ABSTRACT
Protein-containing nutrients result in the efficient hypertrophy of muscles by increasing muscle protein synthesis. Soybean is often ingested by athletes or individuals who exercise; however, it takes very long to be absorbed. Lactic acid-fermented and enzyme-digested (LFED) soybean is absorbed faster than untreated soybean. This study aims at determining muscle protein synthesis after ingesting a single bolus of soybean or LFED soybean produced by lactic acid bacteria and protease digestion. Eight-week-old overnight-fasted ICR mice were administered powdered or LFED soybean. Mice were euthanized at 7, 15, 30, 60, 90, and 120 min after soybean intake. We have demonstrated that LFED soybean administration was quicker in stimulating muscle protein synthesis by activating mammalian target of rapamycin (mTOR) signaling than orally ingesting untreated soybean in the gastrocnemius muscle. These results suggested that LFED soybean is a more efficient source of nutrition for muscle hypertrophy than untreated soybean.
GRAPHIC ABSTRACT
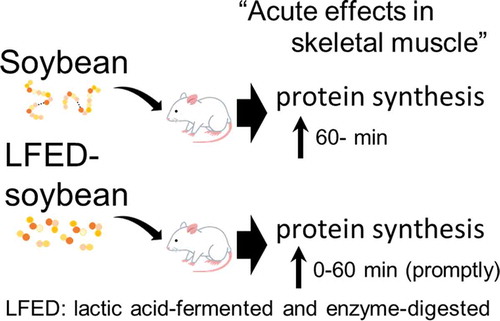
Schema for acute effects of lactic acid-fermented and enzyme-digested soybean on protein synthesis via mTOR signaling in the skeletal muscle
Increased muscle mass improves physical strength and performance [Citation1,Citation2]. The accretion or loss in muscle mass is regulated by balanced protein synthesis and degradation [Citation3]. Muscle hypertrophy occurs when the rate of muscle protein synthesis exceeds that of degradation [Citation3]. Muscle protein synthesis is stimulated by ingesting proteins as well as resistance training [Citation3–Citation5].Mammalian target of rapamycin (mTOR) is influenced by a wide range of stimuli, such as mechanical stress, growth factors, hormones, and nutrients, to regulate protein synthesis [Citation3–Citation5]. mTOR signaling is the main pathway that results in increased muscle protein synthesis [Citation4–Citation10]. Ingesting amino acids (the smallest unit constituting proteins) promotes mTOR signaling [Citation11,Citation12]. mTOR induces the phosphorylation of the ribosomal protein S6 (rpS6), which is a downstream target of mTOR signaling and increases muscle protein synthesis [Citation13,Citation14]. Therefore, muscle protein synthesis induced by the ingestion of amino acids is regulated by mTOR that senses intracellular amino acids, thereby enhancing the activation of rpS6 [Citation11–Citation14].
Soybean contains an abundance of amino acids and affects muscle hypertrophy via the activation of mTOR signaling [Citation15,Citation16]. In addition, soybean is often used in combination with resistance training [Citation16]. Whey and casein are also frequently used [Citation17]. Protein synthesis depends on the digestion rate of proteins [Citation17]. In addition, whey was digested faster than casein [Citation17]. However, since these animal proteins are rich in cholesterol and neutral fats, large quantities are harmful to daily intake. In contrast, soybean has a low saturated fatty acid content without any cholesterol [Citation17]. Furthermore, soybean contains a single homogeneous protein fraction, which is digested in a manner more similar to whey than casein [Citation15,Citation16]. Therefore, soybean is healthy and nutritious. However, soybean is digested and absorbed slowly as compared to other proteins; moreover, ingesting soybean after training is not sufficiently nutritious for muscle hypertrophy [Citation16]. Therefore, there is a need to resolve these issues and develop soybean as an effective supplement for muscle hypertrophy.
Using lactic acid bacteria and protease generates lactic acid-fermented and enzyme-digested (LFED) soybean that has a lower molecular weight than untreated soybean. Fermentation (and enzymatic digestion) degrades proteins into peptides and amino acids that enable faster rates of digestion and absorption than high molecular weight proteins [Citation18,Citation19]. Muscle protein synthesis is associated with the rate of digestion and absorption of proteins in the acute phase after ingestion [Citation20,Citation21]. Therefore, using LFED soybean could bypass the limitations associated with untreated soybean and be an effective nutritive intervention for muscle hypertrophy. However, the differences in acute changes in muscle protein synthesis upon ingesting LFED versus untreated soybean remains unclear. Thus, this study aims at evaluating the acute effects of protein synthesis in skeletal muscle upon the ingestion of LFED and untreated soybean.
Material and methods
Animals
A total of 36 male ICR mice (seven-week-old) for protein synthesis experiment and 84 mice (seven-week-old) for Western blot analysis were obtained from Japan SLC (Hamamatsu, Japan). All the mice were housed for 1 week in an environment maintained at 22 ± 2°C with a 12 h light-dark cycle; feed and water were freely available. This study was approved by the Institutional Animal Care and Use Committee and was performed according to the Kobe University Animal Experimentation Regulations (No. P130903-R2). All experiments were conducted in accordance with the National Institutes of Health Guidelines for the Care and Use of Laboratory Animals (National Research Council, 1996).
Administering soybean and sample collection
LFED soybean and untreated soybean (Mitake Food, Toda, Japan) were used in the present study. LFED soybean was prepared as following; 10% soybean powder (Mitake Food) and 0.1% glucose were dissolved in water and the soybean solution was sterilized at 90 °C for 30 min. The solution was then fermented with Pediococcus acidilactici strain R037 at 37°C for 3-h and digested with enzyme (Amano Enzyme, Nagoya, Japan) at 60°C for 1-h, and was freeze-dried. Coomassie Brilliant Blue stained sodium dodecyl sulfate (SDS)- polyacrylamide gel confirmed that the molecular weight of LFED soybean was lower than that of untreated soybean (). We performed a time-course analysis of the signaling and muscle protein synthesis after administering untreated (Soy group) or LFED soybean (LFED-Soy group) following an 18 h fast as described below. The Soy and LFED-Soy groups were administered untreated or LFED soybean (3 g/kg body weight in 5% aqueous solution of gum arabic) (), respectively by oral gavage. The volume of soybean solution administered was 1 mL per 100 g of body weight. For determination of phosphorylated mTOR and rpS6 expression, the animals (n = 6 per each measured time point) were euthanized 0, 7, 15, 30, 60, 90, or 120 min after administering untreated soybean or LFED-soybean. For protein synthesis experiment, the animals (n = 6 per each measured time point) were euthanized 0, 30, 60 min after administering untreated soybean or LFED-soybean. The gastrocnemius muscle was removed, weighed (wet weight), immediately frozen in dry ice-cooled isopentane, and stored at −80°C until further analysis.
Table 1. Compositions of the experimental diets.
Muscle protein synthesis
Muscle protein synthesis was measured using the in vivo surface sensing SUnSET method [Citation4,Citation22]. Briefly, puromycin (WAKO, Osaka, Japan; 0.04 µmol/g body weight) was diluted using a 0.02 mol/L phosphate buffered saline (PBS) solution and intraperitoneally injected into the mice after 5 min of anesthesia by an i.p. injection of sodium pentobarbital (40 mg/kg) after which muscle was removed exactly 15 min after administering puromycin. The muscle samples from administering untreated soybean after 0 min, 30 min and 60 min mice were used. Portions (approximately 20 mg) of frozen tissue samples were homogenized in ice-cold homogenizing buffer containing 20 mM Tris-HCl (pH 7.5), 1% NP40, 1% sodium deoxycholate, 1 mM ethylenediaminetetraacetic acid (EDTA), 1 mM ethylene glycol tetraacetic acid (EGTA), 150 mM NaCl, and protease and phosphatase inhibitor cocktail (Sigma Aldrich, St. Louis, MO, USA) according to the buffer contents described by Ogasawara et al. [Citation4] and centrifugation at 2,000 × g for 3 min at 4°C, the supernatant was collected and processed for Western blotting. A mouse monoclonal anti-puromycin antibody (Merck Millipore, Billerica, MA, USA) was used to detect the incorporation of puromycin (sum of the intensities of all the protein bands from the Western blot). The blots were analyzed and quantified by the Multi-Gauge Image Analysis Software (Fujifilm). The total protein synthesis was calculated by accumulated 30 min and 60 min of band intensities. All blots were stained with Coomassie Blue (Nacalai Tesque, Kyoto, Japan) to verify equal sample loading ()). The intensity of puromycin were normalized to the corresponding intensity of Coomassie Brilliant Blue staining.
Figure 2. Representative images for the blot probed with antibodies against puromycin and Coomassie Blue-stained membrane (a). Puromycin-labeled peptides are shown for each condition (b) Soy, untreated soybean (total: n = 18, 0 min: n = 6, 30 min: n = 6, 60 min: n = 6) after administration of untreated soybean; LFED-Soy, Lactic acid-fermented and enzyme-digested soybean (total: n = 18, 0 min: n = 6, 30 min: n = 6, 60 min: n = 6) after administration of lactic acid-fermented and enzyme-digested soybean. Total amount of proteins at 30- and 60-min phases (c). Soy: accumulated Soy groups band intensity at 30- and 60-min phases; LFED-Soy: accumulated LFED-Soy groups band intensity at 30- and 60-min phases. Data are relative to the control group and represent the mean ± SEM. In Figure 2(b), * and † indicate significant differences (P < 0.05) as compared to the Soy group, 0 min time-point of the same group, 30 min time-point of the same group, respectively (* vs. 0 min, † vs. 30 min). a is significantly difference from Soy group at p < 0.05 in panel (b) (a vs. Soy group). In Figure 2(c), * indicates significant differences (P < 0.05) as compared to the Soy group.

Western blotting
We determined the levels of phosphorylated mTOR (Ser2448) and rpS6 (Ser235/236) as indicators of active mTOR signaling [Citation4,Citation23] by Western blotting as reported previously [Citation4,Citation24]. In brief, portions (approximately 20 mg) of frozen tissue samples were homogenized in a homogenization buffer containing 20 mM Tris-HCl (pH 7.5), 1% NP-40, 1% sodium deoxycholate, 1 mM ethylenediaminetetraacetic acid (EDTA), 1 mM ethylene glycol tetraacetic acid (EGTA), 150 mM sodium chloride, and protease and phosphatase inhibitor cocktail (1:100, P8340; Sigma Chemicals, Perth, WA, USA). The homogenates obtained were centrifuged at 15,000 rpm at 4°C for 10 min and supernatants were collected. The total protein concentration of the supernatants was measured using a Bradford-based [Citation25] protein assay kit (Bio-Rad Laboratories, Hercules, CA, USA) before loading onto 5% or 12.5% SDS-polyacrylamide gels. The separated proteins were blotted onto polyvinylidene difluoride membranes and blocked for 1 h with 3% bovine serum albumin (BSA) in Tris-buffered saline (TBS) containing 0.1% Tween 20 (TBST). The membranes were then washed 3 times with TBST and incubated overnight at 4°C with primary antibodies, namely phosphorylated (phospho) mTOR (Ser2448, cat. no. 2971, Cell Signaling, Beverly, Massachusetts), total mTOR (cat. no. 4517, Cell Signaling), phospho-rpS6 (Ser235/236, cat. no. 2211, Cell Signaling), and total rpS6 (cat. no. 2317, Cell Signaling). The membranes were washed again in TBST and incubated for 1 h at room temperature with the respective secondary antibodies. Protein bands were detected using EzWestLumi One (ATTO, Tokyo, Japan) and imaged using a luminescent image analyzer (LAS-1000, Fujifilm, Tokyo, Japan). The blots were analyzed and quantified by the Multi-Gauge Image Analysis Software (Fujifilm). Glyceraldehyde 3-phosphate dehydrogenase (GAPDH, cat. no. 97166, Cell Signaling) was used as an internal control. The total phosphorylated mTOR/total mTOR and phosphorylated rpS6/total rpS6 were calculated by accumulated from 0 min to 120 min of each band intensity.
Statistical analyzes
All data have been represented as mean ± standard error of the mean (SEM). The Student’s t-test was used to compare the total protein synthesis, total phosphorylated mTOR/total mTOR and phosphorylated rpS6/total rpS6 among the Soy and LFED soybean groups. Two-way ANOVA was used analyses the protein synthesis and western blot protein expression levels among the Soy and LFED soybean groups followed by Tukey’s post-hoc tests to determine specific group differences. The level of significance was set at P < 0.05.
Results
Body mass and absolute and relative gastrocnemius muscle mass
There were no significant differences upon administering untreated or LFED soybean in each group ().
Table 2. Body mass and absolute and relative gastrocnemius muscle mass.
Muscle protein synthesis
Muscle protein synthesis at 0 min in the Soy group did not change as compared to at 30 min of administering untreated soybean, whereas muscle protein synthesis at 0 min in the LFED-Soy was higher than that at 30 min ()). Muscle protein synthesis at 60 min in the Soy group was higher than that at 30 min. Orally administering the LFED soybean increased the muscle protein synthesis compared to that at 30 min in Soy group, whereas there was no significantly different between two groups at 60 min. Moreover, the total amount of puromycin-labeled peptides at two time-points was higher in LFED-Soy group than that in the Soy group ()).
mTOR signaling
Representative images of Western blots for mTOR and rpS6 in the gastrocnemius muscle are shown in ). Phosphorylated mTOR levels increased and reached saturation at 30 min after orally administering untreated soybean ()). In comparison, phosphorylated mTOR levels were elevated within 7 min and reached saturation within 7–30 min after orally administering LFED soybean. The LFED-Soy group had higher phospho-mTOR levels than the Soy group between 7–15 min after oral administration. The total amount of phospho-mTOR levels from 0 min to 120 min was higher in LFED-Soy group than that in the Soy group ()).
Figure 3. Representative images for the blot probed with antibodies against mTOR, rpS6 and GAPDH (a).Time-dependent changes in the levels of phospho-mammalian target of rapamycin (mTOR) Ser2448 after administering untreated and lactic acid-fermented and enzyme-digested soybean (b). Time-dependent changes in the levels of phospho-ribosomal protein S6 (rpS6) Ser235/236 after administering untreated and lactic acid-fermented and enzyme-digested soybean (c). Soy, untreated soybean (total: n = 42, 0 min: n = 6, 7 min: n = 6, 15 min: n = 6, 30 min: n = 6, 60 min: n = 6, 90 min: n = 6, 120 min: n = 6); LFED-Soy, Lactic acid-fermented and enzyme-digested soybean (total: n = 42, 0 min: n = 6, 7 min: n = 6, 15 min: n = 6, 30 min: n = 6, 60 min: n = 6, 90 min: n = 6, 120 min: n = 6). Total amount of phospho-mTOR levels (d) and phospho-mTOR levels (e) from 0- to 120-min phases. Soy: accumulated Soy group band intensities; LFED-Soy: accumulated LFED-Soy group band intensities. Data have been represented as mean ± SEM. p indicates phosphorylated and t indicates total protein. * indicates significant differences (P < 0.05) as compared to 0 min time-point of the same group (* vs. 0 min). a is significantly difference from Soy group at p < 0.05 (a vs. Soy group). In Figure 3(d,e), * indicates significant differences (P < 0.05) as compared to the Soy group (* vs. Soy group).

Phosphorylated rpS6 levels remained constant within the first 7 min of orally administering untreated soybean (). After 7 min, phospho-rpS6 levels were gradually elevated and reached saturation at 60 min. In contrast, after orally administering LFED soybean, phospho-rpS6 levels increased and reached saturation within 15 min and were maintained within 15–30 min before returning to basal levels by 90 min. The LFED-Soy group had higher phospho-rpS6 levels than the Soy group between 15–30 min after oral administration. The total amount of phospho-rpS6 levels from 0 min to 120 min was higher in LFED-Soy group than that in the Soy group ()).
Discussion
A single dose of soybean (untreated and LFED) increased muscle protein synthesis in mice. LFED soybean was more potent in promptly increasing muscle protein synthesis by activating mTOR signaling faster than untreated soybean. Therefore, LFED soybean is a more effective form of nutrition for promptly increasing muscle protein synthesis as compared to untreated soybean.
In this study, ingesting both untreated and LFED soybean led to an increase in muscle protein synthesis. After the soybean was ingested, the activation of the mTOR signaling could be immediately observed followed by that of rpS6. The peak of the rpS6 activation was consistent with muscle protein synthesis. The mTOR signaling is one of the major pathways involved in muscle protein synthesis that is induced by ingesting amino acid-containing nutrients [Citation4,Citation23,Citation26]. Therefore, the increase in muscle protein synthesis by ingesting both the untreated and LFED soybean might have resulted from the mTOR-mediated rpS6 activation that is in good agreement with previous reports [Citation27,Citation28].
The differences in the effect of the LFED and untreated soybean is due to their absorption kinetics, wherein high molecular weight proteins are absorbed slower than low molecular weight proteins [Citation18]. Proteins that are ingested orally are digested, absorbed, and transported into the blood in an amino acid form [Citation5,Citation29]. Notably, low molecular weight proteins, peptides, and amino acids are absorbed directly and quicker than high molecular weight proteins via the peptide transporter in the small intestine [Citation19]. Maebuchi et al. reported that low molecular weight soybean increased the plasma amino acid concentration faster than untreated soybean [Citation30]. A high plasma amino acid concentration enables the amino acid uptake by the muscle. These amino acids are stored in the free amino acid pool in the skeletal muscle [Citation5,Citation29]. A large free amino acid pool in the muscle leads to the activation of the mTOR signaling [Citation6,Citation29]. Moreover, protein digestion and absorption rates modulate the postprandial muscle protein synthesis [Citation21]. In this study, the total amount of the phosphorylated mTOR and rpS6 from 0 min to 120 min were significantly higher in the LFED-Soy group than in the Soy group presented. Thus, ingesting LFED soybean enhanced muscle protein synthesis by activating the mTOR pathway faster than the untreated soybean. These results suggested that, compared to untreated soybean, ingesting LFED soybean leads to faster and better digestion and absorption, and it activates all the components of the mTOR signaling for muscle protein synthesis.
In this study, ingesting LFED soybean increased muscle protein synthesis promptly compared to untreated soybean. These striking differences between ingesting untreated and LFED soybean could be attributed to their different digestion and absorption characteristics, as mentioned above. The rapid increase in the plasma amino acid levels supports this hypothesis, which enhances muscle protein synthesis and anabolic signaling significantly more than small pulses of an identical amount of protein [Citation21]. Therefore, our findings suggest that ingesting LFED soybean benefits the quantity and quality of quickly increasing muscle protein synthesis compared to untreated soybean. Muscle hypertrophy is strongly associated with the muscle protein synthesis rate [Citation3,Citation31]. An increase in muscle protein synthesis induces muscle hypertrophy to a greater extent [Citation3,Citation31]. It has been suggested that an exercise-induced muscle protein synthesis increase occurs rapidly for 60 min [Citation32]. In addition, protein synthesis with nutrition support after exercise training exhibited a higher level of increase than without nutrition support [Citation33]. We found that ingesting LFED soybean induced a quick increase in muscle protein synthesis until 60 min in the present study. Thus, combined exercise and ingesting LFED soybean after exercise might result in effective muscle hypertrophy via leading to the synergistic effects of protein synthesis.
Nevertheless, this study also has several limitations. Previous studies suggested that the level of protein synthesis changed together with the phosphorylated rpS6 expression at approximately the same time during the acute phase [Citation34,Citation35]. The protein synthesis rate was measured until 60 min after the administration of soybean in the present study. The phosphorylated rpS6 expression level in the Soy group reached a peak at 60 min and decreased slowly compared to the LFED-soy group. Therefore, it would be possible that the total protein synthesis in the Soy group might reach a peak at 60 min and decrease slowly compared to the LFED-soy group. However, it could not be clarified as not the total protein synthesis was not measured for 60 min. Hence, further studies would be required to clarify if an increasing total protein synthesis could occur subsequently beyond 60 min in the Soy and LFED-soy groups.
In addition, the LFED-soybean dose could be potentially too high in humans. Therefore, further studies would be required to clarify the appropriate dose for humans.
A previous study suggested that protein ingestion, such as that of soy protein, induced increased blood insulin levels [Citation36]. Insulin is a regulator of increased protein synthesis via the PI3 K/Akt/mTOR pathway [Citation37]. Furthermore, peptide absorption occurred more quickly than in the case of high molecular weight proteins [Citation18,Citation19]. LFED soybean ingestion could induce increased blood insulin levels more quickly than untreated soybean in the present study. However, this possibility was not completely clarified as the blood insulin level and amino acid concentration were not measured in the present study. Therefore, further investigations would be required to describe these values.
In conclusion, we have demonstrated that orally ingested LFED soybean quickly stimulated muscle protein synthesis by activating the mTOR signaling pathway compared to orally ingested untreated soybean. This suggests that ingesting LFED soybean after exercise could be more nutritious than untreated soybean for muscle hypertrophy. Taken together, these results might help the development of a novel nutrition strategy in order to stimulate muscle synthesis and enhance effective muscle hypertrophy for athletes after resistance training.
Author’s contribution
Kohei Ono and Minoru Tanaka did the experiment, dissected mice, analyzed the results, and write and modified the present article. Takuya Ikeji, Ryosuke Nakanishi, and Takumi Hirabayashi did the experiment, dissected mice and analyzed the results. Airo Tategaki, Hiroyo Kondo, and Akihiko Ishihara helped how analysis we should do and modified the paper. Hidemi Fujino helped to define the policy of the experiment and modified the paper.
Acknowledgments
We appreciated the grant from Grants-in-Aid for Scientific Research (JSPS KA-KENHI) with grant number 16K21517, 18H03130.
Disclosure statement
No potential conflict of interest was reported by the authors.
References
- Norman K, Stobäus N, Gonzalez M, et al. Hand grip strength: outcome predictor and marker of nutritional status. Clin Nutr. 2011;30(2):135–142. .
- Bilgin Ü, Çetin E, Çolak M, et al. Is body fat ratio of lower extremities a predictor of race time in university triathletes? J Phys Educ Sport Sci. 2017;11(3):227–233.
- Gordon BS, Kelleher AR, Kimball SR. Regulation of muscle protein synthesis and the effects of catabolic states. Int J Biochem Cell Biol. 2013;45(10):2147–2157.
- Ogasawara R, Fujita S. The role of mTOR signalling in the regulation of skeletal muscle mass in a rodent model of resistance exercise. Sci Rep. 2016;6:31142.
- Fujita S, Dreyer HC, Drummond MJ, et al. Nutrient signalling in the regulation of human muscle protein synthesis. J Physiol. 2007;582(15):813–823.
- Rennie MJ, Edwards RHT, Halliday D, et al. Muscle protein synthesis measured by stable isotope techniques in man: the effects of feeding and fasting. Clin Sci. 1982;63(6):519–523.
- Esmarck B, Andersen J, Olsen S, et al. Timing of postexercise protein in- take is important for muscle hypertrophy with resistance training in elderly humans. J Physiol. 2001;535(15):301–311.
- Cuthbertson D, Smith K, Babraj J, et al. Anabolic signaling deficits underlie amino acid resistance of wasting, aging muscle. Faseb J. 2005;19(4):422–424.
- Tipton K, Ferrando A, Phillips S, et al. Postexercise net protein synthesis in human muscle from orally administered amino acids. Am J Physiol. 1999;276(4):E628–E634.
- Roux PP, Shahbazian D, Vu H, et al. RAS/ERK signaling promotes site-specific ribosomal protein S6 phosphorylation via RSK and stimulates cap-dependent translation. J Biol Chem. 2013;282(19):14056–14064.
- Shimobayashi M, Hall M. Multiple amino acid sensing inputs to mTORC1. Cell. 2016;26(1):7–20.
- Goberdhan DCI, Wilson C, Harris AL. Amino acid sensing by mTORC1: intracellular transporters mark the spot. Cell Metab. 2016;23(12):580–589.
- Laplante M, Sabatini D. mTOR signaling in growth control and disease. Cell. 2012;149(13):274–293.
- Loewith R, Hall M. Target of rapamycin (TOR) in nutrient signaling and growth control. Genetics. 2011;189(4):1177–1201.
- Istfan N, Murray E, Janghorbani M, et al. The nutritional value of a soy protein concentrate (STAPRO-3200) for long-term protein nutritional maintenance in young men. J Nutr. 1983;113(12):2524–2534.
- Tang J, Moore D, Kujbida G, et al. Ingestion of whey hydrolysate, casein, or soy protein isolate: effects on mixed muscle protein synthesis at rest and follow- ing resistance exercise in young men. J Appl Physiol. 2009;107(3):987–992.
- Saneyasu T, Shindo H, Honda K, et al. The extract of soybean protein increases slow-myosin heavy chain expression in C2C12 myotubes. J Nutr Sci Vitaminol (Tokyo). 2018;64(4):296–300.
- Grimble G, Keohane P, Higgins B, et al. Effect of peptide chain length on amino acid and nitrogen absorption from two lactalbumin hydrolysates in the normal human jejunum. Clin Sci (Lond). 1986;71(1):65–69.
- Adibi S. The oligopeptide trans- porter (Pept-1) in human intestine: biology and function. Gastroenterology. 1997;113(1):332–340.
- Boirie Y, Dangin M, Gachon P, et al. Slow and fast dietary proteins differently modulate postprandial protein accretion. Proc Natl Acad Sci USA. 1997;94(26):14930–14935.
- Dangin M, Boirie Y, Garcia-Rodenas C, et al. The digestion rate of protein is an independent regulating factor of postprandial protein retention. Am J Physiol Endocrinol Metab. 2001;280(2):340–348.
- Goodman CA, Mabrey DM, Frey JW, et al. Novel insights into the regulation of skeletal muscle protein synthesis as revealed by a new nonradioactive in vivo technique. Faseb J. 2011;25(3):1028–1039.
- Hulmi J, Tannerstedt J, Selänne H, et al. Resistance exercise with whey protein ingestion affects mTOR signaling pathway and myostatin in men. J Appl Physiol. 2009;106(5):1720–1729.
- Ogasawara R, Sato K, Matsutani K, et al. The order of concurrent endurance and resistance exercise modifies mTOR signaling and protein synthesis in rat skeletal muscle. Am J Physiol Endocrinol Metab. 2014;306(10):E1155–E1162.
- Bradford MM. A rapid and sensitive method for the quantitation of microgram quantities of protein utilizing the principle of protein-dye binding. Anal Biochem. 1976;72(7):248–254.
- Anthony J, Yoshizawa F, Anthony T, et al. Leucine stimulates translation initia- tion in skeletal muscle of post- absorptive rats via a rapamycin- sensitive pathway. J Nutr. 2000;130(10):2413–2419.
- Dalle Pezze P, Ruf S, Sonntag AG, et al. A systems study reveals concurrent activation of AMPK and mTOR by amino acids. Nat Commun. 2016;21(7):13254.
- Anthony JC, Lang CH, Crozier SJ, et al. Contribution of insulin to the translational control of protein synthesis in skeletal muscle by leucine. Am J Physiol Endocrinol Metab. 2002;282(5):E1092–E1101.
- Biolo G, Tipton K, Klein S, et al. abundant supply of amino acids enhances the metabolic effect of exercise on muscle protein. Am J Physiol. 1997;273(1 Pt 1):E122–E129.
- Maebuchi M, Samoto M, Kohno M, et al. Improvement in the intestinal absorption of soy protein by enzymatic digestion to oligo- peptide in healthy adult men. Food Sci Technol Res. 2007;13(1):45–53.
- Rennie MJ, Wackerhage H, Spangenburg EE, et al. Control of the size of the human muscle mass. Annu Rev Physiol. 2004;66:799–828.
- Phillips SM, Tipton KD, Aarsland A, et al. Mixed muscle protein synthesis and breakdown after resistance exercise in humans. Am J Physiol. 1997;273(1 Pt 1):E99–E107.
- Dreyer HC, Drummond MJ, Pennings B, et al. Leucine-enriched essential amino acid and carbohydrate ingestion following resistance exercise enhances mTOR signaling and protein synthesis in human muscle. Am J Physiol Endocrinol Metab. 2008;294(2):E392–E400.
- Smeuninx B, Nishimura Y, McKendry J, et al. The effect of acute oral phosphatidic acid ingestion on myofibrillar protein synthesis and intracellular signaling in older males. Clin Nutr. 2019;38(3):1423–1432.
- Ogasawara R, Suginohara T. Rapamycin-insensitive mechanistic target of rapamycin regulates basal and resistance exercise-induced muscle protein synthesis. Faseb J. 2018;14:fj201701422R.
- Krezowski PA, Nuttall FQ, Gannon MC, et al. The effect of protein ingestion on the metabolic response to oral glucose in normal individuals. Am J Clin Nutr. 1986;44(6):847–856.
- Coleman ME, DeMayo F, Yin KC, et al. Myogenic vector expression of insulin-like growth factor I stimulates muscle cell differentiation and myofiber hypertrophy in transgenic mice. J Biol Chem. 1995;270(20):12109–12116.