Abstract
Understanding the effect of seed transmission of Maize chlorotic mottle virus (MCMV) is important in managing the spread of the disease especially secondary transmission caused by vectors. This study was set out to investigate the effect of different genotypes and crop growth stage at the time of infection to rate of MCMV transmission from seeds to seedlings. The experiments were laid out in randomised complete block design with three replications. Detection of MCMV in the grow outs was determined using double antibody sandwich enzyme-linked immunosorbent assay and real time reverse transcription polymerase chain reaction. The results showed low rates of seed transmission from the genotypes in which their was detection of MCMV in their samples (0.08–1%). Similarly, crop growth stage at time of infection also showed low transmission rate of up to 0.1%, with highest transmission rate at the four-leaf stage.
1. Introduction
Maize is a major source of food in subsaharan Africa, leading to expansion of production over the years (Santpoort Citation2020). The crop is a main staple food in the eastern Africa region, in particular Kenya, with an estimated consumption of 67.3 kg/person in 2019 (KNBS Citation2020). Maize lethal necrosis (MLN) is a devastating disease that causes high losses in maize yields (De Groote et al.Citation2016; Kagoda et al.Citation2016) in both farmers and seed production fields (De Groote et al.Citation2016; Eunice et al.Citation2021). The disease is caused by Maize chlorotic mottle virus (MCMV) when in synergistic interaction with a cereal potyvirus, with the Sugarcane mosaic virus (SCMV), as the most common potyvirus in the eastern Africa region (Mahuku et al.Citation2015; Redinbaugh and Stewart Citation2018). The disease increases production challenges, ultimately causing food shortage. In the eastern African region, MCMV was first reported in 2011 (Wangai et al.Citation2012) while SCMV was reported since 1930s (Storey Citation1936). The recent MLN outbreak in the region has therefore been mainly attributed to MCMV.
Maize chlorotic mottle virus belongs to the family Tombusviridae and genus Machlomovirus (Nutter et al.Citation1989). There is 96–99% similarities of the complete genome of the virus in different countries where it has been reported (Adams et al.Citation2014; Braidwood et al.Citation2018; Wamaitha et al.Citation2018; Kiruwa et al.Citation2020; Mwatuni et al.Citation2020). Prior to the identification in eastern Africa, MCMV had been reported in Peru, Kansas, Nebraska, Hawaii and China and later in Taiwan and Ecuador (Niblett and Claflin Citation1978; Jiang et al.Citation1992; Xie et al.Citation2011; Deng et al.Citation2014; Quito-Avila et al.Citation2016). Infection of maize by MCMV causes leaf mosaic with fine chlorotic and longitudinal yellow streaks, which lead to chlorotic mottling. The symptoms deteriorate to leaf necrosis, yield reduction, stunting and death of the plant in severe infections (Nelson et al.Citation2011).
Research efforts have been geared towards understanding MLN disease, the epidemiology of the causative viruses, how to manage the viruses and transmitting insect vectors, and development of maize resistant varieties (Prasanna et al.Citation2020). The transmission of MCMV is by insect vectors, through seed and mechanical means e.g. farm implements that have been contaminated with MCMV-infected crop or soil residues (Nelson et al.Citation2011). Corn thrips (Frankliniella williamsi) (Wangai et al.Citation2012; Cabanas et al.Citation2013; Mahuku et al.Citation2015; Kiruwa et al.Citation2020), the southern corn rootworm (Diabrotica undecimpunctata), the northern corn rootworm (D. longicornis), western corn rootworm (D. virgifera), cereal leaf beetle (Oulema melanopa), corn flea beetle (Chaetocnema pulicaria) and flea beetle (Systena frontalis) (Nault et al.Citation1978) are vectors that transmit MCMV in maize. Evidence of seed transmission of MCMV is reported at low rates (Jensen et al.Citation1991; Kitira Citation2018; Kimani et al.Citation2021). Factors that contribute to transmission of MCMV include the host variety, environmental factors, virus isolate and crop/plant growth stage at the time of infection (Maule and Wang Citation1996; Albrechtsen Citation2006). The danger of seed transmitted viruses is that they introduce virus inoculum into new fields, or new locations. The virus is then spread by insect vectors where present causing epidemic (Sastry Citation2013a).
In this study the transmission of MCMV through seed of various maize genotypes was screened for commercial and breeders’ hybrids in Kenya. The study also evaluated the effect of crop stage at time of infection in transmission of MCMV from seeds obtained from infected plants to seedlings. Development of resistant varieties against the causative viruses and management of transmission of the vector-transmitting MCMV are a sustainable way in the management of MCMV. Thus, identifying the crop stage that is risky for infection of the seed is important in development of management strategies against MLN especially in seed production fields.
2. Materials and methods
2.1. Experimental materials
Two experiments were carried out in this study. The first experiment was conducted to screen the effect of genotypes on seed transmission of MCMV. In this experiment, MCMV-free seeds (certified by Kenya Plant Health Inspectorate Services)of ten varieties (commercial hybrids) were obtained from local agrovet shops and five breeders’ hybrids developed by Kenya Agricultural and Livestock Research organization (KALRO) and International Maize and Wheat Improvement Center (CIMMYT) (). Most of the commercial hybrids are susceptible to MCMV (Gowda et al.Citation2015; Sitta et al.Citation2017) while the breeders’ maize hybrids have varying tolerance levels to MLN disease.
Table 1. List of genotypes used in the study, their source and reaction to maize lethal necrosis (MLN) disease infection.
The second experiment was conducted to determine the effect of crop growth stage at time of infection on seed transmission of MCMV to the subsequent crop using commercial hybrids H614, Duma 43 and DK777. DK777 is tolerant to MLN (KEPHIS Citation2020) while H614 and Duma 43 are susceptible varieties.
MCMV-infected plants are maintained at KALRO Biosafety level II greenhouse and were used for preparation of virus inoculum for development of infected maize plants by experimental inoculation.
2.2. Screening for effect of genotypes on seed transmission of MCMV
In order to generate MCMV-infected seeds to be tested in experiment one, seeds from the genotypes listed in were sowed at the KALRO Njoro quarantine field in the year 2017 and 2018. KALRO Njoro is situated at an altitude of 2120 m above sea level, in Nakuru County (0.3409° S, 35.9435° E) in Kenya. Planting was during the long rainy season that lasted from March to late May with average annual rainfall of 960 mm, and average temperatures ranging from 9.7 °C (night) to 24 °C (noon). The seeds were sown in 2.5 m long plots with 5 rows per plot, and 25 cm spacing between holes. Di-ammonium phosphate (DAP 18:46:0) was applied at planting at a rate of 60kgP2O5/ha while calcium ammonium nitrate was applied at 60kgN/ha at eight weeks after planting. Three guard rows of a commercial variety, PH30G19 were planted around the experimental trial area. The experiment was laid out in a randomised complete block design.
Virus inoculum was prepared by grinding MCMV-infected leaves in 0.1 M phosphate buffer in the ratio of 1:10. The mixture was then passed through a muslin cloth to filter out the debris. The resulting inoculum was transported to the field site in a cool box. Carborundum (0.6% of 22 µm grit) was added to the mixture prior to inoculation. The inoculum was applied to the plants at four leaf stage using a motorized sprayer and the process was repeated after one week.
The field was kept weed free throughout the growth period and sprayed weekly with insecticides to control MCMV and SCMV vectors and fall army worm. The insecticides used included 50 g/liter lufenuron (Match 050EC, Syngenta), 45 g/litre Chlorantraniliprole and 18 g/litre Abamectin (Voliam targo® 063SC, Syngenta) and 19 g/liter emamectin benzoate (Escort 19EC, Greenlife Crop Protection). The plants were visually inspected once a week and the symptom severity of MCMV infection was scored using a scale of 1 to 5 (Karanja et al., Citation2018) where 1 = no MCMV symptoms, 2 = fine chlorotic streaks on leaves, 3 = chlorotic mottling throughout plant, 4 = excessive chlorotic mottling, necrosis on leaves and in some cases dead heart and 5 = complete plant necrosis. At maturity, the maize seed was harvested from the middle three rows threshed and winnowed by hand to avoid cross contamination between the different plots. The seed was then sun-dried to a moisture content of 13%, before storage at room temperature. Testing for MCMV transmission from the harvested seeds was then done as described in Section 2.4 below. The maize stalks were removed from the field, allowed to dry in a pit before burning to ashes. No maize was sowed in the field where the experiment was laid for one year after harvest.
2.3. Determining the effect of crop growth stage at time of infection on seed transmission of MCMV
Seeds of commercial varieties, Duma 43, H614 and DK777, were sowed in KALRO Njoro quarantine field, in similar spacing, plot sizes and field management practices as described in the above experiment. The experiment was laid out in a randomised complete block design with three replicates. The treatments comprised of the crops stages at time of inoculation which were: four-leaf (V4), eight-leaf (V8), 15-leaf (V15), tasselling (VT) stages and a control that was not inoculated. Three guard rows were planted in between the plots to separate the crops inoculated at different crop stages. Disease severity was scored using the scale described above once a week. Harvesting, threshing and storage were done as in the previous experiment. The seeds obtained were tested for the rates of MCMV transmission together with those obtained from experiment one.
2.4. Seed grow-out tests to assess the effect of genotype and time of infection on seed transmission of MCMV
The seeds obtained from the MCMV-infected plants from the two field experiments above were sown in separate trays that were filled with a mixture of sterile soil and vermiculite (2:1) and placed in an insect-free seed laboratory at KALRO Kabete, at average day temperature of 21 °C with 12 light and night hours. On each tray, 20 or 50 seeds were sown. MCMV-free certified seed purchased from an agrovet was planted on a separate tray and used as healthy negative control. The seeds were handled with clean gloves to avoid contamination between seeds from different sources.
Ten days after planting (at two-leaf stage), the germinated seedlings were counted and recorded, and a leaf from each germinated seedling on a tray was sampled and placed in one bag. These were analysed as pooled samples in the laboratory (Shango et al.Citation2019). The pools consisted of a range of 18–50 seedling leaves samples, depending on how many seeds were sown and those that germinated. Twenty to thirty pooled samples were tested for presence of MCMV using double antibody sandwich enzyme linked immunosorbent assay (DAS-ELISA) while pooled samples tested using real time reverse transcription polymerase chain reaction (real time RT-PCR) were fewer (18 pooled samples) due to limitation of reagents for this method
Polyclonal MCMV antiserum for DAS-ELISA was developed at the University of Minnesota. A brief procedure of DAS-ELISA involved coating 96-well plates with coating antibody diluted in carbonate buffer (1:1000). The plates were incubated for one hour at 37 °C, then washed using phosphate buffered saline with tween solution (PBST). Hundred microlitres of the samples were then added to the coated wells. Each sample was duplicated on the plate. After one hour incubation and a washing step, the secondary antibody (1:4000) conjugated to the enzyme alkaline phosphatase was added to the wells. Enzyme alkaline phosphatase substrate, para-nitrophenyl phosphate, was added to the wells after incubation and washing. The absorbance value at 405 nm (A405nm) from the DAS-ELISA was recorded using a microplate reader (Elx808 BioTek Instruments) after the plates were incubated at room temperature for one hour. A sample was considered positive when the mean of the duplicates A405nm readings was twice that of the healthy control.
RNA was extracted from leaf samples using CTAB modified method (Monger et al. Citation2001). Real-time RT-PCR was carried out using SensiFAST Probe Hi-Rox one-step kit (BIO 77001, Bioline USA, Taunton, MA). Five microliters of SensiFAST probe Hi-Rox one-step buffer was mixed with forward and reverse MCMV primers (0.5 μl) (forward primer 5′-GAGTCCTGCCAATCCAAAGTG-3′, reverse primer 5′-TGGGTGGGTCAAGGCTTACTA-3′) and 0.3 μl fluorescent-labelled probe (5′-FAM-AGCCGCCGCCCACTCTCCAG-BHQ-3′) (Liu et al. Citation2016), 0.2 μl RNase inhibitor, 0.1 μl of reverse transcriptase, 2 μl of RNA template and 1.4 μl of diethyl pyrocarbonate-treated water. A real-time PCR equipment was used to run the reactions at 50 °C for 10 min, 95 °C for 1 min, and then 40 cycles of 94 °C for 10 s and 60 °C for 1 min (Kimani et al. Citation2021). Data collected from real time RT-PCR was the cycle threshold (Ct) values determined by the automatic threshold and baseline setting of the StepOne plus software. Samples with average Ct values that were less than those of the healthy and negative controls (Ct value higher than 30) were assigned as positive for MCMV.
2.4.1. Data analysis
Statistical analysis on the MCMV severity ordinal data was assessed for differences using the Kruskal-Wallis test using the base packages in R (R Core Team Citation2021). The severity scores observed for the genotypes evaluated were compared using the Dunns test in the rstatix package (R Core Team Citation2021).
Ordinal logistic regression was used for the analysis of the non-parametric data on the severity of MCMV observed when three maize varieties were inoculated at various crops stages. The severity scores were categorized into three groups of no symptoms, below 2.5 and above 2.5 and their association with the crops stage and variety, determined. The proportional odds logistic regression from the MASS package was used (Venables and Ripley Citation2002) for determination of the odds ratio and significant differences on the overall data and at 63 days post inoculation separately. Descriptive statistics were included to show the importance of the crop stages at time of infection and the varieties on MCMV severity.
Seed transmission rates were determined by subjecting the data on number of sample pools with the positive virus detection, and total number of pools tested using DAS-ELISA and real time RT-PCR assays, to variable pool sizes and perfect test on the Epitools Epidemiological calculators (Sergeant Citation2018). The data was analysed depending on number of seedling samples per pool. Pearson correlation of the severity scores of the genotypes to the seed transmission rates; and for the crops stage at time of infection to the seed transmission rate was determined in R software.
3. Results
3.1. Effect of genotype on seed transmission of MCMV
The range of the mean severity score of the genotypes ranged from 1.35 to 2.88 as the disease progressed differently in the genotypes analysed, and was significantly different for the 15 maize genotypes (p = 0.001, Kruskal Wallis test) at 63 days post inoculation. The severity of MCMV also differed significantly (p < 0.001) between the two years with higher mean severity scores of 2.39 recorded in 2017 compared to severity score of 2.12 in 2018. The genotypes WE5139, WE5140, WE5138, KATEH 16-01, KATEH 16-02 and DK777 had significantly (p < 0.05) low MCMV symptoms severity as compared to PH30G19, that had the highest severity score ().
Table 2. Comparison of mean MCMV severity scores at 63 days post inoculation for 15 genotypes with that of the genotype on top row (DH02).
When transmission of MCMV from seeds to seedling grow-outs were tested using DAS-ELISA, most of the pooled samples had absorbance values (A405nm) that were less than twice that of the healthy controls which ranged from 0.118–0.175. Fifteen samples had values higher than twice the healthy controls ranging from 0.288 to 0.52. Only in one sample was MCMV detected and this was from the 2018 harvest the other 14 were from the samples harvested in 2017. The samples were from varieties - Duma 43, KH500-33A, WE5138, DK8031 and DH02 with transmission rates ranging from 0.08 to 0.48% ().
Table 3. Seedling grow-outs samples that tested positive for MCMV from different genotypes for seed harvested in 2017 and 2018 by DAS-ELISA.
Real time RT-PCR was used to detect MCMV in 1,642 seedlings that were harvested in 2018. Fourteen pooled samples from genotypes DK8031, H6213, H625, KATEH 16-01, KATEH 16-02, PH30G19, PHB3253, WE5138 and WE5140 had average Ct values that were lower than that of the healthy negative controls. The average Ct values for the negative controls ranged from 31.04 to 34.40. This was the criteria used to identify the positive samples. The seed transmission rates when the samples were tested using real time RT-PCR were 1.04 and below (). The correlation between the severity of MCMV from the genotypes evaluated and seed transmission rates of MCMV was not significant (r = 0.02, p = 0.878).
Table 4. Number of seedlings tested in samples of seedling grow-outs from different genotypes (harvested in 2018) that had a positive MCMV detection and the seed transmission rate when tested with real time reverse transcription polymerase chain reaction.
3.2. Effect of crop growth stage at time of infection to seed transmission of MCMV
The mean MCMV severity score in this study ranged from 1.22 for plants of DK777 inoculated at V15 stage to 2.88 for Duma 43 plants inoculated at V4 stage. Crop stage at time of infection for the three varieties contributed to significant differences in severity of MCMV, with the overall severity at V4 and V8 stages being significantly different from the control (). The three varieties also presented significant differences of the severity scores (p < 0.001). DK777 had the lowest mean severity scores as compared to Duma 43 that had the highest mean severity score.
Table 5. Coefficient’s estimates, odds ratio and standard error of MCMV severity scores obtained from plants inoculated at different crop stages during the 2017 and 2018 year from the regression model.
At 63 days after the first inoculation at four-leaf stage, the mean severity for plants inoculated at four-leaf and eight-leaf stages were significantly higher (p < 0.0001) as compared to those inoculated at fifteen-leaf and tasselling stages and the control (). Duma 43 plants inoculated at four-leaf stage had the highest disease severityi, while the severity on DK777 on plants inoculated at all the crops stages and control were below 2 (). However, contamination with MCMV of the control plots was observed for all the three varieties.
Figure 1. Mean MCMV disease severity scores, 63 days after the first inoculation for three maize varieties inoculated at crop stages V4 (four-leaf), V8 (eight-leaf), V15 (fifteen-leaf), VT- tasselling and control that was not inoculated.
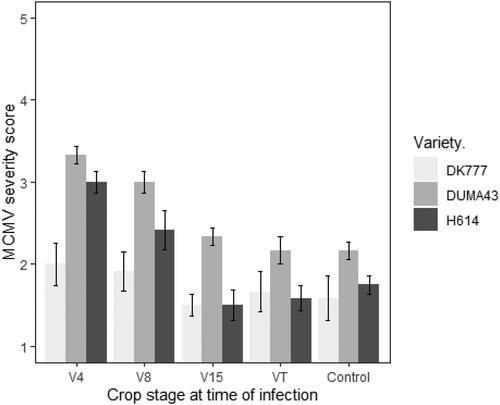
The seeds harvested from the plants inoculated at V4 and V8 stages in 2017 and 2018 were grown out, and transmission of MCMV tested using DAS-ELISA. The transmission rate was 0.1% for V4 stage (Kimani et al.Citation2021) with two positive samples from DK777 and one from H614 seeds. While the transmission rate from seed from the plants inoculated at V8 stage was 0.04%, with only one positive sample from the 1142 seedlings tested in 24 groups. There was no positive detection of MCMV from the samples obtained from the 2018 seed.
When samples from seed harvested in 2018 from the plants inoculated at V4 and V8 stages were tested using real-time RT-PCR, one pooled samples of variety DK777 inoculated at V8 stage was positive for MCMV (). Pearson correlation of the severity of the MCMV inoculated at different crops stages to the seed transmission rates of MCMV was not significant (r = 0.265, p = 0.339).
Table 6. MCMV Positive samples tested in 18 groups each of seedling grow outs using real-time RT-PCR and rate of transmission via seeds obtained from plants inoculated at four- and eight-leaf stages.
4. Discussion
Maize chlorotic mottle virus, one of the viruses involved in the development of maize lethal necrosis disease, is transmitted through seed to seedlings (Jensen et al.Citation1991; Kimani et al. Citation2021). Many factors contribute to the transmission of viruses through seed, however, in this study the effect of genotypes and crop growth stage at time of infection on the seed to seedling transmission of MCMV was assessed.
The study demonstrated that there was no correlation of severity of symptoms caused by MCMV infection due to the genotypes to transmission rate of the virus via seed. This was so despite there being significant differences (p ≤ 0.001) in the symptoms severity in the genotypes assessed. Similarly, there was no correlation of severity of symptoms caused by infection of MCMV due to time at which the plants were infected to seed transmission of the virus.
The results indicate that maize plants with varying severity of symptoms caused by MCMV infection can yield seeds that have the virus and this can be transmitted to seedlings at a rate of 0 to 1%. Use of resistant varieties is the most economical method for the management of maize lethal necrosis disease (Prasanna et al.Citation2020), and the study displayed evidence of transmission rate of 0 to 0.67% from seeds of MLN tolerant genotypes. Similar findings were reported for hybrids that have tolerance to MCMV, with cultivar WE5138 and WE5139 having seed transmission rates of 0.6% and 2.1%, respectively (Mwatuni Citation2021). Seed transmission of viruses is suggested to be controlled by genes that regulate the invasion of gametes prior to fertilization and those that control the direct invasion of the embryos (Maule and Wang Citation1996). Varietal effect on the transmission of Barley stripe mosaic virus have been reported with rates ranging from 0–75%, conditioned by the presence of a single recessive gene (Caroll et al.Citation1978). Similar effect of genotypes in the transmission of Pea seed-borne mosaic virus via seed varied with different pea cultivars despite plants showing symptoms (Stevenson and Hagedorn Citation1973), while transmission of Bean common mosaic virus strains in 12 bean cultivars varied (Morales and Castaño Citation1987).
The results in this study showed significantly higher MCMV severity at four leaf and eight-leaf stages as compared to the other stages (p < 0.001). Virus transmission via seed is higher when plants are infected in early growth stages (Sastry Citation2013b). The speed of movement of viruses in the host and ability to multiply in the plant inflorescence have been reported as the best estimators for seed transmission (Cobos et al.Citation2019). Once the virus is in the embryo, it’s transmission is at a higher rate. Legumes have been shown to have higher seed transmitted viruses at higher rates (Sastry Citation2013b). These is facilitated by the presence of more cytoplasmic structures (Sastry Citation2013b) that enable faster movement of viruses into the embryo, within the limited window that virus can invade (Cobos et al.Citation2019).
The transmission rate via seed for all the evaluated categories in the study was 1% and below. This was despite the knowledge of the location of the virus in the seed. This low rate is similar to the previous reports of MCMV transmission via seed from Hawaii (Jensen et al.Citation1991), Kansas and Nebraska (Bockelman et al.Citation1982) and Kenya (Kitira Citation2018; Kimani et al.Citation2021). Seed transmission of other viruses infecting maize has also been low, ranging from 0–4.8% (Mikel et al.Citation1984; Forster et al.Citation2001; Li et al.Citation2007).
Transmission of viruses through seed is affected by other factors (Sastry Citation2013c; Simmons and Munkvold Citation2014). The effect of strain in the transmission of the virus (Simmons and Munkvold Citation2014) is a key factor. However, MCMV isolates globally have high nucleotide sequence similarity of 96–100% (Braidwood et al.Citation2018), and the different isolates have shown equally low seed transmission of the virus. The rate of transmission is also low when the seeds were obtained from plants infected with both MCMV and a cereal potyvirus (MLN infection) (Kitira Citation2018).
Seed transmission of viruses is complicated where virus is spread by vectors. MCMV is spread by corn thrips and chrysomelid beetles (Nelson et al.Citation2011; Cabanas et al.Citation2013). Not only are the corn thrips present in most maize growing regions in Kenya (Mahuku et al.Citation2015), but they are also attracted to MCMV-infected plants by volatiles emitted (Mwando et al.Citation2018). Increase in the number of thrips leads to further spread of MCMV to other parts in the fields and surrounding maize fields. Since vectors are the main problem in secondary transmission of viruses (Sastry Citation2013a), efforts should be taken to understand the ecology of the vectors transmitting MCMV in the region, factors affecting the populations and management methods against the vectors (Redinbaugh and Stewart Citation2018).
The development of maize lethal necrosis is heightened when MCMV is present in plants before infection with a potyvirus (Kiambi et al.Citation2019). This emphasizes the need to control the introduction of MCMV through seed. Cereal potyviruses are common in Kenya and other maize growing areas and will lead to the development of MLN disease and increase in MCMV (Wang et al.Citation2017). The effect of the strains to transmission of MCMV and SCMV through seed would increase the understanding of this topic as well as assist in further development of methods for seed certification.
The effect of environment on seed transmission of MCMV was not factored in this study, however, there was higher MCMV transmission observed from seed harvested in 2017 as compared to 2018 with significant differences in the MCMV disease severity in the field recorded in the two years. The effect of environment on MCMV severity is not clear (Redinbaugh and Stewart Citation2018) as well as the effect of environmental factors to seed to seedling transmission.
5. Conclusion
The study concluded that seed transmission of MCMV was not significantly correlated to the maize genotypes studied nor to the crop stage at time of infection. The low rates of seed transmission detected are achieved by using both ELISA and real time RT-PCR. Despite the low rates of seed transmission detected, seed certification for MCMV is important and should be sustained for all maize genotypes. Low infection of MCMV in seedlings are of concern as these lead to attraction of corn thrips through volatile compounds released from MCMV-infected plants. This leads to secondary transmission through the insect vectors that causes wider and faster spread of the virus in the infected field.
Acknowledgements
This work was supported by the Bill & Melinda Gates Foundation (Grant Number: INV-006697/OPP1138693), project name “Understanding and Preventing Seed Transmission of Maize Lethal Necrosis (MLN) in Africa”. Under the grant conditions of the Foundation, a Creative Commons Attribution 4.0 Generic License has already been assigned to the author accepted manuscript version that might arise from this submission. The open access fee for publication of the manuscript was funded by CGIAR Plant Health Initiative.
The authors acknowledge Henry Onzere, George Mosota and Fredrick Otieno who provided immense support in the management of the plants in the greenhouse, preparation of the samples in the laboratory and management of the field trials; and the seed merchants who provided the seed from their commercial lots.
Disclosure statement
The authors declare no conflict of interest.
Data availability statement
The authors confirm that the data supporting the findings of this study is available within the article.
References
- Adams IP, Harju VA, Hodges T, Hany U, Skelton A, Rai S, Deka MK, Smith J, Fox A, Uzayisenga B, et al. 2014. First report of maize lethal necrosis disease in Rwanda. New Dis Rep. 29(1):22–22. doi:10.5197/j.2044-0588.2014.029.022.
- Albrechtsen SE. 2006. Seed transmission of viruses. In: Testing methods for seed-transmitted viruses: principles and protocols. Wallingford (UK): CABI Publishing; p. 14.
- Bockelman DL, Claflin LE, Uyemoto JK. 1982. Host range and seed transmission studies of Maize chlorotic mottle virus in grasses and corn. Plant Dis. 66(1):216–218. doi:10.1094/PD-66-216.
- Braidwood L, Quito-Avila DF, Cabanas D, Bressan A, Wangai A, Baulcombe DC. 2018. Maize chlorotic mottle virus exhibits low divergence between differentiated regional sub-populations. Sci Rep. 8(1):1173. doi:10.1038/s41598-018-19607-4.
- Cabanas D, Watanabe S, Higashi CHV, Bressan A. 2013. Dissecting the mode of Maize chlorotic mottle virus transmission (Tombusviridae: machlomovirus) by Frankliniella williamsi (Thysanoptera: thripidae). J Econ Entomol. 106(1):16–24. doi:10.1603/EC12056.
- Caroll TW, Gossel PL, Hockett HA. 1978. Inheritance to resistance to seed transmission of Barley stripe mosaic virus in barley. Phytopathology. 69:431–433.
- Cobos A, Montes N, López-Herranz M, Gil-Valle M, Pagán I. 2019. Within-host multiplication and speed of colonization as infection traits associated with plant virus vertical transmission. J Virol. 93(23):e01078-19. doi:10.1128/JVI.01078-19.
- De Groote H, Oloo F, Tongruksawattana S, Das B. 2016. Community-survey based assessment of the geographic distribution and impact of maize lethal necrosis (MLN) disease in Kenya. Crop Prot. 82:30–35. doi:10.1016/j.cropro.2015.12.003.
- Deng T-C, Chou C-M, Chen C-T, Tsai C-H, Lin F-C. 2014. First report of Maize chlorotic mottle virus on sweet corn in Taiwan. Plant Dis. 98(12):1748–1748. doi:10.1094/PDIS-06-14-0568-PDN.
- Eunice J, Miano D, W.m M, Mutitu E, Macharia I. 2021. Status of maize lethal necrosis disease in seed production system in Kenya. Cogent Food Agric. 7(1):1918406. doi:10.1080/23311932.2021.1918406.
- Forster RL, Seifers DL, Strausbaugh CA, Jensen SG, Ball EM, Harvey TL. 2001. Seed transmission of the High plains virus in sweet corn. Plant Dis. 85(7):696–699. doi:10.1094/PDIS.2001.85.7.696.
- Gowda M, Das B, Makumbi D, Babu R, Semagn K, Mahuku G, Olsen MS, Bright JM, Beyene Y, Prasanna BM. 2015. Genome-wide association and genomic prediction of resistance to maize lethal necrosis disease in tropical maize germplasm. Theor Appl Genet. 128(10):1957–1968. doi:10.1007/s00122-015-2559-0.
- Jensen SG, Wysong DS, Ball EM, Higley PM. 1991. Seed transmission of Maize chlorotic mottle virus. Plant Dis. 75(5):497–498. doi:10.1094/PD-75-0497.
- Jiang XQ, Meinke LJ, Wright RJ, Wilkinson DR, Campbell JE. 1992. Maize chlorotic mottle virus in Hawaiian-grown maize: vector relations, host range and associated viruses. Crop Prot. 11(3):248–254. doi:10.1016/0261-2194(92)90045-7.
- Kagoda F, Gidoi R, Isabirye BE. 2016. Status of maize lethal necrosis in eastern Uganda. Afr J Agric Res. 11:652–660.
- Karanja J, Derera J, Gubba A, Mugo S, Wangai Ann. 2018. Response of selected maize inbred germplasm to maize lethal necrosis disease and its causative viruses (sugarcane mosaic virus and maize chlorotic mottle virus) in Kenya. TOASJ. 12(1):215–226. doi:10.2174/1874331501812010215.
- KEPHIS. 2020. National crop variety list Kenya.
- Kiambi RG, Miano DW, Wagacha JM, Wangai AW. 2019. Effect of sequence of infection of maize with viruses causing maize lethal necrosis on disease development. J Nat Sci Res. 9:44–50. doi:10.7176/JNSR/9-8-06.
- Kimani EN, Kiarie SM, Micheni CM, Muriki LG, Miano DW, Macharia I, Munkvold GP, Muiru WM, Prasanna BM, Wangai A. 2021. Maize seed contamination and seed transmission of Maize chlorotic mottle virus in Kenya. Plant Health Prog. 22(4):496–502. doi:10.1094/PHP-02-21-0018-RS.
- Kiruwa FH, Mutiga S, Njuguna J, Machuka E, Senay S, Feyissa T, Ndakidemi PA, Stomeo F. 2020. Status and epidemiology of maize lethal necrotic disease in Northern Tanzania. Pathogens. 9(1):4. doi:10.3390/pathogens9010004.
- Kitira FG. 2018. Determining the role of seed and soil in the transmission of viruses causing maize lethal necrosis disease [MSc thesis]. Nairobi (Kenya): University of Nairobi.
- KNBS. 2020. Economic survey 2020. Nairobi (Kenya): Kenya National Bureau of Statistics.
- Li L, Wang X, Zhou G. 2007. Analyses of maize embryo invasion by Sugarcane mosaic virus. Plant Sci. 172(1):131–138. doi:10.1016/j.plantsci.2006.08.006.
- Liu Z, Zhang L, Yang C, Xia X. 2016. Development of real-time reverse transcription PCR for detection of maize chlorotic mottle virus based on a novel molecular marker. Cogent Food & Agric. 2(1). doi:10.1080/23311932.2016.1224047.
- Mahuku G, Lockhart BE, Wanjala B, Jones MW, Kimunye JN, Stewart LR, Cassone BJ, Sevgan S, Nyasani JO, Kusia E, et al. 2015. Maize lethal necrosis (MLN), an emerging threat to maize-based food security in Sub-Saharan Africa. Phytopathology. 105(7):956–965. doi:10.1094/PHYTO-12-14-0367-FI.
- Maule AJ, Wang D. 1996. Seed transmission of plant viruses: a lesson in biological complexity. Trends Microbiol. 4(4):153–158. doi:10.1016/0966-842x(96)10016-0.
- Mikel MA, D’Arcy CJ, Ford RE. 1984. Seed transmission of Maize dwarf mosaic virus in sweet corn. Phytopathol Z. 110(3):185–191., doi:10.1111/j.1439-0434.1984.tb00746.x.
- Monger WA, Seal S, Cotton S, Foster GD. 2001. Identification of different isolates of cassava brown streak virus and development of a diagnostic test. Plant Pathol. 50(6):768–775. doi:10.1046/j.1365-3059.2001.00647.x.
- Morales FJ, Castaño M. 1987. Seed transmission characteristics of selected bean common mosaic virus strains in differential bean cultivars. Plant Dis. 71(1):51–53. doi:10.1094/PD-71-0051.
- Mwando NL, Tamiru A, Nyasani JO, Obonyo MAO, Caulfield J, C, Bruce TJA, Subramanian S. 2018. Maize chlorotic mottle virus induces changes in host plant volatiles that attract vector thrips species. J Chem Ecol. 44(7-8):681–689. doi:10.1007/s10886-018-0973-x.
- Mwatuni FM. 2021. Distribution, molecular characterization, MCMV seed transmission and diagnostics of viruses causing maize lethal necrosis (MLN) - in Kenya [PhD thesis]. Kenya: Jomo Kenyatta University of Agriculture and Technology.
- Mwatuni FM, Nyende AB, Njuguna J, Xiong Z, Machuka E, Stomeo F. 2020. Occurrence, genetic diversity, and recombination of maize lethal necrosis disease-causing viruses in Kenya. Virus Res. 286:198081. doi:10.1016/j.virusres.2020.198081.
- Nault LR, Styer WE, Coffey ME, Gordon DT, Negi LS, Niblett CL. 1978. Transmission of Maize chlorotic mottle virus by Chrysomelid beetles. Phytopathology. 68(7):1071. doi:10.1094/Phyto-68-1071.
- Nelson S, Brewbaker J, Hu J. 2011. Maize chlorotic mottle. Plant Dis. 79:1–6.
- Niblett CL, Claflin LE. 1978. Corn lethal necrosis- a new virus disease in maize in Kansas. In: The plant disease reporter. Beltsville, Maryland, USA: Bureau of Plant Industry, U.S. Department of Agriculture; p. 15–19.
- Nutter RC, Scheets K, Panganiban LC, Lommel SA. 1989. The complete nucleotide sequence of the Maize chlorotic mottle virus genome. Nucleic Acids Res. 17(8):3163–3177. doi:10.1093/nar/17.8.3163.
- Prasanna BM, Suresh LM, Mwatuni F, Beyene Y, Makumbi D, Gowda M, Olsen M, Hodson D, Worku M, Mezzalama M, et al. 2020. Maize lethal necrosis (MLN): efforts toward containing the spread and impact of a devastating transboundary disease in sub-Saharan Africa. Virus Res. 282:197943. doi:10.1016/j.virusres.2020.197943.
- Quito-Avila DF, Alvarez RA, Mendoza AA. 2016. Occurrence of maize lethal necrosis in Ecuador: a disease without boundaries? Eur J Plant Pathol. 146(3):705–710. doi:10.1007/s10658-016-0943-5.
- R Core Team. 2021. R: a language and environment for statistical computing.
- Redinbaugh MG, Stewart LR. 2018. Maize lethal necrosis: an emerging, synergistic viral disease. Annu Rev Virol. 5(1):301–322. doi:10.1146/annurev-virology-092917-043413.
- Santpoort R. 2020. The drivers of maize area expansion in Sub-Saharan Africa. How policies to boost maize production overlook the interests of smallholder farmers. Land. 9(3):68. doi:10.3390/land9030068.
- Sastry KS. 2013a. Identification and taxonomic groups. In: Seed-borne plant virus diseases. New Delhi, India: Springer; p. 55–58. doi:10.1007/978-81-322-0813-6.
- Sastry KS. 2013b. Mechanism of seed transmission. In Seedborne plant virus diseases. New Delhi, India: Springer; p. 85–95.
- Sastry KS. 2013c. Virus transmission. In: Seed-borne plant virus diseases. New Delhi, India: Springer; p. 75–83. doi:10.1007/978-81-322-0813-6.
- Sergeant ESG. 2018. Epitools epidemiological calculators. https://epitools.ausvet.com.au/.
- Shango P, Onyango C, Oganda JK, Macharia I, Kariuki G. 2019. Seed certification as a means of curbing emerging diseases: a case study of maize lethal necrosis disease in Kenya. J Agric Res Adv. 1:39–43.
- Simmons HE, Munkvold GP. 2014. Seed transmission in the potyviridae. In: Gullino ML, Munkvold G, editors. Global perspectives on the health of seeds and plant propagation material. Dordrecht (Netherlands): Springer; p. 3–15. doi:10.1007/978-94-017-9389-6_1.
- Sitta J, Nzuve F, Olubayo F, Mutinda C, Muiru WM, Miano D, Muthomi JW, Leley K. 2017. Response of assorted maize germplasm to the maize lethal necrosis disease in Kenya. J Plant Stud. 6(2):65. doi:10.5539/jps.v6n2p65.
- Stevenson W, Hagedorn D. 1973. Further studies on seed transmission of pea seedborne mosaic virus in Pisum sativum. Plant Dis Rep. 57:248–252.
- Storey HH. 1936. Virus diseases of East African plants. East Afr Agric J. 1(4):333–337. doi:10.1080/03670074.1936.11663679.
- Venables WN, Ripley BD. 2002. Modern applied statistics with S, 4th ed. New York, USA: Springer.
- Wamaitha MJ, Nigam D, Maina S, Stomeo F, Wangai A, Njuguna JN, Holton TA, Wanjala BW, Wamalwa M, Lucas T, et al. 2018. Metagenomic analysis of viruses associated with maize lethal necrosis in Kenya. Virol J. 15(1):90. doi:10.1186/s12985-018-0999-2.
- Wang Q, Zhang C, Wang C, Qian Y, Li Z, Hong J, Zhou X. 2017. Further characterization of Maize chlorotic mottle virus and its synergistic interaction with Sugarcane mosaic virus in maize. Sci Rep. 7(1):39960. doi:10.1038/srep39960.
- Wangai AW, Redinbaugh MG, Kinyua ZM, Miano DW, Leley PK, Kasina M, Mahuku G, Scheets K, Jeffers D. 2012. First report of Maize chlorotic mottle virus and maize lethal necrosis in Kenya. Plant Dis. 96(10):1582–1582. doi:10.1094/PDIS-06-12-0576-PDN.
- Xie L, Zhang J, Wang Q, Meng C, Hong J, Zhou X. 2011. Characterization of Maize chlorotic mottle virus associated with maize lethal necrosis disease in China. J Phytopathol. 159(3):191–193. doi:10.1111/j.1439-0434.2010.01745.x.