ABSTRACT
Norbormide (NRB) is a Rattus-specific toxicant that has low palatability and produces sub-lethal dosing in the field, thereby limiting its effectiveness as a rodent control tool. In this study, we assessed the species-specific effect and time to death of a lethal dose of a fatty acid derived pro-toxicant of NRB, named DR8, in six rodent species from China; three species of Rattus and three non-Rattus species. Our results showed three species/subspecies of Rattus were sensitive to DR8, with LD50 values being less than 50 mg/kg, while non-Rattus rodent species were totally insensitive to this drug. As expected, the time to death in the three species of Rattus was longer in those rats dosed with DR8 compared to those dosed with NRB. This indicated that DR8 possesses a slower activity profile to NRB, and therefore supports its potential to increase palatability and be a promising species-specific tool for rodent pest management.
Introduction
As a member of the natural ecosystem in many parts of the world, including China, the rodent plays a key role in the food web with many rodent species making a significant contribution in maintaining the ecological balance (Kausrud et al. Citation2008). However, some rodent species are viewed as pests from a human perspective, causing huge losses to agricultural crops, stored food and posing high risk to the human health, food safety and the environment (Battersby et al. Citation2008). Most countries in the world suffer substantial rodent damage, this is especially true in tropical areas where rodents have a huge impact on agricultural production causing significant losses both during pre- and post-harvest stage (Singleton Citation2003; Singla and Parshad, Citation2010). The Food and Agriculture Organisation claims that rodents destroy more than 42 million tons of food throughout the world, with a cost estimate of around US$30 billion (Rennison et al. Citation2012). In China, rodent pest incursions occur in 20–40 million hectares of farmland, and cause 5–10% yield loss of agricultural crops annually (Guo et al. Citation2013). In addition to impacting on agricultural production, risk to human health is high through zoonosis and disease transmission. Rodents can transmit more than 35 types of disease to humans and/or animals such as bubonic plague, leptospirosis, Q fever and salmonella (Meerberg et al. Citation2009). Furthermore, rodents are recognised to be the most numerous of the world’s invasive vertebrate species and are responsible for major loss of biodiversity and native habitats, threatening both plant and animal species through predation and habitat destruction, and even resulting in many instances in species extinction (Atkinson Citation1985; Howald et al. Citation2007). In order to minimise these impacts, effective rodent management methodologies need to be developed and implemented responsibly, preferably at a landscape scale in order to prevent immigration from neighbouring areas and population rebound.
There are many methods available for rodent management, each has their advantages and disadvantages (Brown et al. Citation2017). Undoubtedly, lethal chemical agents remain the most effective tool for rodent pest management. There are a number of highly toxic compounds on the market that are widely used in controlling rodents but almost all are broad-spectrum toxicants that can kill non-target species (Eason et al. Citation2002; Ruizsuárez et al. Citation2014; Campbell et al. Citation2015). Since the 1940s, first-generation anticoagulants, zinc phosphide, cholecalciferol, bromethalin and second-generation anticoagulants were developed sequentially for rodent control. These have achieved varying degrees of success (Buckle and Smith Citation1994; Pelfrene Citation2001). The discovery of the anticoagulant rodenticides was, undoubtedly, the most important step ever made towards the introduction of effective rodent control. Even now second-generation anticoagulants are still the most effective products for rodent pest management because of their high toxicity and chronic mode of action (Buckle & Eason Citation2015) and in recent years have been the only ones available on the market for rodent pest management in China (data from China ministry of agriculture). However, with all of these broad-spectrum rodenticides there are some common disadvantages – particularly risks associated with non-target poisoning. Rodenticides have been responsible for numerous accidents involving humans, domestic and wild animals, through (a) direct poisoning via consumption of baits containing the compounds (Valchev et al. Citation2008) with rodenticide poisoning in people being ranked second in the number of pesticide-related poisonings recorded each year, (Bronstein et al. Citation2008), and (b) indirect poisoning through the ingestion of the dead or alive poisoned rodents (Valchev et al. Citation2008) as these toxicants are known to have long persistence times in live animals and carcasses. Indirect poisoning is particularly a high-risk issue to rodent predators, raptors and general scavengers. It has been estimated that a minimum of 11% of the sampled great horned owl population in Quebec, Canada is at risk of being killed by second-generation anticoagulants (Thomas et al. Citation2011). Not only are such predators key to maintaining the balance of ecosystem, many are endangered species (Howald et al. Citation1999). In addition, because many of the anticoagulants are very persistent, their use poses a potential significant risk to the environment through the accumulation of the compound in the food chain (Alomar et al. Citation2018). The development of specific and effective rodenticides for rodent management with particular emphasis on those that have minimal impact on non-target species is being actively explored by several research groups (Eason et al. Citation2017) and is a growing research area in light of the increasing restrictions being imposed on the use of second-generation anticoagulants (Alomar et al. Citation2018).
Norbormide (NRB), a highly selective toxicant to the genus Rattus, was developed in the 1960s (Rennison et al. Citation2013) to serve as a non-anticoagulant rat poison being selectively toxic to rats with low toxicity to other species (Roszkowski et al. Citation1964; Eason et al. Citation2017). This compound with such optimal characteristics should have been the perfect species-specific rodenticide, however, its acute lethality caused rats to associate the onset of uncomfortable symptoms with the ingestion of the poison bait, which resulted in many cases in sub-lethal doses being consumed by the rats (bait-shyness phenomenon) (Greaves et al. Citation1968; Kusano Citation1975). Combination of this bait-shyness with taste aversion caused poor field efficacy of this compound in rodent control operations (Eason et al. Citation2017) and as a consequence, NRB was quickly withdrawn from the rodenticide market. However, in recent years, a series of pro-toxicants (substances administered in a nontoxic form that is then metabolised in the body in vivo into toxicant) of NRB were developed as a means of overcoming these issues by delaying the onset of toxicity (Rennison et al. Citation2012, Citation2013; Jay-Smith et al. Citation2016). A NRB-derived pro-toxicant 3d (CAS no. 1383691-91-5), named DR8 (Choi et al. Citation2016), showed a significantly improved efficacy profile with the delay to onset of symptoms allowing lethal doses to be consumed in dwarf rats, Sprague–Dawley rats, wild Norway rats and wild ship rats (Choi et al. Citation2016). In this study, we assess the species-specific effect and the time to death using lethal doses of the pro-toxicant DR8 in key rodent pest species from China.
Materials and methods
Capture and maintenance of animals
We live captured six rodent species from China belonging to 6 species/subspecies of 4 genera. Rattus norvegicus norvegicus, Rattus losea and Bandicota indica were captured in the fields of Zhongshan county, Guangdong province, R.n.caraco was from the fields of Harbin, Heilongjiang province. The non-Rattus species, Lasiopodomys brandtii and Microtus fortis were taken from a breeding colony of wild-caught stock that was established one year before this study commenced. The progenitors of L.brandtii were trapped from Xilinhot of Inner Mongolia and M.fortis was obtained from Yueyang county, Hunan province. In all experiments, the animals were individually housed in indoor laboratory facilities, in 535 × 390 × 200 mm plastic cages with a wire mesh cover for R.n.norvegicus, R.n.caraco, R.losea and B.indica, and 290 × 180 × 160 mm plastic cages for M.fortis and L.brandtii. Food and water were made freely available, with bedding/nesting substrate(s) provided. Natural illumination and temperature of the local province the rats originated from, were used in the laboratory. The wild-caught animals were acclimatised for a period of one week before being used in trials. Body weights of each wild animal were made on day 1 and then on every alternate day until the start of the experimental treatment. General body condition of the animals was assessed daily through monitoring of food and water intake. Individuals whose body weight fell >25% from their starter weight were omitted. Lastly, only healthy and mature rodents were grouped for the experimental trials.
Administration of DR8
A sample of pure grade DR8 power was supplied by Landcare Research, New Zealand. The compound was dissolved to a final concentrate of 10 mg/mL in a solution of 5% DMSO and 0.2M HCL this being the maximum concentration that could be achieved with this compound. The solution was made up not more than 2 hours before oral gavage and was kept at 0°C until animals were ready to be orally gavaged (a technique where liquid compounds are administered directly into the stomach of rats).
Adult rodents of the 3 species of Rattus, were randomly divided into 5–6 groups (each of 6 animals) with equal males and females per group. There was no significant difference in the body weight between the groups (R.losea: F(5,30) = 0.477, p = .790; R.n.norvegicus: F(5,30) = 1.250, p = .311; R.n.caraco: F(6,35) = 0.591, p = .735). For each species, one group of animals was administrated an initial trial dose of 100 mg/kg body weight of DR8 solution. If less than half animals died at this dose, we determined that an accurate estimation of the Mean Lethal Dose (LD50) was not feasible as higher volumes could not ethically be delivered at this concentration, and higher concentrations were difficult to attain due to low solubility of this compound formulation (Choi et al. Citation2016). As a consequence no further testing was attempted for these species. If more than half the number died at 100 mg/kg dose, the other groups were dosed at 50, 30, 20 and 10 mg/kg consecutively but dependent upon number of deaths occurring. In the case of R.n.caraco, because 5/6 died at ≧20 mg/kg dose, we tested a further 6 animals at 15 mg/kg in order to obtain a reasonable curve and estimate of the LD50 value. Of those species not belonging to Rattus, we selected two groups (each of 6 animals with equal male: female ratio) for each species that showed no significant difference in their body weights (B.indica: F(1,10) = 0.759, p = .404; L.brandtii: F(1,10) = 2.616, p = .137; M.fortis: F(1,10) = 0.003, p = .959). One group (the treatment group) was orally gavaged with a volume that equated to 200 mg/kg body weight of DR8 suspension. The control group was orally gavaged with solvent (5% DMSO/0.2M HCL lacking DR8).
We removed the food (but not water) from cages the night before the treatment so animals had an empty stomach on the day of the experimentation. Every animal was lightly anaesthetised using ether, before administrating a defined dose of DR8 solution, or corresponding volume of the solvent depending on their body weight. The animals were individually housed in indoor laboratory facilities and food and water were made freely available after the different treatments. We recorded numbers dying and time to death for each species for 7 days after treatment with the different DR8 dosing regimens, and observed poison symptoms induced by the compound. All animal manipulation was approved by the Animal Care & Welfare Committee of China Agricultural University (CAU20160823–3).
Statistical analyses
For those species sensitive to DR8, we used a chi-square test of independence to compare average mortality across all dose groups (to R.n.caraco, 15 mg/kg was exclusive), grouping males, females and across a third group containing both sexes. A one-way ANOVA was run comparing the average time to death between species at the doses that caused mortality≧50% to all sensitive species (30, 50 and 100 mg/kg), respectively. LD50 to DR8 was calculated in all species by the method of Miller and Tainter (Al-Ali et al. Citation2008). All data are expressed as the means ± S.E.M.; all statistical analyses were performed using the SigmaStat program (SPSS, Inc., Chicago). Any statistical differences were considered as significant and highly significant at p < .05 and p < .001, respectively.
Results
Mortality of different rodent species and species-specific effects
In all 6 species/sub species treated, no deaths were observed in the control groups during the treatment period. All non-Rattus species, B.indica, L.brandtii and M.fortis, survived the highest dose of 200 mg/kg DR8. In comparison, all Rattus, R.losea, R.n.norvegicus and R.n.caraco, were sensitive to DR8, but different mortalities were obtained at the different doses for each species (). No differences in average percentage mortality was observed in the sensitive species across all dose groups and across both combined and different sex groups (combined sexes (χ2 = 4.481, df = 8, p = .811), the females (χ2 = 2.488, df = 8, p = .962) and males (χ2 = 2.475, df = 6, p = .871)) (). Similarly, we found no difference in average percentage mortality when comparing male to female for each species (R.losea: χ2 = 0.782, df = 4, p = .941; R.n.norvegicus: χ2 = 1.339, df = 3, p = .720; R.n.caraco: χ2 = 0.157, df = 3, p = .984) ().
Figure 1. Average percentage mortality caused by DR8 across all dose groups for male, female and both sexes of Rattus species, R.losea, R.n.norvegicus and R.n.caraco (for R.n.caraco, 15 mg/kg was exclusive); no differences were found either inter-species/subspecies of Rattus or between sexes of same species.
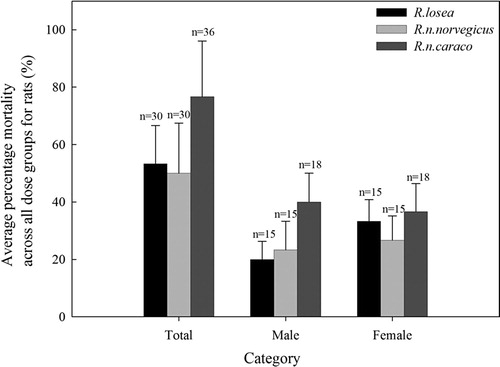
Table 1. Mortality (% of deaths) of six rodent species dosed with different levels of DR8 (n = 6 per group).
Time to death in Rattus
In all sensitive species, onset of symptoms occurred after 10–20 minutes following oral gavage of DR8, the treated animals showing a cycle of normal versus heavy breathing for 20–30 minutes, at which time the animals generally became very quiet, often sitting hunched in the corner of the cage. After a further 10–20 minutes, some showed a loss of balance often with staggered gait and more obvious dyspnoeic, and then died and/or some of them recovering after a few hours. Severity of effects was directly related to the level of dose. The time to death occurred between 0.5 and 30 hours, at the level of dose proximate to the LD50 value; 30 mg/kg for R.n.norvegicus, 20 mg/kg for R.n.caraco and 30 mg/kg for R.losea with the average times to death being 9.87, 7.15 and 10.1 hours, respectively (). There were no significant differences in the average time to death among species at the dose of 30 mg/kg (F(2,10) = 0.646, p = .544) and 100 mg/kg (F(2,13) = 3.705, p = .053), respectively. However, the time to death in R.losea was obviously longer than that of R.n.norvegicus and R.n.caraco at the dose of 50 mg/kg (F(2,13) = 10.304, p = .002), but no significant difference was found between the other two species at this dose ().
Median lethal dose (LD50) in Rattus
Mortality increased with the concentration of DR8 in all Rattus species, with a linear dose effect apparent between percentage mortality and log-dose of compound in all species (probit analysis) (). The LD50 of DR8 in these rodent species were calculated to be 27.89 mg/kg (7.24–48.54, 95% confidence interval) for R.losea, 32.93 mg/kg (16.69–49.17, 95% confidence interval) for R.n.norvegicus and 16.15 mg/kg (8.72–23.58, 95% confidence interval) for R.n.caraco.
Discussion
Presently, anticoagulant rodenticide is the most widely used toxicant for rodent management in the majority of countries. However, there are obvious disadvantages to using these compounds such as risks to non-target species and environmental persistence that compel researchers to explore more safer toxicants as alternative control tools. Development of a species-specific rodenticide is one of a number of research approaches under investigation (Campbell et al. Citation2015). One such approach is based on NRB, a promising Rattus-specific rodenticide found to be uniquely toxic to some species of rats but relatively harmless to other rodents and mammals (Roszkowski et al. Citation1964, Citation1965). NRB was discovered in the 1960s and introduced onto the commercial market fifty years ago under the trade names of Shoxin® and Raticate®. From the reported articles, NRB was highly toxic to the species of Rattus with LD50 values of 11.5 mg/kg (11.0–13.0, 95% confidence interval), 52 mg/kg (47.0–57.0, 95% confidence interval) and 10 mg/kg for R.norvegicus, R.rattus and R.hawaiiensis, respectively (Roszkowski et al. Citation1965). To most other non-Rattus species, the LD50 values of the drug were extremely high and in some cases showed no sensitivity to the drug at all (Roszkowski et al. Citation1964, Citation1965). However, the toxicant failed commercially due to the high incidence of bait-shyness induced through its extremely fast-acting activity (symptoms appeared after 5 minutes) and/or bad physiological sensation (Choi et al. Citation2016) which limited the efficacy of NRB-containing bait against rodents in field operations to less than 30% of the population were killed (Gratz Citation1973).
With the requirement to refine the use of rodent control substances particularly for the conservation of non-target organisms, there has been renewed interest in NRB with modifications of the structure being investigated to overcome this bait-shyness issue (Choi et al. Citation2016; Jay-Smith et al. Citation2016). DR8 is one of the derivatives of NRB synthetised by researchers from New Zealand, which appears to have overcome the rapid onset of NRB activity and the corresponding onset of bait-shyness whilst retaining its species-specific toxicity (Choi et al. Citation2016). Choice trials have shown that consumption of bait containing DR8 was 67% more than that of bait containing a similar concentration of NRB in Sprague–Dawley rat, and 2-3 times more in R.norvegicus and R.rattus (Choi et al. Citation2016). In our results for the 6 rodent species profiled, LD50 values of DR8 in R.losea, R.n.norvegicus and R.n.caraco were less than 50 mg/kg (), while the non-Rattus species B.indica, L.brandtii and M.fortis were insensitive to this toxicant (). For Rattus species, the LD50 value of DR8 (16.15 mg/kg and 32.96 mg/kg for R.n.caraco and R.n.norvegicus) was 1.4–2.8 times more than NRB (11.5 mg/kg for R.n.norvegicus) (Roszkowski et al. Citation1965), but this compound still retained a high toxicity. In our trials, the animals receiving oral DR8 gradually became more and more staggered and dyspnoeic before death, the symptoms being similar to that reported for NRB (Roszkowski et al. Citation1964; weber Citation2014), but the time of onset delayed from about 5 minutes of the treatment for NRB to 10–20 minutes for DR8 (Rennison et al, Citation2013). In addition, the time to death induced by DR8 was related to the level of dose. The average times to death (from 7.15 to 10.1 hours) caused by the dose proximate to the LD50 value were longer than NRB (within 35 minutes) in rats () (Roszkowski et al. Citation1964; weber Citation2014). That means DR8 modification results in a delay in the release of the active ingredient (NRB) in the rat.
Generally, our results demonstrated that DR8, a new analogue of NRB, retains NRB’s unique species-selective characteristics but time to onset of symptoms and death is delayed compared to NRB, such that sub-lethal doses and bait shyness are less likely to occur in cage and field efficacy trials against sensitive species of rats. This compound displays a promising profile for species-specific rodent pest management, and appears to be one of the most exciting developments in the rodent control research area for quite some time. A suitable palatable bait formulation needs now to be developed to maximise DR8s potential in cage and field trials.
Acknowledgements
The authors thank Dr. Wang Yong and Dr. Liu Xiaohui for providing excellent facilities during our experiments.
Disclosure statement
No potential conflict of interest was reported by the authors.
Additional information
Funding
References
- Al-Ali A, Alkhawajah AA, Randhawa MA, Shaikh NA. 2008. Oral and intraperitoneal LD50 of thymoquinone, an active principle of Nigella sativa, in mice and rats. Journal of Ayub Medical College Abbottabad. 20(2):25–27.
- Alomar H, Chabert A, Coeurdassier M, Vey D, Berny P. 2018. Accumulation of anticoagulant rodenticides (chlorophacinone, bromadiolone and brodifacoum) in a non-target invertebrate, the slug, Deroceras reticulatum. Science of the Total Environment. 610:576–582. doi: 10.1016/j.scitotenv.2017.08.117
- Atkinson IAE. 1985. The spread of commensal species of Rattus to oceanic islands and their effects on island Avifaunas. In: Moors PJ, editor. Conservation of island birds. Cambridge: ICPB Technical Publication No. 3, International Council for Bird Preservation [now BirdLife International]; p. 35–81.
- Battersby S, Hirschhorn RB, Amman RB. 2008. Commensal rodents. In: Bonnefoy X, Kampen H, Sweeney K, editors. Public health significance of urban pests. Regional office for Europe. Copenhagen (Denmark): World Health Organization; p. 387–419.
- Bronstein AC, Spyker DA, Cantilena LR, Green JL, Rumack BH, Heard SE. 2008. 2007 annual report of the American association of poison control centers’ national poison data system (NPDS): 25th annual report. Clinical Toxicology. 46(10):927–1057. doi: 10.1080/15563650802559632
- Brown PR, Douangboupha B, Htwe NM, Jacob J, Mulungu L, Nguyen TMP, Singleton GR, Stuart AM, Sudarmaji. 2017. Control of rodent pests in rice cultivation. In: Sasaki T, editor. Achieving sustainable rice cultivation. Cambridge: Burleigh Dodds Science Publishing; p. 1–34.
- Buckle AP, Eason CT. 2015. Control methods: chemical. In: Buckle AP, Smith RH, editors. Rodent pests and their control. 2nd ed. Wallingford: CABI; p. 123–155.
- Buckle AP, Smith RH. 1994. Rodent pests and their control. Wallingford: CABI. 405 p.
- Campbell KJ, Beek J, Eason CT, Glen AS, Godwin J, Gould F, Holmes ND, Howald GR, Madden FM, Ponder JB, et al. 2015. The next generation of rodent eradications: innovative technologies and tools to improve species specificity and increase their feasibility on islands. Biological Conservation. 185:47–58. doi: 10.1016/j.biocon.2014.10.016
- Choi H, Conole D, Atkinson DJ, Laita O, Jay-Smith M, Pagano MA, Ribaudo G, Cavalli M, Bova S, Hopkins B, et al. 2016. Fatty acid-derived pro-toxicants of the rat selective toxicant Norbormide. Chemistry & Biodiversity. 13(6):762–775. doi: 10.1002/cbdv.201500241
- Eason CT, Murphy EC, Wright GRG, Spurr EB. 2002. Assessment of risks of brodifacoum to non-target birds and mammals in New Zealand. Ecotoxicology. 11(1):35–48. doi: 10.1023/A:1013793029831
- Eason CT, Shapiro L, Ogilvie S, King C, Clout M. 2017. Trends in the development of mammalian pest control technology in New Zealand. New Zealand Journal Zoology. 44(4):267–304. doi: 10.1080/03014223.2017.1337645
- Gratz NG. 1973. A critical review of currently used single-dose rodenticides. Bulletin of the World Health Organization. 48(4):469–477.
- Greaves JH, Rowe FP, Redfern R, Ayres P. 1968. Microencapsulation of rodenticides. Nature. 219(5152):402–403. doi: 10.1038/219402a0
- Guo YW, Wang D, Shi DZ. 2013. Rodent pests damage and the management in the agricultural regions in China. China Plant Protection. 39(5):62–69. (In Chinese).
- Howald G, Donlan CJ, Galvan JP, Russell JC, Parkes J, Samaniego A, Wang YW, Veitch D, Genovesi P, Pascal M, et al. 2007. Invasive rodent eradication on islands. Conservation Biology. 21(5):1258–1268. doi: 10.1111/j.1523-1739.2007.00755.x
- Howald GR, Mineau P, Elliott JE, Cheng KM. 1999. Brodifacoum poisoning of avian scavengers during rat control on a seabird colony. Ecotoxicology. 8(6):431–447. doi: 10.1023/A:1008951701780
- Jay-Smith M, Murphy EC, Shapiro L, Eason CT, Brimble MA, Rennison D. 2016. Stereoselective synthesis of the rat selective toxicant norbormide. Tetrahedron. 72(35):5331–5342. doi: 10.1016/j.tet.2016.07.014
- Kausrud KL, Mysterud A, Steen H, Vik JO, Østbye E, Cazelles B, Framstad E, Eikeset AM, Mysterud I, Solhøy T, et al. 2008. Linking climate change to lemming cycles. Nature. 456(7218):93–97. doi: 10.1038/nature07442
- Kusano T. 1975. Physiological and psychological analysis of the acceptability of acute rodenticides by rats. Journal of the Faculty of Agriculture Tottori University. 10:15–26.
- Meerburg BG, Singleton GR, Kijlstra A. 2009. Rodent-borne diseases and their risks for public health. Critical Reviews in Microbiology. 35(3):221–270. doi: 10.1080/10408410902989837
- Pelfrene AF. 2001. Rodenticides. In: Krieger RI, editor. Handbook of pesticide toxicology. 2nd ed. San Diego (CA): Academic Press; p. 1793–1836.
- Rennison D, Laita O, Bova S, Cavalli M, Hopkins B, Linthicum DS, Brimble MA. 2012. Design and synthesis of prodrugs of the rat selective toxicant norbormide. Bioorganic & Medicinal Chemistry. 20(13):3997–4011. doi: 10.1016/j.bmc.2012.05.014
- Rennison D, Laita O, Conole D, Jay-Smith M, Knauf J, Bova S, Cavalli M, Hopkins B, Linthicum DS, Brimble MA. 2013. Prodrugs of N-dicarboximide derivatives of the rat selective toxicant norbormide. Bioorganic & Medicinal Chemistry. 21(18):5886–5899. doi: 10.1016/j.bmc.2013.06.071
- Roszkowski AP, Nause BR, Michael EH, Jacobs L. 1965. The pharmacological properties of norbormide, a selective rat toxicant. Journal of Pharmacology and Experimental Therapeutics. 149(2):288–299.
- Roszkowski AP, Poos GI, Mohrbacher RJ. 1964. Selective rat toxicant. Science. 144(3617):412–413. doi: 10.1126/science.144.3617.412
- Ruiz-Suárez N, Henríquez-Hernández LA, Valerón PF, Boada LD, Zumbado M, Camacho M, Almeida-González M, Luzardo OP. 2014. Assessment of anticoagulant rodenticide exposure in six raptor species from the canary islands (Spain). Science of Total Environment. 485:371–376. doi: 10.1016/j.scitotenv.2014.03.094
- Singla N, Parshad VR. 2010. Efficacy of acute and anticoagulant rodenticide baiting in sugarcane fields of Punjab, India. International Journal of Pest Management. 56(3):201–210. doi: 10.1080/09670870903342547
- Singleton GR. 2003. Impacts of rodents on rice production in Asia. IRRI Discuss Paper Serier. 45:1–30.
- Thomas PJ, Mineau P, Shore RF, Champoux L, Martin PA, Wilson LK, Fitzgerald G, Elliott JE. 2011. Second generation anticoagulant rodenticides in predatory birds: probabilistic characterisation of toxic liver concentrations and implications for predatory bird populations in Canada. Environment International. 37(5):914–920. doi: 10.1016/j.envint.2011.03.010
- Valchev I, Binev R, Yordanova V, Nikolov Y. 2008. Anticoagulant rodenticide intoxication in animals–a review. Turkish Journal of Veterinary and Animal Science. 32(4):237–243.
- Weber LP. 2014. Norbormide. Encyclopedia of Toxicology. 3:617–618. doi: 10.1016/B978-0-12-386454-3.00171-8