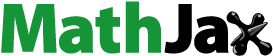
ABSTRACT
Faecal contamination across the lettuce value chain was assessed in Maputo, Mozambique. Escherichia coli was used as an indicator of faecal contamination, with concentrations ranging from 3.4 to 5.7 log units/100 ml in groundwater, river water and partially treated wastewater. Municipal tap water used to wash lettuce heads in the markets had lower than 1 log unit/100 ml. Irrespective of the source of irrigation water, the lettuce heads were contaminated throughout the value chain, with concentrations ranging between 6.5 and 7.8 log units/100 g. Interventions and awareness raising should be applied at every stage of the value chain.
Introduction
Urban and peri-urban agriculture has been increasingly practised in many countries due to its contribution to economic development, job creation, and food security (Orsini et al., Citation2013; Whittinghill & Rowe, Citation2012). These farming activities take place on small plots within or around urban areas (Orsini et al., Citation2013; Whittinghill & Rowe, Citation2012). The high cost of freshwater sources and the availability of municipal wastewater (Niquice Janeiro et al., Citation2020) are driving forces for the use of wastewater as a source for irrigation in (peri-)urban agriculture (Drechsel et al., Citation2008; Keraita et al., Citation2008; Scheierling et al., Citation2011).
If a wastewater treatment plant (WWTP) exists, there is often a lack of capacity to properly treat the conveyed wastewater (Nansubuga et al., Citation2016; Niquice Janeiro et al., Citation2020; Thebo et al., Citation2017). In Maputo, the capital of Mozambique, peri-urban agriculture is practised in the Infulene Valley, producing mainly horticultural crops such as lettuce and cabbage to supply the local informal markets of Greater Maputo (Halder et al., Citation2018; Salamandane et al., Citation2020; Salvador et al., Citation2016). The sources of irrigation water (each with different quality) are groundwater (GW), river water (RW), and partially treated wastewater (WW) from the Infulene WWTP. Using a specific water source for irrigation in Infulene Valley depends on the location and accessibility of the water source from the farm. Some crops produced in the Infulene area are irrigated with untreated or partially treated wastewater and are consumed raw, which can cause a severe health risk for consumers and farmers (Hamilton et al., Citation2006; Matangue et al., Citation2018; Urbano et al., Citation2017; Woldetsadik et al., Citation2017).
The WHO guidelines, developed in 1989, are extensively used in many developing countries, including Mozambique, to improve and regulate the use of wastewater in agriculture. Nevertheless, the WHO (Citation1989) guidelines only assess the water quality at the point of use and thus overlook the risk of contamination across the value chain, encompassing agricultural practices, handling, market practices, and consumer practices. Therefore, the WHO updated its guidelines in 2006 to incorporate Disability Adjusted Life Years, a metric based on specific regional conditions and supported by quantitative microbial risk assessment models. The 2006 WHO guidelines propose a combination of measures to reduce crop pathogen levels at different stages of production, i.e., pre-farm, farm-based, and post-harvest (World Health Organization, Citation2006). The combination of measures depends on the context and needs to be worked out by looking at the hazard analysis and critical control points (HACCPs).
This work follows up on a previous study investigating sewage-contaminated irrigation water for lettuce production in the Infulene Valley (Matangue et al., Citation2018). Also, a desktop review showed that contamination could occur across the production value chain (Niquice Janeiro et al., Citation2020). We hypothesized that health risks are not limited to unsafe farm-level irrigation practices but can also arise elsewhere in the value chain, including handling, transportation, and selling practices. This hypothesis is linked to the findings of Sousa et al. (Citation2021), who investigated vegetable contamination within the Maputo markets and recommended an assessment of vegetable contamination throughout the value chain. Our study serves as a fundamental step in shaping the pathways for intervention in collaboration with stakeholders in the lettuce production value chain. This work provides the possibility of understanding the contextual urban irrigation and cultivation (Veldwisch et al., Citation2024) and discusses the potential for hazard analysis, identifying points for health risk reduction.
Materials and methods
The study sites in the Infulene Valley () were selected based on their differences in irrigation water sources. Four farm sites were selected: two farms located at opposite points across the river irrigating with groundwater, i.e., GW1 and GW2; a farm irrigating with river water (RW3); and a farm irrigating with partially treated wastewater (WW4). The distances between the sites varied between 200 and 700 m and the sampling plot sizes were 4–6 m2.
Figure 1. Farm sampling sites from irrigating sources (groundwater (GW), i.e., GW1 and GW2, river water (RW3) and partially treated wastewater (WW4)) at the Infulene Valley in Maputo, Mozambique, and markets where the crops were sold in nearby Maputo and Matola.

Study area description
Infulene Valley, also known as Maputo’s green belt for crop production, is a natural depression in the flat land area west of the capital city Maputo, located in the southern part of Mozambique. The Infulene Valley has an extent of 20 × 0.5 km and covers some of the peri-urban areas of Maputo and Matola cities, the two most populated cities in the country (Sitoe & Mina Pinto, Citation2019). Small-scale agriculture in this area mainly produces horticultural crops such as lettuce, kale, and cabbage. Farmers use manual irrigation, i.e., watering cans to irrigate the crops. The crops are mainly produced for commercial purposes. Lettuce is the most common crop in the area, comprising 64% of all crops grown in Maputo (Smart et al., Citation2015). The cultivated crops are sold in local markets across the Greater Maputo area, which includes the area of Maputo, Matola and Boane cities, and Marracuene districts (Batran et al., Citation2018; Salvador et al., Citation2016; Sitoe & Mina Pinto, Citation2019). Around 86% of the producers sell lettuce during the wet season (September–March), and nearly half of the producers sell during the dry season (April–August; Smart et al., Citation2016).
Type of water sources
This study assesses the quality of water used for irrigation and washing of lettuce (Lactuca sativa) heads sold in the markets of Maputo City. Different water sources are used for irrigation in Infulene Valley, classified as groundwater, river water, and partially treated wastewater. Groundwater for irrigation is collected from farmers’ artisanal wells and drainage ditches (Salamandane et al., Citation2020). The Infulene river flows through the valley, which also serves as an irrigation source despite being polluted by the discharges from on-site sanitation systems of houses on the river’s banks. The river also receives effluent discharges from factories upstream, for instance, a beverage industry and a pulp and paper industry (Scarlet & Bandeira, Citation2014). Furthermore, the effluent of the WWTP also discharges into the Infulene river (Salamandane et al., Citation2020; Taviani et al., Citation2008). The WWTP, consisting of a pond system, treats 5–10% of the sewage generated in the city of Maputo (Arsénio et al., Citation2018; Rietveld et al., Citation2016) but is not well maintained (Rietveld et al., Citation2016) and lacks quality monitoring. Moreover, sludge removal has never taken place since its construction, and the tanker trucks discharge septic content directly into the anaerobic ponds (Arsénio et al., Citation2018). As a result, the volume of sediments and the growth of algae and macrophytes in the ponds reduce the treatment capacity and efficiency ().Footnote1 Nevertheless, the WWTP effluent is used for small-scale irrigation in the downstream peri-urban areas.
Sampling
This study was conducted in Infulene Valley from February to July 2019 (6 months), which corresponds to the transition period from rainy to dry season. Lettuce handling practices were observed across the entire value chain, and sampling was done accordingly. Farmers were observed for two hours during their farming activities (from 6.30am to 8.30am) to understand their irrigation procedures, manure application, and harvesting methods. Further, vendors were observed for 3 hours in the selected markets (from 900am to 12.00pm) to assess (1) whether they washed the crops to remove farm residuals upon arrival at the market and (2) how the crops were displayed and stored. The sampling timing and the sequence were set based on the observed practices.
The duration of sampling was three days per sampling week, and each sampling day was followed by laboratory analysis. Samples were collected for 6 weeks under normal farming conditions, and the harvested lettuce was followed to the market sites where they were sold. The lettuce samples collected at the market corresponded to those originating from the sites selected for this study. Samples were collected from two markets (one in Maputo city, the other in Matola) and one street vendor in Matola. Lettuce from the GW2 and WW4 sites was sold in one market. At the other market, the lettuce was exclusively from RW3, while the street vendor obtained lettuce from the GW1 site. The weekly sampling routine is described below.
On the first sampling day, the irrigation water from the sources at the farm was sampled ().
On the second day, the lettuce was collected at the farm and market. At the farm sites, the lettuce samples were collected 24 hours after the last irrigation. At the market sites, lettuce samples were taken before and after washing with municipal water. In addition, the fresh municipal water and the water after being used for washing the lettuce heads were collected and further analysed.
On the third day, lettuce samples were collected only when the impact of delayed harvest was researched. Lettuce samples were then collected 48 hours after the last irrigation at the farm ().
Table 1. Overview of microbial monitoring of irrigation water and lettuce heads in Infulene Valley, Maputo, from February to July 2019.
The water and lettuce samples were analysed for the presence and concentration of the indicator organism Escherichia coli, which is the commonly used indicator for public health assessment (Edberg et al., Citation2000). We only measured the indicator pathogen E. coli in this research. Other pathogenic organisms, such as different bacterial indicators, as well as protozoa, viruses, and worms, should be included for a complete understanding of pathogen contamination. Furthermore, it will be essential to conduct research on non-organic contamination, particularly focusing on the presence of heavy metals.
A total of 78 water samples () were collected as grab samples at the farm and markets from 07.00am to 09.00am. After collection, water samples were placed in 250-ml glass sterile bottles and stored in a cool box with ice packs for transportation. They were kept in the cool box until processing within 2–8 hours after collection. A total of 96 lettuce samples () were randomly collected early in the morning (7.00–9.00am). Each sample consisted of two lettuce heads, obtained from the state they were available for consumer purchase. The samples were placed in sterile bags, then stored in coolers with ice packs and transported to the laboratory for analyses.
At the farm, samples were collected at normal harvest and delayed harvest at each site (GW1, GW2, RW3, WW4). In , LF refers to lettuce samples at the farm after normal harvest. The normal harvest corresponds to lettuce samples collected from the farm locations 24 hours after ceasing irrigation. The delayed harvest (LH) corresponds to lettuce samples collected from the farm locations 48 hours after ceasing irrigation. At the market, samples were collected before and after washing. LM_B refers to lettuce heads before being washed and before being displayed at the vendor table. LM_A refers to washed lettuce heads being displayed at the vendor’s table. Following this sampling procedure, the contamination in the value chain was studied because the lettuce samples collected at the market were from the selected farm locations.
Following the above-described sampling procedure, each site (GW1, GW2, RW3, and WW4) had corresponding water and lettuce samples WF, LF, LH, LM_A, LM_B, municipal water at the market (MWM), municipal water after washing 10 lettuce heads at the market (MW_10), and municipal water after washing all lettuce heads at the market (MW_T), which were collected along the chain from farm to market. The samples from the different sites were compared after analysis.
Laboratory analysis
The collected lettuce samples were prepared for analysis by removing the outer leaves and core of the lettuce and washing them in distilled water (Bencardino et al., Citation2018). Approximately 20 g of lettuce were weighed and then washed in 500 ml distilled water (Bencardino et al., Citation2018), which involved placing each lettuce leaf in a beaker cup with distilled water, which was then agitated at least 10 times. The agitation facilitates the transference of E. coli present on the surface of the leaves to the water solution.
The United States Environmental Protection Agency (USEPA) guidelines were followed for all laboratory studies (USEPA, Citation2010). The following sample volumes were obtained: 0.001, 0.01, 0.1, 1, and 10 ml, and subsequently tested for E. coli concentration; sample size depended on the bacterial concentration. If the sample volume was less than 10 ml, 50 ml sterile buffered dilution water was added to the filter funnel before applying the vacuum. Filter sterility was checked by placing one membrane filter per group of filters on a chromocult plate, after which it was incubated for 24 ± 2 hours at 35°C ± 0.5°C. The absence of growth indicated the sterility of the filter. The samples were filtered using 0.45-μm membrane filters, which were subsequently placed onto chromocult coliform agar plates (media from Merck, Darmstadt, Germany) and incubated for 24 hours at 35–37°C. Plates were checked for growth, and presumptive E. coli (blue) colonies were considered positives for E. coli; approximately 20–200 colonies were counted for each filter. Any counting outside this range was ignored in the calculations (Jensen et al., Citation2013).
Analytical procedures
The number of E. coli colonies was determined according to EquationEquations 1(1)
(1) and Equation2
(2)
(2) in water and lettuce samples, respectively. EC is the concentration of E. coli (CFU/100 mL) in water, V the volume of water used to wash the lettuce (ml), and Wg the weight of lettuce samples washed in distilled water (g). A factor of 100 was used to convert E. coli counts to colony-forming units (CFU)/100 ml and CFU/100 g for water and lettuce samples, respectively.
Data analysis
The data for E. coli concentration in water and lettuce have been analysed using the Statistical Package for the Social Sciences (SPSS) by one-way analysis of variance (ANOVA). The E. coli concentrations in this experiment were normalized by log10 transformations and then used ANOVA for multiple mean comparisons; when significant differences in the group were found, post-hoc evaluation by Tukey Honestly Significant Differences test was employed.
Results
Lettuce production and market handling
In Infulene Valley, farmers follow specific practices for irrigation, manure application, harvesting, transportation, and market handling. The irrigation sources used in the study were groundwater (GW1 and GW2), river water (RW3), and partially treated wastewater (WW4). Farmers used irrigation cans to water their lettuce crops by fetching water from nearby sources located 5–10 m away. They typically carry about 20 l of water per trip. During the dry period, irrigation is carried out twice per day to ensure adequate moisture for the crops. However, one day before harvest, irrigation is stopped to reduce the weight of the lettuce heads so that transportation to the markets is easier. Occasional rain events occurred during the first 2 months of the experimental period, and only one rain event (which occurred in the first week) was recorded during sampling. Manure is applied to the lettuce crops approximately 2 weeks after planting, at least once per cropping period. The application of manure provides essential nutrients to the plants, supporting their growth and development.
Lettuce is typically harvested in the morning. Farmers usually arrive at the field between 4.00am and 5.30am to cut and organize the lettuce heads. This early harvesting ensures that the lettuce is fresh when it reaches the market, maintaining its quality and extending its shelf life. After harvesting, the lettuce heads are packed in sacks for transportation. It should be mentioned that transportation is done in open cars where various market products are mixed. It can be hypothesized that this transportation method presents a potential risk as it can expose the lettuce to external contaminants or physical damage during transit. Upon arrival at the market, the vendors collected municipal tapwater in a bucket and used it to wash the lettuce, removing any residuals from the farm. The lettuce heads undergo screening and washing processes before being displayed for sale. Screening helps remove damaged or low-quality lettuce heads and ensures that only the best ones are available to customers. Washing the lettuce helps to eliminate dirt and surface contaminants, which enhances its visual appeal and cleanliness.
Concentration of Escherichia coli in water
The lettuce was followed from the different farm plots to the market. shows the E. coli concentration in water samples collected at (1) water at the farms (WF), (2) municipal water at the market (MWM), (3) municipal water after washing 10 lettuce heads at the market (MW_10), and (4) municipal water after washing all lettuce heads at the market (MW_T). The results show that all water samples exceeded the WHO 1989 guidelines of 3 log unit CFU/100 ml for irrigation water, the except municipal tapwater samples used to wash the lettuce heads (MWM samples), which showed E. coli concentrations of less than 1 log unit (). The municipal tapwater used for washing is also used for consumption and sourced from the drinking water mains.
Figure 2. Log concentrations of Escherichia coli in water samples (CFU/100 ml) in water at farms (WF), municipal water at the market (MWM), municipal water after washing 10 lettuce heads (MW_10), municipal water in the market after washing all (about 120–150) lettuce heads (MW_T).

Escherichia coli concentrations in MW_10 ranged between 2.5 and 4 log units at the market, whereas for WF and MW_T, the concentrations ranged between 3 and 6 log units. A comparison of sources and wash water (WF, MW_10 and MW_T) showed that the E. coli concentration in MW_10 was lower than in WF and MW_T at all sampling locations. The results indicate that washing the lettuce heads with MWM transferred E. coli from the lettuce heads to the washing water. Consecutive washing of multiple lettuce heads in the used municipal water significantly declined the water quality, leading to contamination of the subsequently washed lettuce. The results of MW_T and WF showed that the E. coli concentration varied among the sampling sites. Results in clearly show that for the water quality related to both groundwater sites (GW1 and GW2), the quality of MW_T is worse than that of WF. However, for the wastewater site, MW_T showed an approximately 1 log unit reduction compared to WF, whereas, for the river water site, the E. coli concentration at the market site (MW_T) was similar to the farm levels (WF). Our data show that for the wastewater-irrigated site, E. coli concentrations were highest. At the same time, lettuce washing resulted in a transfer of E. coli from the lettuce to the washing water. Notably, E. coli concentrations in the wash water (MW_10, MW_T) of the WW site were higher but consistently lower than those found at the farm level (WF). The lower E. coli concentration can be attributed to the high quality of the municipal tapwater (<1 log unit E. coli) used for washing at the market site.
Overall, washing the lettuce crops did not reduce the contamination when all lettuce heads were washed in the same water. Our findings show that other means of contamination, such as market practices, may affect the product’s quality. The water quality reduced after repeated use of the same water for washing, reflected in higher E. coli concentrations in MW_T compared to MW_10 ().
Table 2. Log E. coli concentrations (mean and P values) of water (CFU/100 ml) in the farm (WF), of municipal water in the market (MWM), of municipal water after washing 10 heads (MW_10), and of municipal water after washing all lettuce (MW_T). For each factor, are significant when P < 0.05.
Statistical analysis shows a significant difference between the different water qualities at the various farm sites (). The results reveal two groups with significant similarities in water quality at the farm. The first group, consisting of sites RW3 and WW4, was characterized by a higher E. coli concentration than the second group (GW1 and GW2). The results show no significant differences in irrigating with river water compared to partially treated wastewater. Moreover, comparing E. coli concentrations in the different market water samples revealed differences between samples from MWM and MW_10.
Escherichia coli concentration on lettuce
presents E. coli concentrations on lettuce at the four irrigated agricultural sites in Infulene Valley. Following a similar approach to the water samples for each irrigation site, the lettuce samples were collected at: (1) lettuce farms (LF) after 24 hours following the cessation of irrigation (normal harvest period), (2) lettuce at the market before lettuce washing (LM_B), and (3) lettuce in the market after washing (LM_A) all lettuce heads.
Table 3. Log E. coli concentrations (means and P values) on lettuce (CFU/100 g) from the farm (LF), lettuce after delayed harvest (LH) at the farms, lettuce at the market before washing (LM_B), and lettuce at market after washing (LM_A).
The cultivation of lettuce in the Infulene Valley takes place using irrigation water of uncontrolled water quality and using manure as a nutrient source. These practices may contribute to E. coli contamination of the irrigation water and exposure of the lettuce. In addition, farmers harvested the lettuce by using a knife to cut and detach it from the roots, which helped reduce contamination risks from the soil as transport of soil particles was prevented. Following this procedure, lettuce was collected and piled in sacks that were transported in a car with other vegetables produced in the valley. The lettuce was not handled differently from other crops during transportation to the market. Potentially, an increase in E. coli contamination might have occurred during transport because of cross-contamination from other vegetables.
Overall, the E. coli concentration on lettuce varied from 6 to 8 log units/100 g among the different irrigation water sources. This range exceeded the 5 log units CFU/100 g in all samples, referring to the threshold value recommended by the International Commission on Microbiological Specifications for Foods (ICMSF; Blumenthal et al., Citation2000). Independently of the sample location in the value chain, GW2 showed a higher E. coli concentration on the lettuce than other irrigation water sources (). Escherichia coli on lettuce irrigated with groundwater signalized contamination within the value chain. It showed that other factors might influence the quality of the lettuce produced in Infulene Valley than only irrigation water quality, such as manure application and crop handling. Manure is applied around the plant during the lettuce production cycle, and farmers apply it at least once during this stage. The manure is a mixture of animal excreta, coming from, for example, cows or chickens and mixed with plant residues. Although not tested in this study, the manure might have been a potential source of E. coli contamination of the lettuce on the farm, apart from the water sources.
The results in show that E. coli concentrations in LM_B samples were higher than in the LF samples irrigated with WW4 and GW2. However, for lettuce irrigated with GW1 and RW3, the E. coli concentrations were approximately at the same level. The lettuce from WW4 and GW2 were sold at the same market, and the handling practices were like the other markets. Comparison of the LM_B and LM_A samples shows that washing lettuce at the market reduced E. coli concentrations by 1 log unit at all sites except the RW3 site, which showed similar concentrations. Traoré et al. (Citation2020) also found that washing lettuce with fresh tapwater reduced E. coli concentrations. They found similar results when washing for 15 minutes compared with applying chemical disinfectants. Hence, the results show that washing lettuce at the market can contribute to risk reduction if done appropriately, as it is necessary to refresh the washing water regularly. Possibly, the decrease in E. coli concentration would have been significant when a reduced number of lettuce heads were washed in approximately 20 l of clean water. This volume was determined based on the storage capacity of the buckets that the vendor used to wash the lettuce in the market. However, this procedure is not followed at the market sites and was not part of our study.
presents E. coli concentrations on lettuce at harvest (LF) and delayed harvest (LH). Stopping irrigation is not a practice in any site of Infulene Valley. Farmers usually rely only on irrigating fields that are about to be harvested the next day. For this study, samples were collected one day after the usual harvest day. Results showed that the reduction in E. coli concentration on the lettuce grown at the different sites varied between 0.25 and 1.1 log unit per day. At least 1 log unit E. coli concentration reduction was observed for the GW sites, and less than 0.5 log unit reduction was observed in the RW3 and WW4 sites.
Statistical analysis showed similar E. coli concentrations on the grown lettuce, considering the different irrigation water sources (). The washing procedures at the market and late harvest did not significantly contribute to reduce the E. coli content on the lettuce. In addition, all produced lettuce, irrespective of the irrigation water sources used, exceeded the limits established by ICMSF for E. coli concentrations allowed for human consumption.
Discussion
In the Infulene Valley, farmers use groundwater, river water, and partially treated wastewater (depending on the location of their farm) as irrigation sources for lettuce production. All irrigation water sources had elevated levels of E. coli at varying levels, which indicated faecal contamination. Water sources were possibly contaminated by the indiscriminate dumping of garbage, discharge of farm effluents, partially treated water from WWTP plants, and or overflows from onsite sanitation systems into the river. Also, the irrigated lettuce was contaminated regardless of the irrigation water source. The results indicate that all water sources used for irrigation in the Infulene Valley were a potential source of faecal contamination. While irrigation water was not the only source of contamination, improving the quality of irrigation water alone may be insufficient to ensure an acceptable level of contamination of the crops. At the farms, groundwater sources showed less contamination (i.e., less than 2 log units of E. coli) than river and partially treated wastewater sources. Faecal contamination in groundwater may be due to manure application for nutrient supply and the lack of sanitary infrastructure in the area.
Similar studies have been carried out in other countries. The Faisalabad city region in Pakistan is characterized by agricultural practices similar to Infulene Valley regarding the use of wastewater for irrigation. There, smallholder farmers irrigate crops (including vegetables) with wastewater from the city (Ensink et al., Citation2005). A study carried out in Faisalabad showed high levels of E. coli concentrations in wastewater and partially treated wastewater as expected but low crop contamination levels. Compared with studies carried out in Ghana, Mexico, and Israel, the authors concluded that lower contamination levels could be attributed to the applied irrigation method (Ensink et al., Citation2007). In Faisalabad, the irrigation water was conveyed via furrows with minimal contact with the produce, whereas in other countries, sprinklers or watering cans increased the potential for contamination. Maputo’s case may reflect the conditions in countries like Ghana, where irrigation was carried out using watering cans. The present findings have implications for farmers irrigating with contaminated water. The use of contaminated water could affect their health, leading to various diseases, such as diarrhoea and skin diseases (Mengesha et al., Citation2021). Also, Amoah et al. (Citation2005) suggest that vectors for water-borne diseases can breed in these water sources and should be carefully considered. The public health concerns highlight the importance of addressing the issue of pollution and ensuring the availability of clean and safe water for irrigation purposes.
At the market, the E. coli concentration on lettuce before washing (M_B) was higher than the E. coli concentration on lettuce at the farm (LF), except for the RW3 and GW1 sources. This suggests that contamination happens during the handling and transport of lettuce, highlighting the need for improved measures along the value chain to reduce consumers’ health risks. Such measures may also include adopting protective measures during crop handling at the farm (Caponigro et al., Citation2010). The current production and handling practices in Infulene Valley present potential risks for E. coli contamination, which can subsequently affect the water and the vegetables produced. In addition, the harvesting process, where lettuce heads are cut from the roots using a knife, followed by piling them in sacks for transportation, may pose a risk for cross-contamination. If any initial contamination of the lettuce or other vegetables occurred at the market, the lack of handling or separation during transportation could contribute to the spread of contaminants, including E. coli, at the marketplace (Amoah et al., Citation2005). Escherichia coli can reproduce under favourable non-host conditions, such as those in humid tropical areas like Maputo. The resulting natural bacterial growth can increase the total E. coli concentration on lettuce along the value chain (Keraita et al., Citation2007). The outside temperatures, prevailing humidity, and transportation methods in cities like Maputo enhance the growth of bacteria and demand a critical look at the agricultural value chain. Studies in low- and middle-income countries show that unsanitary conditions contribute to increased levels of bacterial contamination (Winfield & Groisman, Citation2003).
If appropriately managed, practices along the value chain, such as washing the produce and strategically delaying the harvest, can contribute to risk reduction. For example, proper washing procedures must consider the proportion of lettuce versus water and the duration of the washing. However, the results indicate that the current implementation of washing and refreshing in the market can also introduce contamination. The primary reason is that washing several lettuce heads in the same water deteriorates the quality of the washing water and, therefore, results in possible cross-contamination. These findings indicate that lettuce heads are contaminated if the washing water is not regularly refreshed, resulting in cross-contamination of the produce (Dao et al., Citation2018). Similar research conducted in other developing countries, such as Ghana and Pakistan, describes that market vendors even use water of much lower quality for lettuce refreshments than observed in our present work (Ensink et al., Citation2007; Keraita & Drechsel, Citation2009). Nonetheless, the washing procedure using fresh water decreased the E. coli concentrations on lettuce at the marketplace. This indicates the washing practice’s potential to contribute to reducing health risks (Amoah et al., Citation2007). A similar finding was observed in Ouagadougou, Burkina Faso, where clean water was used for post-harvest washing of lettuce. The washing procedure was optimized by adding additives such as a chlorine solution to the wash water (Dao et al., Citation2018). Results from our present research suggest the need to adopt additional measures, like regular refreshment of the washing water, to reduce E. coli concentrations.
In addition to proper handling methods at the market, a delay in harvest may contribute to risk reduction to acceptable levels for consumption (). Post-irrigation and pre-harvest periods contribute to the deactivation of E. coli by sunlight (Dao et al., Citation2018; Keraita et al., Citation2008). The observed E. coli reduction was within the ranges suggested by WHO, indicating a range of 0.5–2 log units per day for E. coli die-off (Keraita et al., Citation2008) for all water sources except for partially treated wastewater. For partially treated wastewater, results showed an E. coli reduction of 0.25 log units per day, which is below the averages indicated by the WHO. Our results are possibly influenced by the time the samples were collected, which coincided with the transition from the wet to the dry season when rain events occur and solar radiation is not optimal. In fact, the cessation of irrigation practice is ineffective in reducing contamination during the wet season due to the recontamination of vegetables as result of splashes from soils, and wet conditions generally allow longer pathogen survival (Keraita et al., Citation2007). Therefore, late harvest only contributes to risk reduction in dry and sunny seasons. Keraita et al. (Citation2008) recommended longer periods of, for example, 4 days for delayed harvest compared to the 2 days used here to achieve improved risk reduction. However, this methodology is not feasible for lettuce, as a longer cessation of irrigation will negatively impact the lettuce’s quality and result in a loss of profit for farmers (Drechsel & Karg, Citation2013). Hence, further research is needed to optimize the pre-harvest period that will reduce the E. coli concentrations in Maputo’s local (climatological) conditions.
The importance of hazard identification in the critical contamination pathway and awareness raising
Our research results on irrigation and wash water reveal potential health risks along the agricultural value chain and also indicate that post-harvest practices could help in risk reduction. While assessing the indicator of hazardous condition such as E. coli, it is important to identify the critical control points for intervention. Our findings emphasize the need for complementary actions to reduce contamination along the value chain. Safeguarding irrigation water quality will not be sufficient to control the risks along the value chain for lettuce production in Infulene Valley. At the farm level, agricultural practices need to be amended to irrigation water quality, while current market practices regarding produce washing did not improve the produce quality. Like other sub-Saharan countries, Mozambique faces challenges to adopt the updated WHO guidelines and is still using the 1989 WHO guidelines for wastewater irrigation practices adopted by countries with unplanned use of wastewater (Drechsel & Qadir, Citation2022). The updated 2006 guidelines have not yet been implemented due to the perceived notion that they are complex and impractical, given the lack of infrastructure, and capacity within the country. Thus, the results of this study were assessed using the WHO 1989 guidelines. Nonetheless, our findings may change the perception of irrigation water quality and produce safety and may influence the stakeholders’ role in guaranteeing produce safety along the value chain.
Possible interventions to improve the policy recommendations
The findings of this study suggest that contamination risks can only be addressed effectively with a holistic risk management approach, which includes considering the entire value chain and adopting a multiple barrier approach (Abaidoo et al., Citation2010; Niquice Janeiro et al., Citation2020). Such an approach considers and manages risks at each step of the chain, including the irrigation source, production practices, transport, market handling, and consumers’ habits. Required measures may include implementing appropriate treatment technologies to treat irrigation water before it is used in agricultural practices. Technologies of interest may involve filtration systems, disinfection methods, or other advanced treatment processes to remove contaminants and pathogens. In addition, adopting improved irrigation methods may also help to reduce crop contamination. These methods may include drip irrigation or other precision irrigation techniques that minimize direct contact between water and plants, reducing the risk of contamination (Abaidoo et al., Citation2010).
Another management strategy of interest is promoting self-awareness among farmers and vendors at the market. By offering education and training programmes to farmers, handlers, vendors, and other stakeholders involved in the production and distribution chain, awareness is raised about good agricultural practices, proper handling, and washing. Such provision will enable the different stakeholders to make informed decisions, minimize contamination risks, and enable the implementation of a multibarrier approach and HACCPs in the chain. Further, regulatory agencies or institutions should establish monitoring programmes to assess water and produce quality at the source, irrigated agricultural plots, and marketplaces. Regulatory institutions are mandated to check compliance with relevant regulations, guidelines, and standards pertaining to water quality and food safety. This involves adhering to local, regional, and national regulations concerning irrigation practices and quality control measures.
By implementing the above measures, it should be possible to mitigate contamination risks, help and protect farmers and consumers, and ensure the production of safe and high-quality agricultural products. Particularly, collaboration between stakeholders, including farmers, vendors at the market, water authorities, and relevant agencies is pivotal to implement and sustain these measures successfully.
Conclusions
This study assessed faecal contamination of lettuce produced at farms and sold at local markets of Maputo, Mozambique. The E. coli concentration served as an indicator for faecal contamination in both water and lettuce samples. Our results show that, regardless of the water source used for irrigation, all irrigation water sources in Infulene Valley are contaminated and the lettuce was contaminated throughout the entire value chain. The average E. coli concentrations in irrigation water were 3.4–3.8, 5.5, and 5.7 log units/100 ml for groundwater (GW1 and GW2), river water (RW3), and partially treated wastewater (WW4), respectively. The E. coli concentration on lettuce was 7.1–7.8, 7.1 and 6.6 log units/100 g for the groundwater, river water, and partially treated wastewater irrigated lettuce, respectively. We also showed that washing lettuce at the marketplace has little effect on risk reduction and a high likelihood of recontamination of the produce. This requires actions to be adopted at the market in order to reduce contamination, such as reducing the number of lettuce heads being washed with the same water.
The results clearly show that the lettuce sold in the market might be contaminated by handling practices. Apparently, irrigation water is not the only potential source of contamination in the value chain. Improved hygiene at the marketplace will significantly impact the contamination levels of the lettuce, irrespective of the use of reclaimed wastewater or other water sources for irrigation in Maputo. In addition, we found that delayed harvest contributes to risk reduction. At least 1 log unit E. coli concentration reduction was observed for the GW sites, and less than 0.5 log unit reduction was observed in the RW3 and WW4 sites. Delayed harvest may compromise product quality due to dehydration problems, reducing the value of the produce.
To date, the government is still adopting the 1989 WHO guidelines. This work can pave the way to plan a multibarrier approach for Maputo, as the 2006 WHO guidelines suggest. The establishment of risk reduction interventions with different stakeholders could be applied at several steps along the value chain, i.e., from irrigation water to farm practices, crop handling, transportation, and vending at the market, to reduce the risks of E. coli contamination while preserving the livelihood of the (peri-)urban farmers. Also, awareness activities involving farmers, vendors, and municipal officials of Infulene Valley are required to safeguard crop contamination levels in the value chain. We recommended that future studies address viable solutions along the value chain to reduce the current contamination risks.
Acknowledgements
The authors acknowledge the Netherlands Organization for Scientific Research (NWO) for funding this research. We also would like to acknowledge the anonymous reviewers who revised the manuscript which improved its quality.
Disclosure statement
No potential conflict of interest was reported by the author(s).
Additional information
Funding
Notes
1. It should be mentioned that since the second half of 2023, WWTP maintenance has improved substantially with cleaning and overhauling the pond area.
References
- Abaidoo, R. C., Keraita, B., Drechsel, P., Dissanayake, P., & Maxwell, A. S. (2010). Soil and crop contamination through wastewater irrigation and options for risk reduction in developing countries. In P. Dion (Ed.), Soil biology and agriculture in the tropics (vol. 21, pp. 275–297). Springer. https://doi.org/10.1007/978-3-642-05076-3_13
- Amoah, P., Drechsel, P., & Abaidoo, R. C. (2005). Irrigated urban vegetable production in Ghana: Sources of pathogen contamination and health risk elimination. Irrigation and Drainage: The Journal of the International Commission on Irrigation and Drainage, 54(S 1), S49–S61. https://doi.org/10.1002/ird.185
- Amoah, P., Drechsel, P., Abaidoo, R. C., & Klutse, A. (2007). Effectiveness of common and improved sanitary washing methods in selected cities of West Africa for the reduction of coliform bacteria and helminth eggs on vegetables. Tropical Medicine and International Health, 12(Suppl. 2), 40–50. https://doi.org/10.1111/j.1365-3156.2007.01940.x
- Arsénio, A. M., Salim, I. C., Hu, M., Matsinhe, N. P., Scheidegger, R., & Rietveld, L. (2018). Mitigation potential of sanitation infrastructure on groundwater contamination by nitrate in Maputo. Sustainability, 10(3), 1–18. https://doi.org/10.3390/su10030858
- Batran, M., Arai, A., Kanasugi, H., Cumbane, S., Grachane, C., Sekimoto, Y., & Shibasaki, R. (2018, July 11–14). Urban travel time estimation in greater maputo using mobile phone big data. In 2018 IEEE 20th Conference on Business Informatics (CBI),(Vol.2, pp.122–127). IEE. https://doi.org/10.1109/CBI.2018.10057
- Bencardino, D., Vitali, L. A., & Petrelli, D. (2018). Microbiological evaluation of ready-to-eat iceberg lettuce during shelf-life and effectiveness of household washing methods. Italian Journal of Food Safety, 7(1). https://doi.org/10.4081/ijfs.2018.6913
- Blumenthal, U. J., Mara, D. D., Peasey, A., Ruiz-Palacios, G., & Stott, R. (2000). Guidelines for the microbiological quality of treated wastewater used in agriculture: Recommendations for revising WHO guidelines. Bulletin of the World Health Organization, 78(9), 1104–1116.
- Caponigro, V., Ventura, M., Chiancone, I., Amato, L., Parente, E., & Piro, F. (2010). Variation of microbial load and visual quality of ready-to-eat salads by vegetable type, season, processor and retailer. Food Microbiology, 27(8), 1071–1077. https://doi.org/10.1016/j.fm.2010.07.011
- Dao, J., Stenchly, K., Traore, O., Amoah, P., & Buerkert, A. (2018). Effects of water quality and post-harvest handling on microbiological contamination of lettuce at urban and peri-urban locations of Ouagadougou, Burkina Faso. Foods, 206(7). https://doi.org/10.3390/foods7120206
- Drechsel, P., & Karg, H. (2013). Motivating behaviour change for safe wastewater irrigation in urban and peri-urban Ghana. Sustainable Sanitation Practice, 16, 10–20. http://www.ecosan.at/ssp/issue-16-behaviour-change/SSP-16_Jul2013_10-20.pdf
- Drechsel, P., Keraita, B., Amoah, P., Abaidoo, R. C., Raschid-Sally, L., & Bahri, A. (2008). Reducing health risks from wastewater use in urban and peri-urban sub-Saharan Africa: Applying the 2006 WHO guidelines. Water Science & Technology, 57(9), 1461–1466. https://doi.org/10.2166/wst.2008.245
- Drechsel, P., & Qadir, M. (2022). The WHO guidelines for safe wastewater use in agriculture: A review of implementation challenges and possible solutions in the global south. Water, 14(6), 864. https://doi.org/10.3390/w14060864
- Edberg, S. C., Rice, E. W., Karlin, R. J., & Allen, M. J. (2000). Escherichia coli: The best biological drinking water indicator for public health protection. Journal of Applied Microbiology, 88(S1), 106S–116S. https://doi.org/10.1111/j.1365-2672.2000.tb05338.x
- Ensink, J. H., van der Hoek, W., Mukhtar, M., Tahir, Z., & Amerasinghe, F. P. (2005). High risk of hookworm infection among wastewater farmers in Pakistan. Transactions of the Royal Society of Tropical Medicine and Hygiene, 99(11), 809–818. https://doi.org/10.1016/j.trstmh.2005.01.005
- Ensink, J. H. J., Mahmood, T., & Dalsgaard, A. (2007). Wastewater-irrigated vegetables: Market handling versus irrigation water quality. Tropical Medicine and International Health, 12(S 2), 2–7. https://doi.org/10.1111/j.1365-3156.2007.01935.x
- Halder, S., Agüero, J., Dolle, P., Fernández, E., Schmidt, C., & Yang, M. (2018). Perspectives of Urban Agriculture in Maputo and Cape Town. Humboldt-Universitat zu.
- Hamilton, A. J., Stagnitti, F., Premier, R., Boland, A. M., & Hale, G. (2006). Quantitative microbial risk assessment models for consumption of raw vegetables irrigated with reclaimed water. Applied and Environmental Microbiology, 72(5), 3284–3290. https://doi.org/10.1128/AEM.72.5.3284-3290.2006
- Jensen, A. N., Storm, C., Forslund, A. L., Baggesen, D., & Dalsgaard, A. (2013). Escherichia coli contamination of lettuce grown in soils amended with animal slurry. Journal of Food Protection, 76(7), 1137–1144. https://doi.org/10.4315/0362-028X.JFP-13-011
- Keraita, B., Jimenez, B., & Drechsel, P. (2008). Extent and implications of agricultural reuse of untreated, partly treated and diluted wastewater in developing countries. CABI Reviews, (2008), 15. https://doi.org/10.1079/PAVSNNR20083058
- Keraita, B., Konradsen, F., Drechsel, P., & Abaidoo, R. C. (2007). Reducing microbial contamination on wastewater-irrigated lettuce by cessation of irrigation before harvesting. Tropical Medicine and International Health, 12(S 2), 8–14. https://doi.org/10.1111/j.1365-3156.2007.01936.x
- Keraita, B. N., & Drechsel, P. (2009). Agricultural use of untreated urban wastewater in Ghana. In C. A. Scott, N. I. Faruqui, & L. Raschid-Sally (Eds.),Wastewater use in irrigated agriculture: Confronting the livelihood and environmental realities (pp. 101–112). CABI Publishing. https://doi.org/10.1079/9780851998237.0101
- Matangue, M. T. A., Saize, H. T. D., & de Sousa, L. S. (2018). Quantitative microbial risk assessment arising from consumption of salad crops irrigated with contaminated water by human sewage in the infulene valley. In Recent Advances in Environmental Science from the Euro-Mediterranean and Surrounding Regions: Proceedings of Euro-Mediterranean Conference for Environmental Integration (EMCEI-1), Tunisia 2017 (pp. 585–587). Springer International Publishing.
- Mengesha, S. D., Kidane, A. W., & Dinssa, D. A. (2021). Microbial risk assessment of vegetables irrigated with akaki river water in Addis Ababa. Research Square, 1–22. https://doi.org/10.21203/rs.3.rs-492022/v1
- Nansubuga, I., Banadda, N., Verstraete, W., & Rabaey, K. (2016). A review of sustainable sanitation systems in Africa. Reviews in Environmental Science and Biotechnology, 15(3), 465–478. https://doi.org/10.1007/s11157-016-9400-3
- Niquice Janeiro, C. A., Arsénio, A. M., Brito, R. M. C. L., & van Lier, J. B. (2020). Use of (partially) treated municipal wastewater in irrigated agriculture; potentials and constraints for sub-Saharan Africa. Physics and Chemistry of the Earth, Parts A/B/C, 119. https://doi.org/10.1016/j.pce.2020.102906
- Orsini, F., Kahane, R., Nono-Womdim, R., & Gianquinto, G. (2013). Urban agriculture in the developing world: A review. Agronomy for Sustainable Development, 33(4), 695–720. https://doi.org/10.1007/s13593-013-0143-z
- Rietveld, L. C., Siri, J. G., Chakravarty, I., Arsénio, A. M., Biswas, R., & Chatterjee, A. (2016). Improving health in cities through systems approaches for urban water management. Environmental Health, 15(1), 151–160. https://doi.org/10.1186/s12940-016-0107-2
- Salamandane, C., Fonseca, F., Afonso, S., & Lobo, M. L. (2020). Handling of fresh vegetables: Knowledge, hygienic behavior of vendors, public health in Maputo markets, Mozambique. International Journal of Environmental Research & Public Health, 17(17), 6302. https://doi.org/10.3390/ijerph17176302
- Salvador, E., Chaúque, A., Irá, T., & Monteiro, L. (2016). Potential risk for spread multidrug resistant Enterobacteriaceae through Lactuca sativa (Lettuce) and Allium fistulosum L. (Welsh onion) from Infulene Valley, Maputo city, Mozambique. Journal of Applied and Environmental Microbiology, 4(1), 21–24. https://doi.org/10.12691/jaem-4-1-2
- Scarlet, M. P., & Bandeira, S. (2014). Pollution in Maputo Bay. In S. Bandeira & J. Paula (Eds.),The Maputo Bay ecosystem. WIOMSA, Zanzibar Town (Vol. 247). https://docplayer.net/55319400-The-maputo-bay-ecosystem-editors-salomao-bandeira-jose-paula.html
- Scheierling, S. M., Bartone, C. R., Mara, D. D., & Drechsel, P. (2011). Towards an agenda for improving wastewater use in agriculture. Water International, 36(4), 420–440. https://doi.org/10.1080/02508060.2011.594527
- Sitoe, P. R., & Mina Pinto, I. M. A. C. (2019). Gestão de Efluentes Municipais da ETAR da Cidade de Maputo e Arredores: Situação actual, impactos e desafios. Scientific Journal of Mathematics, Natural and Applied Sciences, 3(1), 22–29.
- Smart, J. C., Cachomba, I. S., & Tschirley, D. (2016). Input use, input purchase channels and other general characteristics of the Horticultural producers of Maputo. Special Issue Collection of Short Papers Focused on Horticultural Production in the Areas Supplying Maputo, 18–30. https://www.researchgate.net/profile/Tomas-Sitoe/publication/312298727_The_Effect_of_Horticultural_Diversification_on_Income_Evidence_from_Small_Producers_in_the_Green_Belt_of_Maputo/links/5878e91f08ae9275d4d90160/The-Effect-of-Horticultural-Diversification-on-Income-Evidence-from-Small-Producers-in-the-Green-Belt-of-Maputo.pdf
- Smart, J. C., Tschirley, D., & Cachomba, I. S. (2015). Perfil e indicadores socioeconómicos dos produtores de hortícolas. In F. V. Resende, L. L. Haber, C. E. Carvalho, & W. Bowen (Eds.), Horticultura em Moçambique: Caracteristicas, Tecnologias de Produção e de Pós-colheita (pp. 42–52). Embrapa.
- Sousa, I. M., Zucula, L., Nhancupe, N., Banze, L., Zacarias, B., & Noormahomed, E. V. (2021). Assessment of Parasitic Contamination of Lettuce and Cabbages Sold in Selected Markets in Maputo City, Mozambique. EC Microbiology, 17(6), 27.
- Taviani, E., Ceccarelli, D., Lazaro, N., Bani, S., Cappuccinelli, P., Colwell, R. R., & Colombo, M. M. (2008). Environmental Vibrio spp., isolated in Mozambique, contain a polymorphic group of integrative conjugative elements and. FEMS Immunology & Medical Microbiology, 64, 45–54. https://doi.org/10.1111/j.1574-6941.2008.00455.x
- Thebo, A. L., Drechsel, P., Lambin, E. F., & Nelson, K. L. (2017). A global, spatially-explicit assessment of irrigated croplands influenced by urban wastewater flows. Environmental Research Letters, 12(7). https://doi.org/10.1088/1748-9326/aa75d1
- Traoré, S., Cornelius, E. W., Samaké, F., Essilfie, G., Babana, A. H., Bengaly, M., & Koné, A. (2020). Efficiency of common washing treatments in reducing microbial levels on lettuce in Mali. West African Journal of Applied Ecology, 28(2), 64–74. https://www.ajol.info/index.php/wajae/article/view/202695
- Urbano, V. R., Mendonça, T. G., Bastos, R. G., & Souza, C. F. (2017). Effects of treated wastewater irrigation on soil properties and lettuce yield. Agricultural Water Management, 181, 108–115. https://doi.org/10.1016/j.agwat.2016.12.001
- USEPA. 2010. Method 1103.1: Escherichia coli (E. coli) in water by membrane filtration using membrane-thermotolerant Escherichia coli agar (mTEC). https://www.epa.gov/sites/default/files/2015-08/documents/method_1103-1_2010.pdf
- Veldwisch, G. J., Amerasinghe, P., Letema, S., & Wessels, M. T. (2024). The practices and politics of irrigated urban agriculture. Water International, 49(2), 129–143. https://doi.org/10.1080/02508060.2024.2325800.
- Whittinghill, L. J., & Rowe, D. B. (2012). The role of green roof technology in urban agriculture. Renewable Agriculture and Food Systems, 27(4), 314–322. https://doi.org/10.1017/S174217051100038X
- WHO (World Health Organization). (1989). Guidelines for the safe use of wastewater and excreta in agriculture and aquaculture. Food Contr. https://doi.org/10.1016/0956-7135(91)90194-2
- WHO (World Health Organization). (2006). Guidelines for the safe use of wastewater, excreta and greywater. Wastewater Use in Agriculture, 2. https://doi.org/10.1007/s13398-014-0173-7.2
- Winfield, M. D., & Groisman, E. A. (2003). Role of nonhost environments in the lifestyles of Salmonella and Escherichia coli. Applied and Environmental Microbiology, 69(7), 3687–3694. https://doi.org/10.1128/AEM.69.7.3687
- Woldetsadik, D., Drechsel, P., Keraita, B., Itanna, F., Erko, B., & Gebrekidan, H. (2017). Microbiological quality of lettuce (Lactuca sativa) irrigated with wastewater in Addis Ababa, Ethiopia and effect of green salads washing methods. International Journal of Food Contamination, 4(1). https://doi.org/10.1186/s40550-017-0048-8