forensic Sci Int Genet. 40:64–73. doi: 10.1016/j.fsigen.2019.02.009.
- Chen L, Pan X, Wang Y, Du W, Wu W, Tang Z, Xiao C, Han X, Liu C, Liu C. 2021. Development and validation of a forensic multiplex system with 38 X-InDel Loci. Front Genet. 12:1420. doi: 10.3389/fgene.2021.670482.
- Dayton M, Koskinen MT, Tom BK, Mattila A-M, Johnston E, Halverson J, Fantin D, DeNise S, Budowle B, Smith DG, et al. 2009. Developmental validation of short tandem repeat reagent kit for forensic DNA profiling of canine biological material. Croat Med J. 50(3):268–285. doi: 10.3325/cmj.2009.50.268.
- Du W, Feng P, Huang H, Wu W, Zhang L, Guo Y, Liu C, Liu H, Liu C, Chen L. 2019. Technical note: developmental validation of a novel 6-dye typing system with 36 Y-STR loci. Int J Legal Med. 133(4):1015–1027. doi: 10.1007/s00414-018-1864-y.
- Du W, Xiong Y, Ye J, Hua Q. 2008. Genetic polymorphism of Eleven Canine STR Loci. J Forensic Med. 24(6):425–428.
- Du W. 2007. Construction of a fluorescent multiplex amplification system for 11 Canine STR Loci (Chinese), Nanchang university. doi:10.7666/d.y1238756.
- Eichmann C, Berger B, Reinhold M, Lutz M, Parson W. 2004. Canine-specific STR typing of saliva traces on dog bite wounds. Int J Legal Med. 118(6):337–342. doi: 10.1007/s00414-004-0479-7.
- Eichmann C, Berger B, Steinlechner M, Parson W. 2005. Estimating the probability of identity in a random dog population using 15 highly polymorphic canine STR markers. Forensic Sci Int. 151(1):37–44. doi: 10.1016/j.forsciint.2004.07.002.
- Ensenberger MG, Thompson J, Hill B, Homick K, Kearney V, Mayntz-Press KA, Mazur P, McGuckian A, Myers J, Raley K, et al. 2010. Developmental validation of the PowerPlex 16 HS System: an improved 16-locus fluorescent STR multiplex. Forensic Sci Int Genet. 4(4):257–264. doi: 10.1016/j.fsigen.2009.10.007.
- Gouy A, Zieger M. 2017. STRAF-A convenient online tool for STR data evaluation in forensic genetics, Early View. Forensic Sci Int Genet. 30:148–151. doi: 10.1016/j.fsigen.2017.07.007.
- Green RL, Lagacé RE, Oldroyd NJ, Hennessy LK, Mulero JJ. 2013. Developmental validation of the AmpFSTR NGM SElect PCR Amplification Kit: a next-generation STR multiplex with the SE33 locus. Forensic Sci Int Genet. 7(1):41–51. doi: 10.1016/j.fsigen.2012.05.012.
- Halverson J, Basten C. 2005. A PCR multiplex and database for forensic DNA identification of dogs. J Forensic Sci. 50(2):352–363. doi: 10.1520/JFS2004207.
- Kanthaswamy S, Oldt RF, Montes M, Falak A. 2018. Comparing two commercial domestic dog (Canis familiaris) STR genotyping kits for forensic identity calculations in a mixed-breed dog population sample. Anim Genet. 50(1):105–111. doi: 10.1111/age.12758.
- Kanthaswamy S, Tom BK, Mattila A-M, Johnston E, Dayton M, Kinaga J, Erickson BJ-A, Halverson J, Fantin D, DeNise S, et al. 2009. Canine population data generated from a multiplex STR kit for use in forensic casework. J Forensic Sci. 54(4):829–840. doi: 10.1111/j.1556-4029.2009.01080.x.
- Khacha-Ananda S, Mahawong P. 2021. Genetic analysis of 12 X-short tandem repeats loci in a northern Thai population. Med Sci Law. 61(1):34–43. doi: 10.1177/0025802420965000.
- Kun T, Lyons LA, Sacks BN, Ballard RE, Lindquist C, Wictum EJ. 2013. Developmental validation of Mini-DogFiler for degraded canine DNA. Forensic Sci Int Genet. 7(1):151–158. doi: 10.1016/j.fsigen.2012.09.002.
- Lee M, Hwang IK, Jung JY, Kim JY, Chang M, Moon S. 2021. Construction of an in-house allelic ladder for Canine Genotypes Panel 2.1 Kit. J Forensic Sci. 66(6):2362–2368. doi: 10.1111/1556-4029.14812.
- Linacre A, Gusmão L, Hecht W. 2011. ISFG: recommendations regarding the use of non-human (animal) DNA in forensic genetic investigations. Forensic Sci Int Genet. 5(5):501–5. doi: 10.1016/j.fsigen.2010.10.017.
- Liu Y, Guo Y, Jin X, Mei S, Zhu B. 2019. Developmental validation study of a 24-plex Y-STR direct amplification system for forensic application. Int J Legal Med. 134(3):945–951. doi: 10.1007/s00414-019-02220-z.
- Ogden R, Mellanby RJ, Clements D, Gow AG, Powell R, Mcewing R. 2012. Genetic data from 15 STR loci for forensic individual identification and parentage analyses in UK domestic dogs (Canis lupus familiaris). Forensic Sci Int Genet. 6(2):63–65. doi: 10.1016/j.fsigen.2011.04.015.
- Pádár Z, Angyal M, Egyed B, Füredi S, Woller J, Zöldág L, Fekete S. 2001. Canine microsatellite polymorphisms as the resolution of an illegal animal death case in a Hungarian zoological gardens. Int J Legal Med. 115(2):79–81. doi: 10.1007/s004140100222.
- Pádár Z, Egyed B, Kontadakis K, Füredi S, Woller J, Zöldág L, Fekete S. 2002. Canine STR analyses in forensic practice. Observation of a possible mutation in a dog hair. Int J Legal Med. 116(5):286–288. doi: 10.1007/s00414-002-0302-2.
- Qian S, Du W, Liu C, Yang Q, Ye J. 2016. Study on the direct amplification system of canine blood spot STR. Forensic Sci Technol. 41(4):3.
- Sands JM. 2007. Forensic DNA typing: Biology, technology, and genetics of STR markers by John M. Butler. JSTOR. 47(2):245–249.
- Tom BK, Koskinen MT, Dayton M, Mattila A-M, Johnston E, Fantin D, Denise S, Spear T, Smith DG, Satkoski J, et al. 2010. Development of a nomenclature system for a canine STR multiplex reagent kit. J Forensic Sci. 55(3):597–604. doi: 10.1111/j.1556-4029.2010.01361.x.
- Ye J, Du W, Yang Q, Tang X, Zheng W, Xiong Y, Zhao H, Fu P, Lan K, Wang B. 2011. Application of 17A STRin police dog DNA identification (Chinese). Forensic Sci Technol. 3(2):11–13. doi: 10.3969/j.issn.1008-3650.2011.02.003.
- Zenke P, Egyed B, Zöldág L, Pádár Z. 2011. Population genetic study in Hungarian canine populations using forensically informative STR loci. Forensic Sci Int Genet. 5(1):e31–e36. doi: 10.1016/j.fsigen.2010.03.013.
- Zhou H, Wu D, Chen R, Xu Y, Xia Z, Guo Y, Zhang F, Zheng W. 2014. Developmental validation of a forensic rapid DNA-STR kit: expressmarker 16. Forensic Sci Int Genet. 11:31–38. doi: 10.1016/j.fsigen.2014.02.008.
- Zhu B-F, Zhang Y-D, Shen C-M, Du W-A, Liu W-J, Meng H-T, Wang H-D, Yang G, Jin R, Yang C-H, et al. 2015. Developmental validation of the AGCU 21 + 1 STR kit: a novel multiplex assay for forensic application. Electrophoresis. 36(2):271–276. doi: 10.1002/elps.201400333.