ABSTRACT
Pear juice concentrate prepared by boiling Japanese pear (Pyrus pyrifolia Nakai cv. Nijisseiki) juice can significantly inhibit the activity of tyrosinase, a key enzyme in melanin synthesis in human skin. Using the ethanol extract of pear juice concentrate, we homogeneously purified an active compound that was identified as 5-hydroxymethyl-2-furaldehyde (5-HMF) through 1H- and 13C-NMR and mass spectroscopy. We observed that 5-HMF inhibited the monophenolase and diphenolase activities of mushroom tyrosinase as a mixed-type inhibitor (Ki values of 3.81 and 3.70 mmol/L, respectively). In B16 mouse melanoma cells, treatment with 170 µmol/L of 5-HMF significantly reduced α-melanocyte-stimulated melanin synthesis by suppressing the cyclic adenosine monophosphate-dependent signaling pathway involved in melanogenesis. The results of our study indicated that 5-HMF can be potentially used as a skin-lightening agent in the cosmetic industry.
Abbreviations: AC: adenylate cyclase; CREB: cAMP response element-binding protein; dhFAME: S-(−)-10,11-Dihydroxyfarnesoic acid methyl ester; DMEM: dulbecco′s modified eagle medium; l-DOPA: 3-(3,4-Dihydroxyphenyl)- l-alanine; GAPDH: glyceraldehyde-3-phosphate dehydrogenase; HEPES: 4-(2-Hydroxyethyl)-1-piperazine ethane sulfonic acid; 5-HMF: 5-Hydroxymethyl-2-furaldehyde; MITF: microphthalmia-associated transcription factor; α-MSH: α-Melanocyte-stimulating hormone; PKA: protein kinase A; PVDF: polyvinylidene difluoride; SDS: sodium dodecyl sulfate; TRP1: tyrosinase-related protein 1; TRP2: tyrosinase-related protein 2
Graphical abstract
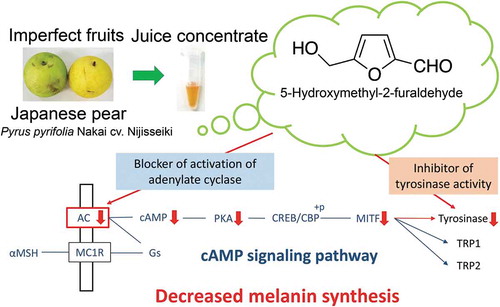
5-Hydroxymethyl-2-furaldehyde can act as a tyrosinase and/or melanogenesis inhibitor, which can be used as a skin-lightening agent in the cosmetic industry.
Melanin protects the skin against ultraviolet radiation-induced injury; however, its dysfunction and accumulation in the skin causes hyperpigmentation disorders, including melasma, freckles, and geriatric pigment spots [Citation1]. Melanin synthesis is controlled by tyrosinase (TYR), tyrosinase-related protein 1 (TRP1), and TRP2 [Citation2]. TYR is a key enzyme that particularly catalyzes the first two steps in melanogenesis: the hydroxylation of l-tyrosine to 3-(3,4-dihydroxyphenyl)- l-alanine (l-DOPA) and the oxidation reaction of l-DOPA to dopaquinone. Several tyrosinase inhibitors have been used as skin-lightening agents in the cosmetics industry [Citation3]. Melanogenesis is mainly regulated by α-melanocyte-stimulating hormone (α-MSH), which binds to the melanocortin receptor 1 on the membrane of skin melanocytes. Moreover, α-MSH continuously activates adenylate cyclase (AC) to form cyclic adenosine monophosphate (cAMP), which functions as a secondary intracellular messenger. cAMP stimulates protein kinase A (PKA) phosphorylation, which induces the expression of the microphthalmia-associated transcription factor (MITF), a master transcription factor in melanin production [Citation4], through cAMP response element-binding protein (CREB) phosphorylation. The upregulation of MITF stimulates TYR, TRP1, and TRP2 expression. To date, several compounds capable of decreasing MITF expression have been isolated from natural resources and subsequently characterized.
Many occidental pear fruits have been reported to contain various phenolic compounds, such as arbutin, chlorogenic acid, rutin, and procyanidins. On another note, oriental pear fruits, including the Japanese pear (Pyrus pyrifolia Nakai cv. Nijisseiki), contain two major phenolic compounds, namely arbutin and chlorogenic acid [Citation5], which have been characterized as antioxidants [Citation6]. The Nijisseiki pear fruit is a major crop in Tottori Prefecture, Japan. However, approximately 10% of the harvested pear fruits are usually discarded as imperfect fruits for being unusually shaped or bruised, or generally unsuitable for commercial use [Citation7]. In the present study, we prepared pear juice concentrate from imperfect Nijisseiki pear fruits and found that the ethanol extract of this concentrate inhibited the activity of TYR. Further, this study investigated the active compound present in this ethanol extract that was responsible for inhibiting TYR activity, described its various properties, studied its inhibitory activity in B16 melanoma mouse cells, and considered its usefulness as a skin-lightening agent in the cosmetics industry.
Materials and methods
Materials
Imperfect Japanese pear (P. pyrifolia Nakai cv. Nijisseiki) fruits were obtained from the Japan agricultural cooperative “JA Tottori Chuo” in Tottori, Japan. Dulbecco′s modified eagle medium (DMEM), phosphate buffer, and trypsin used for cell culture were purchased from Life Technologies Corp. (Carlsbad, CA, USA). Mushroom TYR and synthetic melanin were obtained from Sigma–Aldrich Japan, Inc. (Tokyo, Japan). l-DOPA and α-MSH were purchased from Wako Pure Chemical Industries (Osaka, Japan). Moreover, 5-hydroxymethyl-2-furaldehyde (5-HMF) was acquired from Tokyo Chemical Industry Co., Ltd. (Tokyo, Japan). All other reagents were of the highest commercially available purity. B16 melanoma (murine melanin-producing melanoma, RCB1283) cells were procured from Riken Bio Resource Center (Tsukuba, Japan).
Preparation of Japanese pear juice concentrate and its ethanol extract
Japanese pear fruits were peeled and processes using a juicer (TJ210; Tescom & Co. Ltd., Tokyo, Japan). Pear juice was filtered using a bleached cotton cloth, boiled, and concentrated until approximately 10% of the original volume remained, and used as a Japanese pear juice concentrate. The concentrate (20 mL) was extracted with ethanol (80 mL) using a shaker (AS-1 N; AS ONE, Osaka, Japan) for 1 h to remove the precipitate fraction. The supernatant fraction was evaporated under reduced pressure to remove the ethanol, and the residual solution (5 mL) was dissolved in 45 mL of distilled water. This solution was stored at −30°C and used as an ethanol extract.
Tyrosinase inhibitory assay for the purification of the active compounds
We used a reaction mixture (total volume of 100 μL) containing 40 µL of 0.5 mol/L 4-(2-hydroxyethyl)-1-piperazine ethane sulfonic acid (HEPES) buffer (pH 7.0), 20 µL of 2.5 mmol/L l-DOPA, and 20 μL of a sample solution to determine the diphenolase activity of mushroom TYR. After the mixture was pre-incubated for 3 min at 37°C, the enzymatic reaction was initiated by adding 20 µL of mushroom TYR solution (1.7 units/mL, dissolved in the same HEPES buffer). The reaction mixture was allowed to stand for 5 min at 37°C. Absorbance changes in dopachrome formed from l-DOPA during the experimental period were measured at 475 nm [Citation8] using a Sunrise Rainbow RC-R microplate reader (Tecan Austria GmbH, Salzburg, Austria).
Purification and identification of the tyrosinase inhibitor from Japanese pear juice ethanol extract
Japanese pear juice ethanol extract (6 mL) was applied to an ODS column (1.75 × 14 cm, Cosmosil 140 C18-OPN; Nacalai tesque, Kyoto, Japan) that was washed with 70% (v/v) ethanol and equilibrated with distilled water. Active compounds were eluted from the column with approximately 700 mL of distilled water. Fractions (each 5 mL) were collected using a fraction collector (model 2110; Bio-Rad, Hercules, CA, USA), and the active ones were combined and evaporated under reduced pressure until completely dry. After dissolving the residue in 5 mL of distilled water, the solution subjected to HPLC (20A system; Shimadzu, Kyoto, Japan). The solution (400 µL) was injected onto a reverse-phase HPLC column (Wakosil-II 5C18RS, ϕ 4.0 × 150 mm) and eluted (1.0 mL/min) with 5 (v/v) % methanol containing 0.1 (v/v) % acetic acid at 40°C. Active compounds were monitored by measuring the absorbance at 280 nm. The major peak with a retention time of 8.9 min () was collected, evaporated to dryness under reduced pressure, and purified by HPLC under the same conditions. Then, 10.8 mg of the active compound was obtained and used as a purified compound for further analysis.
Figure 1. Elution patterns of the newly formed tyrosinase inhibitor, arbutin, and chlorogenic acid during HPLC of the ethanol extracts of Japanese pear juice (a) and its boiled concentrate (b).

1H- and 13C-NMR spectra and 2D spectra (COSY, HMQC, and HMBC) were recorded using an Avance II instrument (Bruker, Billerica, MA, USA). ESI–MS data were recorded using a Quattro Micro API mass spectrometer (Waters, Milford, MA, USA) connected to an Acquity UPLC (Waters).
Measurement of diphenolase and monophenolase activities
Commercially available 5-HMF was used for further experimentation. We used a reaction mixture containing 667 µL of 0.1 mol/L HEPES buffer (pH 7.0), 200 µL of various concentrations (1.25–15 mmol/L) of l-DOPA dissolved in the same buffer, and 100 µL of the indicated amounts of 5-HMF to evaluate the effects of different concentrations of 5-HMF on the diphenolase activity of mushroom TYR. The enzymatic reaction was initiated by adding 33 µL of mushroom TYR (1.7 units/mL, dissolved in the same HEPES buffer) after pre-incubating the mixture for 1 min at 30°C. The mixtures were allowed to stand for 2 min, and the absorbance changes during the incubation period were measured at 475 nm [Citation8] using a UV-2550 spectrophotometer (Shimadzu Corp., Kyoto, Japan).
In the case of TYR monophenolase activity, we used a reaction mixture containing 40 µL of the HEPES buffer, 20 μL of different concentrations (0.5–10 mmol/L) of l-tyrosine dissolved in the HEPES buffer, and 20 μL of the indicated amount of 5-HMF. Enzymatic reaction was initiated by adding 20 μL of mushroom TYR (5 units/mL) and allowed to stand for 2 min. Changes in absorbance during the experimental time period were measured at 475 nm [Citation8] using a Sunrise Rainbow RC-R microplate reader (Tecan Austria GmbH).
The Ki values of the diphenolase and monophenolase activities of mushroom TYR were calculated for 5-HMF using the Lineweaver–Burk plot [Citation9].
Cell culture work
Mouse B16 melanoma cells were cultured in DMEM containing 10% (v/v) fetal bovine serum (Biowest, Paris, France) and 1% (v/v) penicillin/streptomycin (Wako Pure Chemical Industries, Osaka, Japan) in an incubator with 5% CO2 at 37°C. The medium was changed every 3 days during cell culture. The B16 cells were cultured in 3 mL of DMEM containing 10 nmol/L α-MSH with 5.0 × 105 cells/dish in a 3.5-cm petri dish. After 1 day, the cells in each dish were treated with 30 µL of the indicated amount of 5-HMF solution (at the final concentrations of 3.4, 34, 170, and 340 µmol/L) and further grown for 3 days. Cell numbers were measured using a TC20™ cell counter (Bio-Rad). Melanin levels were determined as follows: B16 cells were collected through trypsin treatment after washing with PBS. Each cell pellet was dissolved in 1 mL of 1 mol/L NaOH at 80°C for 1 h. The melanin content of each cell lysate was determined by measuring absorbance at 405 nm [Citation10] using the UV-2550 spectrophotometer (Shimadzu). Synthetic melanin was used as the standard.
Quantitative PCR (qPCR)
B16 melanoma cells were cultured under the same aforementioned conditions, collected, and dissolved using a passive lysis buffer (Promega Corp., Madison, WI, USA) on ice for 30 min. The lysate was centrifuged at 10,000 × g for 10 min at 4°C, and the supernatant fraction was subjected to qPCR analysis. Total RNA was extracted from the cell lysate using Sephasol®-RNA1 (Nacalai Tesque), and cDNA was synthesized from the total RNA using PrimeScript™ RT reagent Kit with gDNA Eraser (Takara Bio, Otsu, Japan). The primer pairs used for qPCR analysis were designed using the GENETYX software (GENETYX, Tokyo, Japan) as follows: Tyr, forward, 5′-CAGATCTCTGATGGCCAT-3′, and reverse, 5′-GGATGACATAGACTGAGC-3′; Mitf, forward, 5′-TTATAGTACCTTCTCTTTGCCAGTCC-3′, and reverse, 5′-GTTTATTTGCTAAAGTGGTAGAAAGGTACT-3′; Trp1, forward, 5′-CTTTCTCCCTTCCTTACTGG-3′, and reverse, 5′-TGGCTTCATTCTTGGTGCTT-3′; and Trp2, forward, 5′-TGAGAAGAAACAAAGTAGGCAGAA-3′, and reverse, 5′-CAACCCCAAGAGCAAGACGAAAGC-3′; to yield 18–30 nucleotide sequences with amplification products approximately 100 bp long. A CFX Connect™ Real-Time System (Bio-Rad) with SYBR Premix Ex Taq™ (Takara Bio) was used to perform qPCR. The mRNA levels of glyceraldehyde-3-phosphate dehydrogenase (Gapdh; forward, 5′-GGAGAAGGCTGGGGCTCAT-3′, and reverse, 5′-TGATGGCATGGACTGTGGTC-3′) were used as an internal standard. The qPCR experiments were conducted in triplicate for each cDNA prepared from the three cell preparations.
Western blot analysis
After reaching confluence, the B16 melanoma cells grown under the aforementioned conditions were harvested and dissolved in a passive lysis buffer (Promega Corp.) on ice for 30 min. The solution was centrifuged at 10,000 × g for 10 min at 4°C, and the supernatant fraction was used as a crude enzyme solution for Western blotting. The crude enzyme solution was separated by sodium dodecyl sulfate polyacrylamide gel electrophoresis (SDS–PAGE). We used a precast slab gel (e-PAGEL; model E-R520 L; ATTO Corporation, Tokyo, Japan) for the electrophoresis of samples using a 5%–20% (w/w) linear gradient of polyacrylamide with SDS. After electrophoresis, the proteins were transferred onto a polyvinylidene difluoride (PVDF) membrane (Immuno-Blot PVDF; Bio-Rad) in a Trans-Blot SD semi-dry electrophoretic transfer cell (Bio-Rad). Nonspecific binding was blocked using 5% (w/v) nonfat dry milk in PBS/Tween 20 buffer. The PVDF membranes were probed with an anti-TYR (ab180753; Abcam, Cambridge, MA, USA), anti-MITF (ab20663; Abcam), anti-phosphorylated CREB (serine 133) (ab32096; Abcam), anti-CREB (total CREB) (ab178322; Abcam), and anti-GAPDH (ab8245; Abcam) antibodies. The immunoreactive proteins were detected using an anti-mouse IgG secondary antibody coupled with horseradish peroxidase (ab6721; Abcam) and tetramethylbenzidine, a stabilized substrate for horseradish peroxidase (Promega Corp.), according to the manufacturer’s protocols. Amersham™ ECL™ Rainbow™ Marker-Full Range (GE Healthcare, Minneapolis, MN, USA) was used to determine the molecular mass of the immunoreactive proteins.
Analysis of cAMP-related signaling pathway
The cellular levels of cAMP were quantified using a cAMP enzyme immunometric assay kit (R&D systems, Inc., Minneapolis, MN, USA), according to the manufacturer’s instructions. AC activity was assessed using an AC assay kit (BioVision, Mountain View, CA, USA), according to the manufacturer’s protocols. PKA activity was measured using a PKA kinase assay kit (Enzo Life Sciences, Farmingdale, NY, USA).
Statistical analyses
Cell viability, melanin content, cAMP levels, AC and PKA activities, and mRNA levels of proteins involved in melanogenesis through the cAMP-dependent signaling pathway activated in B16 melanoma cells were analyzed using one-way ANOVA with Tukey′s multiple comparison test. Analyses were performed using GraphPad Prism® for Windows, version 5.03 (GraphPad Software Inc., La Jolla, CA, USA). All data were expressed as mean ± SEM, and differences were considered statistically significant at p < 0.05.
Results
Tyrosinase inhibitory compounds formed in Japanese pear juice concentrate
After Japanese pears were peeled, the juice was squeezed out using a juicer, boiled, and concentrated until approximately 10% of the original volume remained. Pear juice and its boiled concentrate were extracted using ethanol. TYR diphenolase activity was inhibited more strongly in the ethanol extract of the pear juice concentrate (26.4% ± 9.5%, n = 3) that of untreated pear juice (17.4% ± 6.3%, n = 3) when the ethanol extract was diluted with distilled water up to the original volume of the pear juice. HPLC analysis of the original pear juice showed that peaks with retention times of 6.6 and 13.5 min were identical to those of authentic arbutin and chlorogenic acid, respectively ()). However, the pear juice concentrate contained a major compound with a retention time of 8.9 min aside from the peaks of authentic arbutin and chlorogenic acid ()). This major compound had a potent TYR (diphenolase activity) inhibitory activity. The results suggested that the TYR-inhibiting compound was newly formed during pear juice concentrate preparation through boiling.
Purification and identification of the newly formed tyrosinase inhibitor from the ethanol extract of pear juice concentrate
The newly formed TYR inhibitor was purified from the ethanol extract of the pear juice concentrate through ODS-open column chromatography and reverse-phase HPLC. The active compound (10.8 mg) with a retention time of 8.9 min was homogenously purified through a second HPLC.
Positive ESI–MS of the purified compound showed an [M + H]+ ion at m/z 127, indicating that its molecular weight is 126. The 1 H- and 13 C-NMR spectra showed four protons and six carbons, respectively. Thus, the molecular formula of the compound was suggested to be C6H5O2, assuming the presence of one active proton that was not shown in the 1 H-NMR spectrum. shows the 1 H- and 13 C-NMR data of the purified compound in D2O. The hydrogen deficiency index of the purified compound was 4. The 1 H-NMR signal at δH 9.31 ppm and the 13 C-NMR signal at δC180.4 ppm were assigned to a conjugated aldehyde group, whereas the 1 H-NMR signal at δH 4.45 ppm and the 13 C-NMR signal at δC 56.0 ppm were assigned to the methylene group bearing an oxygen atom. The presence of a furan ring was suggested by the remaining signals of the two protons and the four carbons based on their chemical shifts. Two protons (δH 7.40 ppm and δH 6.54 ppm) of the furan ring were connected to carbons with relatively small chemical shifts (δC 126.9 ppm and δC 111.0 ppm) based on the HMQC correlations. Thus, the quaternary carbon signals were assigned to be the 2- and 5- positions of the furan ring, indicating the presence of a 2,5-disubstituted furan ring in the chemical structure. Based on these considerations, this purified compound was identified to be 5-HMF ()). The signals were assigned based on HMBC correlations ()). The spectroscopic data were identical to the reported data [Citation11] and that of the authentic compound.
Table 1. 1 H (500 MHz) and 13 C NMR (150 MHz) data of the purified compound in D2O.
Inhibition of monophenolase and diphenolase activities of mushroom TYR by 5-HMF
The effects of different concentrations of 5-HMF on mushroom TYR monophenolase and diphenolase activities were studied using L-tyrosine and L-DOPA as their respective substrates. Both enzyme activities were significantly dose-dependently inhibited by adding 5-HMF (). The Ki values of monophenolase and diphenolase for 5-HMF were calculated to be 3.70 and 3.81 mmol/L, respectively. The Lineweaver–Burk plots indicated that 5-HMF acted as a mixed-type inhibitor of monophenolase and diphenolase activities.
Figure 3. Lineweaver–Burk double reciprocal plots of the monophenolase and diphenolase activities of mushroom tyrosinase in the presence of 5-hydroxymethyl-2-furaldehyde. Absorbance-change rate (v) was measured using different concentrations of L-tyrosine and L-DOPA as respective substrates for monophenolase (a) and diphenolase (b) activities. 5-Hydroxymethyl-2-furaldehyde was used at concentrations of 0 (●), 2.46 (■), 4.92 (▲), and 9.80 mmol/L (▼) for monophenolase activity and was at concentrations of 0 (●), 1.23 (■), 2.46 (▲), and 4.92 mmol/L (▼) for diphenolase activity. Data were obtained from three independent experiments.

5-HMF significantly reduced α-MSH-induced melanin synthesis in B16 melanoma cells
When B16 melanoma cells were grown in the presence of the indicated amounts of 5-HMF for 3 days, significant cytotoxicity was observed by adding 340 µmol/L 5-HMF but not 170 µmol/L 5-HMF ()). Therefore, in the following experiments, 5-HMF at a final concentration of 170 µmol/L was used.
Figure 4. Effects of 5-hydroxymethyl-2-furaldehyde on α-MSH-induced melanin synthesis in B16 mouse melanoma cells. (a) Cell viability; (b) melanin content. All experiments were conducted in triplicate. Data are expressed as percentage of the values in the cells treated without 5-hydroxymethyl-2-furaldehyde. Values are presented as mean ± SEM from three independent experiments. Different letters indicate statistically significant values (p < 0.05).

Melanin levels were significantly higher in B16 melanoma cells treated with 10 nmol/L of α-MSH than those in the control cells treated without α-MSH. When B16 melanoma cells were grown in a medium with 170 µmol/L of 5-HMF, α-MSH-induced melanin production was significantly reduced to approximately 48.4% compared with that in cells treated without 5-HMF ()). These results suggested that 5-HMF at a concentration of 170 µmol/L acted as a α-MSH-induced melanin production inhibitor in B16 melanoma cells.
Changes in the mRNA and protein levels of modulators and enzymes involved in cAMP-dependent melanogenesis pathway by 5-HMF
The mRNA levels of Tyr, Mitf, Trp1, and Trp2 were determined in the homogenates of B16 melanoma cells treated with different amounts of 5-HMF with α-MSH. Furthermore, 5-HMF at a concentration of 170 µmol/L significantly decreased the expression of mRNAs encoding Tyr and Mitf (,b)) but did not decrease those of Trp1 and Trp2 (,d)). Thus, TYR and MITF protein levels were decreased by adding 170 µmol/L of 5-HMF ().
Figure 5. Effects of different amounts of 5-hydroxymethyl-2-furaldehyde on the mRNA expression of tyrosinase, tyrosinase-related proteins, and microphthalmia-associated transcription factor in the homogenates of cells treated with α-MSH. Using qPCR system, we determined the levels of mRNAs encoding tyrosinase (Tyr) (a), microphthalmia-associated transcription factor (Mitf) (b), tyrosinase-related protein 1 (Trp1) (c), and tyrosinase-related protein 2 (Trp2) (d). Data are expressed as percentages of the values in the cells treated without 5-hydroxymethyl-2-furaldehyde. Values are presented as mean ± SEM of three independent experiments, and the different letters indicate significant values (p < 0.05).

Figure 6. Western blot analysis of tyrosinase (TYR) and microphthalmia-associated transcription factor (MITF) proteins in the homogenate of B16 melanoma cells treated with 5-hydroxymethyl-2-furaldehyde. The crude enzyme solution was separated by SDS-PAGE. After electrophoresis, the proteins were transferred onto a PVDF membrane. TYR, MITF, and GAPDH (as loading control) proteins on PVDF membranes were probed and visualized with goat polyclonal anti-TYR, -MITF, and -GAPDH antibodies. Data are presented as typical immunoreactive patterns of the proteins from three independent Western blot analyses of cells.

AC activity that was stimulated by adding α-MSH significantly decreased in the B16 melanoma cells treated with 170 µmol/L 5-HMF, and cAMP levels significantly reduced to the control level (,b)). The B16 melanoma cells treated with 170 µmol/L 5-HMF showed that the increased PKA activity by adding α-MSH significantly decreased to the control levels ()). Although 170 µmol/L of 5-HMF did not affect the total CREB level in the B16 melanoma cells with or without α-MSH, it decreased the immunoreactive component of the phosphorylated CREB stimulated by α-MSH ()). These results indicate that 5-HMF significantly decreased TYR mRNA and protein levels by inhibiting AC activation, leading to the suppression of the cAMP-dependent signaling pathway of melanogenesis.
Figure 7. Effects of different amounts of 5-hydroxymethyl-2-furaldehyde on the cAMP-dependent signaling pathway in B16 mouse melanoma cells treated with α-MSH. cAMP levels (a), AC activity (b), PKA activity (c), and the protein levels of total CREB and phosphorylated CREB (p-CREB) (d). Total and p-CREB levels were detected with an anti-total CREB and an anti-p-CREB antibodies, respectively, through Western blotting. Data are expressed as the percentages of the values in the cells treated without 5-hydroxymethyl-2-furaldehyde. Values are presented as mean ± SEM of three independent experiments, and the different letters indicate statistically significant values (p < 0.05).

Discussion
In this study, we have described the various properties of 5-HMF as a TYR and/or melanogenesis inhibitor, and the usefulness of this compound as a skin-lightening agent manufacturing cosmetic products. We boiled Japanese pear juice to obtain a concentrate of arbutin (approximately 10 times greater) and we found that an ethanol extract of the concentrate inhibited TYR activity. However, preliminary experiments indicated that the major TYR inhibitory compound was not derived from arbutin, suggesting that another new active compound was derived during pear juice concentrate preparation. We found that the pear juice concentrate contained a newly formed active compound, which was homogeneously purified from the ethanol extract and identified as 5-HMF using 1 H- and 13 C-NMR and mass spectroscopy. This compound is reportedly formed by non-enzymatic fructose and glucose dehydration with amino acids [Citation12]. The Japanese pear juice concentrate used in this experiment contained approximately 614 mg/L of 5-HMF, and this compound has been reportedly found at different concentrations of ~1000 mg/L in many food products, such as wine, spirits, and prune juices [Citation13]. To the best of our knowledge, the present study is the first to show that 5-HMF can act as a TYR inhibitor, although 5-HMF has been characterized as an antioxidant [Citation14,Citation15]. We characterized 5-HMF as a TYR inhibitor using mushroom TYR. TYR (EC 1.14.18.1) is an enzyme that catalyzes two different reactions as follows: orthohydroxylation of monophenols to o-diphenols (monophenolase activity) and oxidation of o-diphenols to their corresponding o-quinones (diphenolase activity) [Citation16]. In the present study, we found that TYR monophenolase and diphenolase activities were significantly inhibited by adding 5-HMF, which acted as a mixed-type inhibitor to both enzymes’ activities.
Many TYR inhibitors have been identified and characterized. Mixed-type inhibitors of diphenolase activity were reportedly identified as arabinose (Ki value, 0.22 mmol/L), terephthalic acid (Ki value,11.01 mmol/L), and piperonylic acid (Ki value, 0.21 mmol/L) [Citation17–Citation19]. Mixed-type inhibitors of monophenolase activity, such as haginin A (Ki value, 1.5 µmol/L), kurarinol (Ki value, 14 mmol/L), and kuraridinol (Ki value, 0.39 µmol/L), have also been previously reported [Citation20,Citation21]. Cycloalliin and 4-chlorosalicylic acid have also been reported to be mixed-type inhibitors of both monophenolase and diphenolase activities [Citation22,Citation23]. Based on the Ki values of these compounds, 5-HMF proved to be a weak inhibitor of TYR.
To the best of our knowledge, there is no information on whether 5-HMF can act as a TYR inhibitor in vivo, and whether skin exposure to 5-HMF induces harmful effects and damages on human health is still unclear. Severin et al. [Citation24] have described that 5-HMF induced weak DNA damage to HepG2 cells only at high concentrations (7.9–25 mmol/L) using the comet assay. These observations suggested that high levels of 5-HMF induced weak cytotoxicity to mammalian cells. However, 170 µmol/L of 5-HMF did not show any cytotoxicity to the B16 melanoma cells, but significantly reduced α-MSH-induced melanin formation.
Kim et al. [Citation25] have reported that cystamine (decarboxylated cystine) significantly reduced the protein expressions of MITF, TYR, and TRP2 levels in human melanoma cells (SK-MEL-2), but not TRP1. Similar results were reported in glyceollin (an isoflavonoid phytoalexin isolated from soybean) [Citation26]. Although the addition of 100 µmol/L of S-(-)-10,11-dihydroxyfarnesoic acid methyl ester (dhFAME) significantly decreased the mRNA levels of Mitf, Tyr, Trp1, and Trp2 in murine melanocyte cells, 50 µmol/L dhFAME-treated cells showed only reduced Mitf mRNA expression [Citation27]. Thus, different degrees of Mitf mRNA expression induced varied changes in the mRNA expressions of Trp1 and Trp2 that are involved in melanin synthesis, which may be dependent on the cell types or the experimental conditions used. In the present study, 5-HMF significantly decreased the expression of mRNAs encoding Tyr and Mitf, but not those of Trp1 and Trp2 ().
The present study also shows that 170 µmol/L 5-HMF-treated B16 melanoma cells significantly decreased the cellular cAMP level by inhibiting α-MSH-stimulating AC activation. Consequently, PKA activity was significantly reduced in 5-HMF-treated cells. Potent inhibitors of AC, such as chrysin (at 10–100 µmol/L) and coumarin (at more than 30 µmol/L), have been reported to block AC activation by stimulating α-MSH, thereby decreasing melanin synthesis in B16 melanoma cells [Citation28,Citation29]. Similar results were obtained with 5-HMF in the present study.
In conclusion, our findings suggest that because 5-HMF has a potent ability of inhibiting both TYR activity and mRNA expression, it can be considered as a potential skin-lightening agent that can be used in manufacturing cosmetic products.
Author contributions
T. B., K.K., R.A., N.U., and T.Y. performed most of the experiments. T.I. and A.I. contributed to the identification of 5-hydroxymethyl-2-furaldehyde. T.B., Y.Y., K.W., and F.W. designed the experiments and interpreted the results. T.B., A.I., and F.W. wrote the manuscript. All authors commented on the manuscript and approved the final version.
Disclosure statement
No potential conflict of interest was reported by the authors.
References
- Kim YJ, No JK, Lee JH, et al. 4,4′-dihydroxybiphenyl as a new potent tyrosinase Inhibitor. Biol Pharm Bull. 2005;28(2):323–327. .
- Kameyama K, VJ H. The expression of tyrosinase, tyrosinase-related proteins 1 and 2 (TRP1 and TRP2), the silver protein, and a melanoma cells of differing melanogenic activities. Pigment Cell Res. 1995;8:97–104.
- Loizzo MR, Tundis R, Menichini F. Natural and synthetic tyrosinase inhibitors as antibrowning agents. An update. Com Rev Food Sci Food Saf. 2012;11(4):378–398.
- Chang TS. Natural melanogenesis inhibitors acting through the down-regulation of tyrosinase activity. Materials. 2012;5(9):1661–1685.
- Cui T, Nakamura K, Ma L, et al. Analyses of arbutin and chlorogenic acid, the major phenolic constituents in oriental pear. J Agric Food Chem. 2005;53:3882–3887.
- Galvis-Sánchez A, Gil-lzquierdo A, Gil MI. Comparative study of six pear cultivars in terms of their phenolic and vitamin C contents and antioxidant capacity. J Sci Food Agric. 2003;83(10):995–1003.
- Ministry of Agriculture, Forestry and Fisheries (MAFF) of Japan. Statistical survey on crops. 2005. http://www.maff.go.jp/j/tokei/kouhyou/sakumotu/Japanese.
- Aroca P, Garcia-Borron JC, Solano F, et al. Regulation of mammalian melanogenesis I: partial purification and characterization of a dopachrome converting factor: dopachrome tautomerase. Biochim Biophys Acta. 1990;1035:266–275.
- Lineweaver H, Burk D. The determination of enzyme dissociation constants. J Am Chem Soc. 1934;56:658–666.
- Fu T, Chai B, Shi Y, et al. Fargesin inhibits melanin synthesis in murine malignant and immortalized melanocytes by regulating PKA/CREB and P38/MAPK signaling pathways. J Dermatol Sci. 2019;94(1):213–219. .
- Khan H, Saeed M, Khan MA, et al. Antimalarial and free radical scavenging activities of rhizomes of Polygonatum verticillatum supported by isolated metabolites. Med Chem Res. 2012;21:1278–1282.
- Antal MJ, Leesomboom T, Mok W, et al. Mechanism of formation of 2-furaldehyde from D-xylose. Carbohydr Res. 1991;271:71–85.
- Van-Gorsel H, Li C, Kerbel EL, et al. Compositional characterization of prune juice. J Agric Food Chem. 1992;40:784–789.
- Kulkarni A, Suzuki S, Etoh H. Antioxidant compounds from Eucalypus grandis biomass by subcritical liquid water extraction. J Wood Sci. 2008;54(2):153–157.
- Talcott ST, Percival SS, Pittet-Moore J, et al. Phytochemical composition and antioxidant stability of fortified yellow passion fruit (Passiflora edulis). J Agric Food Chem. 2003;51:935–941.
- García-Molna F, Muñoz JL, Varón R, et al. A review on spectrophotometric methods for measuring the monophenolase and diphenolase activities of tyrosinase. J Agric Food Chem. 2007;55:9739–9749.
- Hu WJ, Yan L, Park D, et al. Kinetic, structural and molecular docking studies on the inhibition of tyrosinase induced by arabinose. Int J Biol Macromol. 2012;50:694–700.
- Yin SJ, Si YX, Chen YF, et al. Mixed-type inhibition of tyrosinase from Agaricus bisporus by terephthalic acid: computational simulations and kinetics. Protein J. 2011;30:273–280.
- Si YX, Ji S, Fang NY, et al. Effects of piperonylic acid on tyrosinase: mixed-type inhibition kinetics and computational simulations. Process Biochem. 2013;48:1706–1714.
- Kim JH, Baek SH, Kim DH, et al. Downregulation of melanin synthesis by Haginin A and its application to in vivo lightening model. J Invest Dermatol. 2008;128:1227–1235.
- Hyun SK, Lee WH, Jeong DM, et al. Inhibitory effects of kurarinol, kuraridinol, and trifolirhizin from Sophora flavescens on tyrosinase and melanin synthesis. Biol Pharm Bull. 2008;31:154–158.
- Bito T, Koseki K, Moriguchi T, et al. Cycloalliin inhibits melanin biosynthesis in B16 mouse melanoma cells. Food Sci Technol Res. 2018;24:627–633.
- Han P, Chen CQ, Zhang CL, et al. Inhibitory effects of 4-chlorosalicylic acid on mushroom tyrosinase and its antimicrobial activities. Food Chem. 2008;107:797–803.
- Severin I, Dumont C, Jondeau-Cabaton A, et al. Genotoxic activities of the food contaminant 5-hydroxymethylfurfural using different in vitro bioassays. Toxicol Lett. 2010;192:189–194.
- Kim HJ, Lee HJ, Park MK, et al. Involvement of transglutaminase-2 in α-MSH-induced melanogenesis in SK-MEL-2 human melanoma cells. Biomol Ther. 2014;22:207–212.
- Lee YS, Kim HK, Lee K, et al. Inhibitory effect of glyceollin isolated from soybean against melanogenesis in B16 melanoma cells. BMB Rep. 2010;43:461–467.
- Baek SH, Ahn JW, Nam SH, et al. S-(-)-10,11-dihydroxyfarnesonic acid methyl ester inhibits melanin synthesis in murine melanocyte cells. Int J Mol Sci. 2014;15:12750–12763.
- Kim DC, Rho SH, Shin JC, et al. Inhibition of melanogenesis by 5,7-dihydroxyflavone (chrysin) via blocking adenylyl cyclase activity. Biochem Biophys Res Commun. 2011;411:121–125.
- Kim DC, Rho SH, Kim D, et al. Coumarin, a lead compound of warfarin, inhibits melanogenesis via blocking adenylyl cyclase. Am J Biomed Res. 2013;1:43–47.