ABSTRACT
In a randomized double-blind crossover study, a canned beverage was prepared using an emulsion dispersion formulation (EM) of β-carotene and a crystal dispersion formulation (CR) of β-carotene; the beverages were ingested by human subjects daily for 2 weeks to compare the β-carotene bioavailability. EM-β-carotene enhanced the β-carotene concentrations in human plasma approximately 4-fold, but CR-β-carotene showed no statistically significant enhancement. Bioaccessibility is the ratio of the solubilized fraction to the whole amount ingested. Bioaccessibility of β-carotene from EM-β-carotene was higher than that from CR-β-carotene in an in vitro digestion test. Contrarily, β-carotene from CR-β-carotene, consists of all-trans-β-carotene, was higher than that from EM-β-carotene, consists of a mixture of cis and all-trans-β-carotene, on the uptake by intestinal Caco-2 cells, suggesting that bioaccessibility was a critical factor in β-carotene bioavailability in this study. EM-β-carotene thus has potential as a food coloring agent with value added because it enhances β-carotene bioavailability.
Graphical abstract
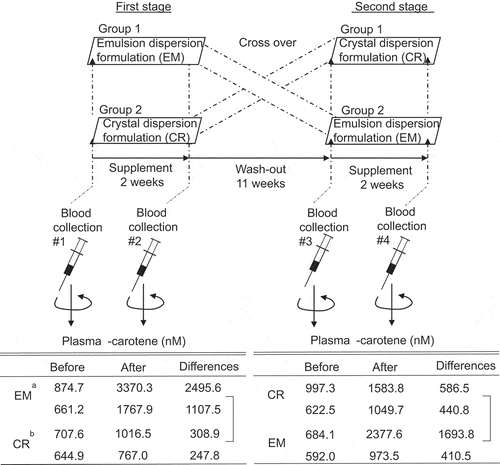
Experimental design of the β-carotene formulations trial, and differences in β-carotene concentration in human plasma before and after intake of β-carotene beverages.
β-Carotene is a main carotenoid found in fruits and vegetables (). β-Carotene not only has a nutrient function, acting as provitamin A, but also functions to prevent disease, via protection from sun damage [Citation1], anti-obesity activity [Citation2], and anti-inflammatory action [Citation3]. In addition, it has been widely used in the food industry as a pigment, because β-carotene and its cleavage products [Citation4] add color and flavor to foods and dishes. Carotenoids in use as edible food coloring agents need to be formulated to satisfy requirements for both uniformity and resistance to oxidation. Fat-soluble carotenoids do not dissolve in water, so they must be capable of uniform dispersion in water in order to use them effectively in foods. As examples, an aqueous dispersion, emulsion [Citation5–7], beadlets [Citation8], and a cyclodextrin inclusion complex [Citation9] of carotenoids have been employed. Carotenoids also display high sensitivity to light and oxygen due to numerous double bonds in the molecules. Antioxidants such as tocopherol, tert-butyl-hydroquinone, ascorbic acid, ethylenediamine tetraacetic acid, and coenzyme Q10 have been reported to inhibit the degradation of β-carotene in an aqueous dispersion system [Citation10–12].
Carotenoids can provide a uniform and stable color in foods using these emulsification and antioxidant technologies, but this only assures their roles as food coloring agents. Unless carotenoids are absorbed into the body, they cannot be expected to have their other described functions in human health. In the digestive tract, carotenoids are released from the food matrix and then dissolved in the fats and oils eaten as part of a meal. After an emulsion is formed with bile, carotenoids therein are solubilized in mixed bile salt micelles by the action of pancreatic juice lipase. Only the mixed bile salt micelles are accessible to intestinal cells, and then carotenoids can be taken up by the cells [Citation13]. In general, the fat-soluble carotenoids have low bioavailability [Citation13]. Thus, if carotenoids as food coloring agents could be provided with high bioavailability, they would have high nutritional functionality. Such food coloring agents will provide high added value. Among the dispersion formulations of β-carotene for food coloring, an emulsion dispersion formulation and a crystal dispersion formulation are now commercially available. Since β-carotene is solubilized in mixed bile salt micelles before being absorbed in the intestinal tract, it appears that β-carotene in an emulsified formulation should be easily solubilized and consequently easily absorbed. On the other hand, in the form of a crystal dispersion, β-carotene may not be easily absorbed in the intestinal tract. However, when small β-carotene crystals are taken directly into the intestinal tract, fine crystallization may increase β-carotene absorption.
For the present study, human subjects took part in a randomized double-blind crossover trial of two types of manufactured canned beverages, where each contained a different formulation: either an emulsified dispersion or a crystalline dispersion of β-carotene. The results would determine which method of dispersion offers more bioavailability of β-carotene.
In addition to the human subjects segment, bioaccessibility, which is the ratio of the solubilized fraction to the whole amount ingested, and cellular uptake of β-carotene were evaluated using in vitro digestion or Caco-2 cells, respectively, to investigate the mechanism underlying the bioavailability of β-carotene in the two formulations.
Materials and methods
Materials
An emulsion dispersion formulation (Control No. EM-BC-1, EM-β-carotene) and a crystal dispersion formulation (Control No. CR-BC-5, CR-β-carotene) were obtained from T. Hasegawa Co., Ltd. (Tokyo, Japan). Pyrogallol and retinol were purchased from FUJIFILM Wako Pure Chemical Corporation (Osaka, Japan). Retinyl acetate, pancreatin (porcine), bile extract porcine, β-carotene (type II), 1-oleoyl-rac-glycerol (monoolein), and fetal bovine serum (FBS) were purchased from Sigma-Aldrich (St. Louis, MO, USA). β-Carotene was purified by alumina column chromatography (neutral, grade III; MP Biomedicals, Solon, OH, USA). Ethyl β-apo-8ʹ-carotenoate was purchased from Fluka Biochemica (Buchs, Switzerland). α-Carotene, lutein, and lycopene were prepared as previously described [Citation14,Citation15]. Dulbecco’s modified Eagle’s medium (DMEM) was purchased from Nissui Pharmaceutical (Tokyo, Japan). The other chemicals and solvents used were of reagent grade.
Manufacture of two canned beverages containing the β-carotene formulation for human subjects
A canned beverage was prepared so as to contain 10 mg of β-carotene in a volume of 190 mL. At least 112 cans of the same beverage per formulation type were made by T. Hasegawa Co., Ltd. The can was opaque and not labeled, to keep subjects from identifying the beverages from the outside. The composition of the canned beverages used in human subjects is shown in . The beverages were stored at 4°C during the test period. At 3 months after manufacture, both beverages still had approximately 90% of the original β-carotene in them.
Table 1. Material composition of the canned beverages
Human subjects
This trial was conducted in accordance with the ethical guidelines for medical research on humans in the Declaration of Helsinki. The following experimental methods were approved by the Ethics Committees (Food Research Institute, 29NFRI-0011). Volunteers were told the method, purpose, potential risks, and duration of the study, and then they provided informed consent.
Eight healthy adults (four men and four nonpregnant women, 24–42 years old) were recruited as volunteers. Smokers were not included as subjects because of concern about increasing their lung cancer risk via intake of β-carotene at a high concentration [Citation16]. The volunteers did not take supplements of carotenoids, including β-carotene/vitamin A, in their daily lives. Subjects were randomly divided into two groups with matching gender ratio. The experimental protocol is shown in . In a double-blind crossover trial of EM-β-carotene versus CR-β-carotene, subjects drank a canned beverage after every lunch for 2 weeks. The washout period was approximately 11 weeks. In previous studies, 2 weeks was reported to be sufficient to significantly change and depletion in plasma β-carotene concentrations [Citation17,Citation18]. During the trial period, the subjects continued their normal daily lives. There were no side effects and no dropouts during the trial period.
Blood sampling was performed on the day before the start of and at the day after the end of β-carotene intake. The blood (10 mL) was put into a heparin vacuum tube, centrifuged at 1,400 g for 10 min, and a plasma fraction was collected. It was immediately stored at – 80°C until analysis.
Analysis of carotenoids and retinoids in human plasma
Carotenoids and retinoids from the human plasma were extracted using a previous method, modified slightly [Citation19]. The plasma (0.4 mL) was mixed with 0.4 mL phosphate-buffered saline (PBS), 0.8 mL ethanol containing both retinyl acetate (1 μmol) as an internal standard and α-tocopherol (400 pmol) as an antioxidant, 0.8 mL ethyl acetate, and 0.8 mL hexane, and then was mixed vigorously with a Vortex mixer for each addition. After centrifugation (410 g for 5 min at 4°C), the supernatant was collected. Ethyl acetate and hexane were further added in the same volumes to the lower layer and mixed in the same manner. After centrifugation, the recovered supernatant was combined with the first one. The combined supernatant was dried with a centrifugal evaporator. The residue was dissolved in 400 μL of dichloromethane/methanol (1:4, v/v), and an aliquot (10 μL) was subjected to high-performance liquid chromatography (HPLC) under the following conditions: system 1 for carotenoids and retinoids and system 3 for geometric isomers of β-carotene.
The recoveries of the β-carotene and retinol spiked into human plasma were 98% and 105%, respectively. The lower limits of quantification of β-carotene and retinol were calculated from a signal-to-noise ratio of 10 in the chromatogram and were estimated as 13 and 41 nmol/L plasma, respectively.
In vitro digestion of the β-carotene formulations
Gastrointestinal digestion was simulated by modifying a method we previously reported [Citation20]. The solution for the digestion step was prepared by diluting each of the two formulations, EM-β-carotene and CR-β-carotene, with water containing 0.55% (w/v) pyrogallol as an antioxidant. The β-carotene concentration in the β-carotene solutions was 0.55% (w/v), which was equal to 100 μM (almost the same as the concentration of β-carotene in the canned beverages). The blank solution, without the β-carotene formulation, was also prepared in the same manner, to use in subsequent intestinal absorption tests described in the following. Pepsin was dissolved in a buffer composed of 3.6 mmol/L of CaCl2, 1.5 mmol/L of MgCl2, 49 mmol/L of NaCl, 12 mmol/L of KCl, with 6.4 mmol/L of KH2PO4 to 0.5% (w/v). The formulation solution or the blank solution, 1.9 mL, was mixed with 1 mL of the pepsin solution in a plastic tube with a screw cap. The pH was adjusted to 2.0 with HCl, and then the mixture was shaken at 120 strokes/min in the dark at 37°C for 1 h under a nitrogen atmosphere. After incubation, the pH of the mixture was raised to 5.0 with NaHCO3, and then 1 mL of a solution composed of pancreatin (4 g/L) and bile extract (25 g/L) dissolved in 0.1 mol/L of NaHCO3 was added to the mixture. After the pH had been further raised to 7.5 with NaOH, the mixture was shaken again at 120 strokes/min in the dark at 37°C for 2 h under a nitrogen atmosphere. After incubation, the digesta was centrifuged at 2,900 g for 20 min at 4°C, and then part of the supernatant was passed through a 0.2-μm filter to obtain a solubilized fraction of β-carotene. The filtrate was the resulting clear solution, and then β-carotene molecules would be incorporated into the mixed bile salt micelles, the structure of which has been previously reported as a disc-shaped micelle model [Citation21]. As in our previous report [Citation20], we have regarded the filtrate as the solubilized fraction. Bioaccessibility (%) was defined as the ratio of the amount of β-carotene in the filtrate (solubilized fraction) to that in the original β-carotene formulation. The filtrate was diluted with 30 volumes of dichloromethane:methanol (1:4, v/v). An aliquot (20 μL) was subjected to HPLC under the system 2 condition.
Cell culture and differentiation
Caco-2 cells, which have been widely used as a model of human intestinal epithelial cells, were purchased from the American Type Culture Collection (Rockville, MD, USA). The cells were cultured in a medium consisting of DMEM, 10% heat-inactivated FBS, 4 mM L-glutamine, 40 units/mL of penicillin, 40 μg/mL of streptomycin, and 0.1 mM nonessential amino acids at 37°C in a humidified atmosphere of 5% CO2 in air, as described and used previously [Citation14]. The differentiated cells served as an intestinal model for evaluating the cellular uptake of β-carotene. To obtain differentiated Caco-2 cell monolayers, the cells were seeded into 12-well plates at a density of 10 × 104 cells per well containing 1 mL of the culture medium, and the medium was replaced with fresh medium twice a week for 21 d. We have previously reported that sufficient cell-to-cell adhesion in the Caco-2 cells was formed under the same culture conditions [Citation14,Citation22].
Evaluation of β-carotene uptake by differentiated Caco-2 cells from digesta obtained in an in vitro digestion test
An intestinal absorption test was selected to evaluate uptake of β-carotene from the solubilized fraction (mixed bile salt micelles) in digesta prepared through in vitro digestion of the β-carotene formulations with the differentiated Caco-2 cells. However, if the solubilized fraction was to be directly applied to the cells, the cells would be lysed. In addition, the β-carotene concentration of the solubilized fraction will vary between digesta of formulations. Therefore, before addition of a solubilized fraction to the cells, the solubilized fraction was diluted and the β-carotene concentration was adjusted as follows: The concentration of β-carotene in the solubilized fraction from digesta of each formulation was adjusted, by adding blank solution, to 20 μM, which was near the lowest concentration among the results obtained from in vitro digestion. This adjusted solution was further diluted 10-fold with the medium used for the cell culture, but without FBS, to give an initial β-carotene concentration (2 μM). To confirm the initial concentration of micellar β-carotene in the medium before adding this to the Caco-2 cells, one part of the medium was diluted with nine parts of dichloromethane/methanol (1:4, v/v), and HPLC analysis under the system 2 condition was performed. The initial concentration was confirmed to be 1.93 ± 0.03 μM.
After washing the differentiated Caco-2 cells in a 12-well plate twice with 1.0 mL of FBS-free culture medium, 1.0 mL/well of the medium containing the micellar β-carotene was added. After 2 h of incubation in a CO2 incubator at 37°C, the medium was collected to evaluate the residual amount of micellar β-carotene as described next. The cells were washed with 1.0 mL of 10 mM taurocholate in PBS and then were washed twice with 1.0 mL of PBS. They were homogenized in 2.0 mL of PBS containing 0.01 mL of 0.2 mM α-tocopherol/methanol as an antioxidant, with a probe-type sonicator (Ultra S, VP-5S; Taitec, Saitama, Japan). An aliquot (1.8 mL) of the cell homogenate was mixed with 0.2 mL of 0.4 mM ethyl β-apo-8ʹ-carotenoate/ethanol as an internal standard. Subsequently, 1.6 mL ethanol, 1.8 mL ethyl acetate, and 1.8 mL hexane were added, and after each addition the solution was agitated with a Vortex mixer. The upper phase was dried in a centrifugal evaporator. The residue was then dissolved in 0.2 mL of dichloromethane/methanol (1:4, v/v), and an aliquot (20 μL) was subjected to HPLC under the system 2 condition.
Another aliquot of the cell homogenate was used to determine the protein content using the Lowry method [Citation23]. To measure the residual micellar β-carotene in the medium at the end of the 2-h incubation, aliquots of the medium were analyzed after passing through a filter and the initial β-carotene concentration was checked because the amount of β-carotene in the micelles during incubation reflects the amount accessible to the cells [Citation24].
Analysis of the geometric isomeric composition of β-carotene in formulations
To confirm the geometric isomer composition of β-carotene in EM-β-carotene and CR-β-carotene, β-carotene was extracted from each formulation and subjected to HPLC analysis as follows: Water (720 μL) was added to 80 μL of the formulation solution containing 100 μM β-carotene prepared at the section of the in vitro digestion test. Subsequently, 0.8 mL each of ethanol, ethyl acetate, and hexane was added, as well as the extraction of β-carotene uptake from Caco-2 cells. The solvents were dried in the centrifugal evaporator. The residue was then dissolved in 0.4 mL of dichloromethane/methanol (1:4, v/v), and an aliquot (20 μL) was subjected to HPLC under the system 3 conditions.
The geometric isomer compositions of β-carotene in each formulation were 15-cis/13-cis/all-trans/9-cis (23.89: 7.15: 44.58: 24.37) for EM-β-carotene and 15-cis/all-trans (1.74: 98.26) for CR-β-carotene, respectively. The identification of these peaks was also performed, as described in the following.
Evaluation of β-carotene uptake by differentiated Caco-2 cells from synthetic mixed micelles
As already described, the geometric isomer composition of β-carotene was very different for the two β-carotene formulations. To compare the uptake of mixed-isomer β-carotene (for EM-β-carotene) versus all-trans-β-carotene (for CR-β-carotene), an intestinal absorption test was performed in which synthetic mixed micelles were prepared instead of the diluted solubilized fraction (mixed bile salt micelles) of the digesta.
All-trans-β-carotene is readily available, but its cis isomers are difficult to obtain. Therefore, β-carotene consisting of cis isomer as the main constituent isomer was obtained by extracting and purifying from EM-β-carotene. After adding an equal amount of water to EM-β-carotene (0.5 mL), β-carotene was extracted with the addition of 1.0 mL each of ethanol, ethyl acetate, and hexane, as described earlier. After drying the solvent, the residue was dissolved in hexane and subjected to silica column chromatography. The red band fraction was collected, dried, redissolved in hexane, and subjected to alumina column (III) chromatography [Citation15]. The concentration of β-carotene was determined, as the all-trans form, using the molecular extinction coefficient (E1%, 1 cm, 2592 at 450 nm in hexane).
Synthetic mixed micelles were prepared as described previously [Citation14]. The synthetic mixed micelle components were dissolved in an appropriate solvent and transferred to glass test tubes. The solvent was evaporated under a stream of argon gas, and further drying took place in a centrifugal evaporator. The dry matter was dispersed in the FBS-free culture medium with vortexing, and the resulting clear solution was passed through a 0.2-μm filter to remove any β-carotene that was not solubilized into the micelles. The synthetic mixed micelles consisted of taurocholate (2 mM), oleic acid (33 μM), monoolein (100 μM), and β-carotene (1 μM). The initial concentration of β-carotene was confirmed to be 0.954 ± 0.002 μM.
Cell culture, incubation with β-carotene, and HPLC analysis of the concentration of micellar β-carotene in the medium before and after incubation with Caco-2 cells and of β-carotene uptake by the cells were performed, as described in the section on evaluation of β-carotene uptake by differentiated Caco-2 cells from digesta.
HPLC analyses
Carotenoids and retinoids in human plasma were analyzed by HPLC (using an LC-20AT pump, an SPD-M10A photodiode array detector, and a CTO-10AS column oven at a constant temperature of 25°C; Shimadzu, Kyoto, Japan) on an ODS-80Ts column (2.0 × 250 mm; Tosoh, Tokyo, Japan) with an ODS-S1 precolumn (2.0 × 10 mm; Tosoh). Solvent A was acetonitrile/methanol/water (75:15:10, v/v/v) containing 0.1% ammonium acetate, and solvent B was methanol/ethyl acetate (70:30, v/v) containing 0.1% ammonium acetate. A linear gradient from solvent A (100%) to solvent B (100%) was applied for 10 min at a flow rate of 0.2 mL/min. Solvent B was on hold from 10 to 32 min. Carotenoids and retinoids were quantified from the peak area at 450 nm and 325 nm, respectively, using a calibration curve of the authentic standard (system 1).
The β-carotene in vitro digestion test and in two cell culture tests were analyzed using the same HPLC system, but the isocratic analysis was conducted using an ODS-80Ts column (2.0 × 150 mm) with solvent B (system 2).
The geometric isomers of β-carotene were analyzed by the same HPLC system, but an isocratic analysis was performed with solvent C, which was methanol/ethyl acetate (60:40, v/v) containing 0.1% ammonium acetate, using a Wakopak Navi C30-5 column (2.0 × 250 mm; FUJIFILM Wako Pure Chemical Corporation) with a Wakopak Navi C30-5 column (2.0 × 30 mm) as a precolumn (system 3).
The isomers were identified from the peak of the authentic standards (for all-trans) at 450 nm and with the literature (for 15-cis, 13-cis, and 9-cis) [Citation25], and from spectrum analysis of each peak on the photodiode array detector (data not shown). The ratio of isomers was indicated by the area ratio. Other than the isomer analysis, the ODS column was used for quantitative analysis of β-carotene as an all-trans form, because the ODS column does not separate the geometric isomers of carotenoid under these conditions.
Average particle size of formulation samples
The β-carotene formulation was diluted, as well as the in vitro digestion test section, with water, but without pyrogallol in this part of the analysis.
The average particle sizes of the diluted solutions were measured using a Zetasizer Nano ZS (633-nm laser, Malvern Panalytical ltd., Malvern, UK). EM-β-carotene and CR-β-carotene particles in solution had a diameter of 168.1 nm and 157.3 nm, respectively.
All the experiments using carotenoids and retinoids except for the measurement of particle size were conducted under dim yellow light to minimize isomerization and degradation of carotenoids under light irradiation.
Statistical analysis
The data were analyzed by one-way analysis of variance (ANOVA), followed by a t-test. Values of P < 0.05 were considered to indicate significance.
Results
Human subjects
shows chromatograms of typical carotenoids (a) and retinoids (b) in human plasma. The main carotenoid peaks were lutein, lycopene, α-carotene, and β-carotene. Two peaks at approximately 20 min and 22 min were not identified. Retinol appeared as a large peak, but no retinol palmitate was detected.
Figure 3. Typical chromatograms of human plasma extracts. Carotenoids (a) and retinoids (b) were analyzed by HPLC with a C18 column. Peak a, lutein; peak b, lycopene; peak c, α-carotene; peak d, β-carotene; peak e, retinol; peak f, retinyl acetate as an internal standard

shows the concentrations of four carotenoids and retinol. These chemical structures are shown in , as found in the plasma before and after ingesting the β-carotene canned beverage for 2 weeks. Only the β-carotene in the EM-β-carotene intake group had a significantly higher concentration after intake than before, and the increased concentrations were 3.5 times for the first stage and 3.9 times for the second stage, respectively. For the CR-β-carotene intake group, β-carotene concentrations after intake showed a trend to increase, by 1.4 times for the first stage and 1.6 times for the second stage, respectively. However, there was no statistical difference between these concentrations before and after intake. In both groups, the concentrations of lutein, α-carotene, lycopene, and retinol were almost the same before and after intake (no statistically significant difference).
Table 2. Retinol and carotenoids in human plasma before and after intake of the beverages prepared from two β-carotene formulations, EM-β-carotene and CR-β-carotene
compares the formulations in terms of the differences in the concentrations of β-carotene and retinol in plasma before and after intake of the canned beverages. In both the first and the second stages, the differences in EM-β-carotene were significantly higher than for CR-β-carotene. On the other hand, there was no difference in the retinol concentration between the two β-carotene formulations.
Table 3. Differences in retinol and β-carotene in human plasma before and after intake of β-carotene beverages
shows a typical chromatogram of β-carotene geometric isomers contained in the β-carotene formulations. As already described, β-carotenes were made up of almost entirely the all-trans form in CR-β-carotene, while more than half were cis forms in EM-β-carotene. However, as shown in , only the all-trans isomer was observed in human plasma, regardless of whether the intake was of EM-β-carotene (a) or of CR-β-carotene (b).
In vitro digestion test
The bioaccessibility of β-carotene in the β-carotene formulations during the digestion process in the stomach and small intestine was evaluated by in vitro simulation (). The bioaccessibility from EM-β-carotene was significantly higher than that from CR-β-carotene.
Figure 6. The bioaccessibility of β-carotene in the formulations. EM-β-carotene and CR-β-carotene aqueous solutions were applied to an in vitro digestion test. Data are the means ± SD (n = 4) in a single experiment. Replicate experiments showed a similar trend. The asterisk indicates a value with statistically significant difference by the unpaired t-test (p < 0.05)

β-Carotene uptake by differentiated Caco-2 cells
Intestinal absorption tests were evaluated following the in vitro digestion tests. The diluted solubilized fraction (mixed bile salt micelles) of digesta obtained by in vitro digestion was applied to intestinal model Caco-2 cells. As shown in ), the amounts of β-carotene taken up by Caco-2 cells were higher with CR-β-carotene than with EM-β-carotene.
Figure 7. The uptake of β-carotene by Caco-2 cells. Caco-2 cells were treated with solubilized β-carotene for 2 h. The cells and β-carotene for the treatments were prepared as described in the Materials and Methods section. (a) β-Carotene solubilized fraction (mixed bile salt micelles) from formulations in the in vitro digestion test. (b) β-Carotene solubilized in the synthetic mixed micelles. Data are the mean ± SD values of four wells in a single experiment. Replicate experiments showed a similar trend. The asterisk indicates a value with statistically significant difference by the unpaired t-test (p < 0.05)

As described in the preceding, the geometric isomeric composition of β-carotene was greatly different for EM-β-carotene versus CR-β-carotene. Therefore, using a cis/trans mixture form of β-carotene (cis:trans = 55.4:44.6) and an all-trans form of β-carotene, respective synthetic mixed micelles were prepared instead of diluted solubilized fractions (mixed bile salt micelles) of digesta. The β-carotene uptake was examined after supplying these synthetic mixed micelles to Caco-2 cells. The amount of β-carotene uptake was significantly higher for synthetic mixed micelles with the all-trans form for CR-β-carotene than with the cis/trans mixture form for EM-β-carotene ()).
There is some concern that the decrease in micellar carotenoids might have reduced the transfer of carotenoids from micelles into the cells because only micellar carotenoids can be taken up by Caco-2 cells. The reduced concentration of the micellar carotenoid could be caused by an increase in insoluble carotenoids due to the cells’ uptake of other micellar components such as fatty acid and monoolein during the incubation with cells [Citation26]. Thus, the residual amounts of micellar β-carotene were analyzed after incubation for 2 h. As shown in , the order of the amount of residual micellar β-carotene was EM-β-carotene > CR-β-carotene and cis/trans mixture form for EM-β-carotene > all-trans form for CR-β-carotene in the experiments using either a diluted solubilized fraction (mixed bile salt micelles) of digesta or synthetic mixed micelles, respectively.
Table 4. Residual amounts of micellar β-carotene in medium after incubation with Caco-2 cells
Discussion
β-Carotene, which is a fat-soluble pigment with a nutritive function as provitamin A, is widely used as a food coloring agent. The present study used a human subjects in a randomized double-blind crossover trial of drinking canned beverages prepared with two types of dispersion formulations (emulsion dispersion formulation, EM, and crystal dispersion formulation, CR) containing β-carotene for use as a food coloring agent, and then compared the bioavailability of β-carotene between the two formulations (). The daily intake of the canned beverage containing EM-β-carotene significantly enhanced the concentration of β-carotene in plasma compared to that of CR-β-carotene ( and ). Initially, intake of the CR-β-carotene beverage did not statistically significantly enhance the β-carotene plasma concentration. A similar result has been reported recently in rat experiments. Oral administration of crystal β-carotene did not increase its plasma concentration at all, whereas administration of noncrystalline β-carotene significantly increased the plasma concentration [Citation27]. In addition, it has also been pointed out that the formulation administered to humans may be less absorbed in crystal than in powder form when using lutein bioavailability studies [Citation28,Citation29]. These findings indicate that intake of carotenoids in the form of crystals is unlikely to enhance its bioavailability. At least, β-carotene will not be directly taken up as crystals in intestinal cells. The form of the formulations, such as crystal, powder, and emulsion, becomes important in terms of the amount of bioavailability of the carotenoids. In other words, EM-β-carotene offers more potential as a high value-added food coloring agent with nutritional and functional properties.
Daily intake of an EM-β-carotene beverage containing 10 mg β-carotene for 2 weeks enhanced the plasma β-carotene concentration by approximately 3.5–3.9 times (). There have been some previous reports of random crossover human trials on β-carotene intake in forms that did not involve any food matrix, such as supplements and vegetable juices. For example, β-carotene plasma concentration before and after intake showed enhancement of 4.4-fold from daily intake of a supplement containing 31.4 mg β-carotene for 3 months [Citation30], 4- to 6-fold by daily intake of a supplement containing 30 mg β-carotene for 6 weeks [Citation31], approximately 2-fold by daily intake of fish oil together with a supplement containing 6 mg β-carotene for 3 weeks [Citation32], and 4.4-fold (2.6 times the baseline) from daily intake of carrot juice containing 27.1 mg β-carotene for 2 weeks [Citation18]. Although the experimental conditions differed from those of the present study, the effect of EM-β-carotene in the present study appears comparable to the results in these other studies in terms of enhancing plasma β-carotene concentrations, if the period and amounts of intake are taken into account.
The geometric isomer composition of β-carotene for EM-β-carotene and that for CR-β-carotene were very different. Specifically, β-carotenes with greatly different compositions were ingested by the two groups in the present study. The EM-β-carotene beverage group ingested a significant amount of cis-β-carotene, accounting for about 60% of the total β-carotene. However, only the peak of all-trans-β-carotene was observed in the geometric isomer analysis of β-carotene in human plasma after intake (). Consistent with these results, previous reports have shown that β-carotene in human serum was almost entirely in the all-trans form [Citation33,Citation34], whereas lycopene was predominantly in the cis form [Citation33]. In a comparison between two groups that took a synthetic all-trans-β-carotene or Dunaliella bardawil containing a 1:1 ratio of all-trans-β-carotene and 9-cis-β-carotene, the human plasma β-carotene concentration in the group with the synthetic all-trans-β-carotene was about 1.8 times higher than that in the other group [Citation35,Citation36]. Intake of Dunaliella salina consisting of 54% all-trans-β-carotene, 36% cis-β-carotene, and α-carotene increased the human serum levels of all-trans-β-carotene and α-carotene, but the amount of 9-cis-β-carotene concentration was below the lower detection limit [Citation37]. In a single dose test of 1 mg of C13-labeled 9-cis-β-carotene (purity 99.4%), all-trans-β-carotene was found in human plasma. The plasma concentration of 9-cis-β-carotene itself was low [Citation38]. Based on these reports, it seems that almost all of the cis-β-carotene failed to be absorbed in the intestinal tract, whereas a part of 9-cis-β-carotene may be converted to all-trans-β-carotene and then absorbed. Thus, β-carotene bioavailability was high for the EM-β-carotene group, even though the EM-β-carotene mainly contains the cis isomer, which was not easily absorbed, suggesting that the dispersed form is working.
As a case similar to cis-β-carotene, neoxanthin, violaxanthin, and fucoxanthin, which are usually ingested in vegetables and seaweeds, have been reported to be hardly detectable in human plasma [Citation19,Citation39], whereas fucoxanthin is absorbable in mice and rats [Citation40,Citation41]. The existence of a selective carotenoid absorption [Citation42] and excretion system in human has been proposed [Citation13]. The intestinal absorption of cis-β-carotene may also be regulated by such systems, for example, a selective facilitated diffusion by the scavenger receptor B type 1 (SR-B1) and an excretion by transporters such as ATP-Binding Cassette Transporter G5/G8, and further studies are required.
A provitamin A such as β-carotene after being absorbed is converted into retinal by the action of β-carotene-15,15ʹ-oxygenase (BCO1) and then is further metabolized to retinol or retinoic acid. In this study, β-carotene intake did not affect plasma retinol levels ( and ), consistent with previous reports [Citation18,Citation30,Citation32]. β-Carotene is known to be partially absorbed via SR-B1 in the intestinal tract [Citation43–45]. Since the expression of both SR-B1 and BCO1 is regulated by retinoic acid and the transcription factor ISX [Citation46], β-carotene will be not converted to more retinol than necessary. It was natural that lutein, lycopene, and α-carotene, which were the other carotenoids measured besides β-carotene, showed no change in plasma concentration before and after β-carotene intake () because these carotenoids were not contained in the beverages. This result may also reflect that subjects maintained a normal diet during the test.
This study elucidated the mechanism by which EM-β-carotene was significantly more effective while CR-β-carotene was less effective in enhancing β-carotene plasma concentrations observed after the beverage intake. The process of carotenoid absorption from food in the gastrointestinal tract was described earlier in this article [Citation13]. There are three key processes that affect the carotenoid bioavailability: release from the food matrix, solubilization to mixed bile salt micelles, and incorporation into intestinal cells. The β-carotene formulations used in the present study have no food matrix. Therefore, the processes that have contributed to β-carotene bioavailability in these human studies would be bioaccessibility and/or intestinal absorbability. This study conducted in vitro digestion for the bioaccessibility of β-carotene and examined the uptake of β-carotene by Caco-2 cells as a measure of intestinal absorption.
For the in vitro digestion test, the bioaccessibility of β-carotene in CR-β-carotene was lower than that of β-carotene in EM-β-carotene (). However, there remains some concern about whether an in vitro digestion test is applicable to evaluate the solubilization of the β-carotene formulations. In this method, for the upper phase of the digesta after centrifugation, the portion that can pass through a 0.2-μm filter was defined as the solubilized fraction, but 90% of these formulation samples after centrifugation was able to pass through the filter even before being used in the digestion test. The bioaccessibilities in the actual test results were 78% for EM-β-carotene and 62% for CR-β-carotene, respectively (). β-Carotene would have been incorporated into the mixed bile salt micelles from the formulation, and then the micelles with a particle size of less than 0.2 μm would have passed through the filter. However, the solubilized fraction of the digesta using CR-β-carotene might be contaminated with small-sized crystals. It is difficult to examine the existence of such crystals.
The difference between the bioaccessibility of β-carotene in two formulations will also be affected by their geometric isomer compositions. As described earlier in this article, EM-β-carotene contained a large amount of cis-form β-carotene, while CR-β-carotene consisted of nearly 100% all-trans-β-carotene (). In general, all-trans-β-carotene is easier to crystallize than the cis isomer. Crystals have strong bonds between molecules and show low solubility. Lycopene, a structural isomer of β-carotene, has an all-trans form with higher crystallinity and lower solubility than the cis form [Citation47]. For β-carotene it has also been reported that the bioaccessibility is higher in the cis form from the in vitro digestion test [Citation48]. Therefore, the bioaccessibility of β-carotene from CR-β-carotene will be lower.
This study examined the intestinal absorption of the solubilized fraction in the digesta by Caco-2 cells. Contrary to the trend of bioaccessibility, the uptake of β-carotene from the CR-β-carotene digesta was higher than that from the EM-β-carotene digesta ()). This was followed by an effort to elucidate the cause of this result in cellular uptake from the viewpoint of the geometric isomeric composition of β-carotene. Similar to the results described earlier, the amount of all-trans-β-carotene uptake for CR-β-carotene was higher than that of the cis/trans mixture of β-carotene for EM-β-carotene in the test using synthetic mixed micelles composed of these β-carotene isomers ()). This result suggested that the tendency to take up the β-carotene isomer in the synthetic mixed micelle reflected the process of β-carotene uptake from the digesta.
The geometric isomer composition of β-carotene taken up by Caco-2 cells has been reported to have a high proportion of all-trans isomers, compared with the isomer composition of β-carotene in the medium used for cellular uptake [Citation48]. That is, the all-trans isomer was more easily taken up by cells than the cis isomer. Therefore, the all-trans carotenoids are easily crystallized and have low solubility, but once they are solubilized in digestive juice, they can be absorbed easily by intestinal cells.
In the present study, the enhancing of plasma concentrations of β-carotene in human subjects showed that CR-β-carotene was less effective and EM-β-carotene was more effective, which appeared to reflect the process of solubilization rather than that of intestinal absorption. In other words, bioaccessibility was a critical factor in the bioavailability of β-carotene in the formulations.
There was uncertainty about the effect of the residual β-carotene micelle concentration in the medium after incubation in the β-carotene uptake by Caco-2 cells. However, the remaining concentration for the cases with larger uptake (mixed bile salt micelles from CR-β-carotene digesta and synthetic mixed micelles containing all-trans-β-carotene) was lower (), indicating that the residual micelle concentration did not affect cellular uptake much, consistent with our previous reports [Citation14,Citation22].
In conclusion, to estimate the β-carotene bioavailability of two types of formulation, namely, an emulsion dispersion formulation (EM) and a crystal dispersion formulation (CR), this study conducted a randomized double-blind crossover trial. After human subject intake of a canned beverage (containing 10 mg β-carotene) manufactured using these formulations daily for 2 weeks, the β-carotene concentration in plasma increased approximately 3.5–3.9 times in the EM-β-carotene group, and did not do so in the CR-β-carotene group. The process of digestion and absorption of β-carotene in the formulations was simulated using in vitro tests. An in vitro digestion test showed that the bioaccessibility of β-carotene in EM-β-carotene was higher than that of the β-carotene in CR-β-carotene. On the contrary, the cellular uptake of β-carotene by Caco-2 cells was higher in digesta from CR-β-carotene than in digesta from EM-β-carotene. The geometric isomer composition of β-carotene differed greatly between EM-β-carotene and CR-β-carotene. More than half of the β-carotene in EM-β-carotene consisted of the cis form, but that in CR-β-carotene consisted of almost entirely all-trans-β-carotene. Only the all-trans-β-carotene could be detected in the human plasma. The cis-β-carotene may not be absorbed by intestinal cells. The trans form of β-carotene is easily crystallized and hard to solubilize, but once it is solubilized, it is easily absorbed. On the other hand, the cis form of β-carotene is easily solubilized but intestinal cells hardly absorbed it. The present study indicates that bioaccessibility rather than intestinal absorbability was a critical factor for the bioavailability of β-carotene in the formulations. EM-β-carotene has potential for use as a food coloring agent with an added value of high β-carotene bioavailability.
Authors contributions
Eiichi Kotake-Nara designed, performed experiments, analyzed data, and, wrote the article. Megumi Hase performed the experiments of in vitro digestion experiments. Both authors contributed to the critical revision of the article.
Acknowledgments
We thank the volunteers for human subjects, the staff who prepared the canned beverages, the staff who prepared and analyzed the formulations, the hospital staff who collected the blood, and Dr. Isao Kobayashi for using Zetasizer Nano ZS on measuring of the average particle size. We also thank Enago (www.enago.jp) for the English language review.
Disclosure statement
No potential conflict of interest was reported by the authors.
References
- Stahl W, Sies H. β-Carotene and other carotenoids in protection from sunlight. Am J Clin Nutr. 2012;96:1179S–1184S.
- Lobo GP, Amengual J, Li HN, et al. Beta,beta-carotene decreases peroxisome proliferator receptor gamma activity and reduces lipid storage capacity of adipocytes in a beta,beta-carotene oxygenase 1-dependent manner. J Biol Chem. 2010;285:27891–29789.
- Imamura T, Bando N, Yamanishi R. Beta-carotene modulates the immunological function of RAW264, a murine macrophage cell line, by enhancing the level of intracellular glutathione. Biosci Biotechnol Biochem. 2006;70:2112–2120.
- Baldermann S, Fleischmann P, Bolten M, et al. Centrifugal precipitation chromatography, a powerful technique for the isolation of active enzymes from tea leaves (Camellia sinensis). J Chromatogr A. 2009;1216:4263–4267.
- Achir N, Hadjal T, Madani K, et al. Carotene reactivity in pink grapefruit juice elucidated from model systems and multiresponse modeling. J Agric Food Chem. 2015;63:3970–3979.
- Li R, Tan Y, Dai T, et al. Bioaccessibility and stability of β-carotene encapsulated in plant-based emulsions: impact of emulsifier type and tannic acid. Food Funct. 2019;10:7239–7252.
- Calligaris S, Manzocco L, Valoppi F, et al. Microemulsions as delivery systems of lemon oil and β-carotene into beverages: stability test under different light conditions. J Sci Food Agric. 2019;99:7016–7020.
- Gellenbeck K, Salter-Venzon D, Lala R, et al. A multicarotenoid beadlet for human nutrition - proof of concept of in vitro timed release. Acta Biochim Pol. 2012;59:35–38.
- Ivanova T, Mircheva K, Balashev K, et al. Monolayer kinetic model of formation of β-cyclodextrin-β-carotene inclusion complex. Colloids Surf B Biointerfaces. 2015;135:542–548.
- Liu Y, Hou Z, Yang J, et al. Effects of antioxidants on the stability of β-Carotene in O/W emulsions stabilized by Gum Arabic. J Food Sci Technol. 2015;52:3300–3311.
- Song HY, Moon TW, Choi SJ, et al. Impact of antioxidant on the stability of β-carotene in model beverage emulsions: role of emulsion interfacial membrane. Food Chem. 2019;279:194–201.
- Qian C, Decker EA, Xiao H, et al. Inhibition of β-carotene degradation in oil-in-water nanoemulsions: influence of oil-soluble and water-soluble antioxidants. Food Chem. 2012;135:1036–1043.
- Kotake-Nara E, Nagao A. Absorption and metabolism of xanthophylls. Mar Drugs. 2011;9:1024–1037.
- Kotake-Nara E, Yonekura L, Nagao A. Glyceroglycolipids affect uptake of carotenoids solubilized in mixed micelles by human intestinal Caco-2 cells. Lipids. 2015;50:847–860.
- Nara E, Hayashi H, Kotake M, et al. Acyclic carotenoids and their oxidation mixtures inhibit the growth of HL-60 human promyelocytic leukemia cells. Nutr Cancer. 2001;39:273–283.
- Tanvetyanon T, Bepler G. Beta-carotene in multivitamins and the possible risk of lung cancer among smokers versus former smokers a meta-analysis and evaluation of national brands. Cancer. 2008;113:150–157.
- Watzl B, Bub A, Brandstetter BR, et al. Modulation of human T-lymphocyte functions by the consumption of carotenoid-rich vegetables. Br J Nutr. 1999;82:383–389.
- Watzl B, Bub A, Briviba K, et al. Supplementation of a low-carotenoid diet with tomato or carrot juice modulates immune functions in healthy men. Ann Nutr Metab. 2003;47:255–261.
- Barua AB, Olson JA. Xanthophyll epoxides, unlike beta-carotene monoepoxides, are not detectibly absorbed by humans. J Nutr. 2001;131:3212–3215.
- Nagao A, Kotake-Nara E, Hase M. Effects of fats and oils on the bioaccessibility of carotenoids and vitamin E in vegetables. Biosci Biotechnol Biochem. 2013;77:1055–1060.
- Small DM, Penkett SA, Chapman D. Studies on simple and mixed bile salt micelles by nuclear magnetic resonance spectroscopy. Biochim Biophys Acta. 1969;176:178–189.
- Kotake-Nara E, Yonekura L, Nagao A. Lysoglyceroglycolipids improve the intestinal absorption of micellar fucoxanthin by Caco-2 Cells. J Oleo Sci. 2015;64:1207–1211.
- Lowry OH, Rosebrough NJ, Farr AL, et al. Protein measurement with the Folin phenol reagent. J Biol Chem. 1951;193:265–275.
- Yonekura L, Tsuzuki W, Nagao A. Acyl moieties modulate the effects of phospholipids on beta-carotene uptake by Caco-2 cells. Lipids. 2006;41:629–636.
- Marx M, Schieber A, Carle R. Quantitative determination of carotene geometricisomers in carrot juices and vitamin supplemented (ATBC) drinks. Food Chem. 2000;70:403–408.
- Kotake-Nara E, Yonekura L, Nagao A. Effect of glycerophospholipid class on the beta-carotene uptake by human intestinal Caco-2 cells. Biosci Biotechnol Biochem. 2010;74:209–211.
- Otani S, Miki S, Nakamura Y, et al. Improved bioavailability of β-carotene by amorphous solid dispersion technology in rats. J Nutr Sci Vitaminol. 2020;66:207–210.
- Chung HY, Rasmussen HM, Johnson EJ. Lutein bioavailability is higher from lutein-enriched eggs than from supplements and spinach in men. J Nutr. 2004;134:1887–1893.
- Bowen PE, Herbst-Espinosa SM, Hussain EA, et al. Esterification does not impair lutein bioavailability in humans. J Nutr. 2002;132:3668–3673.
- Wang W, Kucuk O, Franke AA, et al. Reproducibility of erythrocyte polyamine measurements and correlation with plasma micronutrients in an antioxidant vitamin intervention study. J Cell Biochem. 1996;62:19–26.
- Goodman MT, Hernandez B, Wilkens LR, et al. Effects of beta-carotene and alpha-tocopherol on bleomycin-induced chromosomal damage. Cancer Epidemiol Biomarkers Prev. 1998;7:113–117.
- Kiokias S, Gordon MH. Dietary supplementation with a natural carotenoid mixture decreases oxidative stress. Eur J Clin Nutr. 2003;57:1135–1140.
- Stahl W, Schwarz W, Sundquist AR, et al. Cis-trans isomers of lycopene and beta-carotene in human serum and tissues. Arch Biochem Biophys. 1992;294:173–177.
- Stahl W, Sundquist AR, Hanusch M, et al. Separation of beta-carotene and lycopene geometric isomers in biological samples. Clin Chem. 1993;39:810–814.
- Tamai H, Morinobu T, Murata T, et al. 9-cis beta-carotene in human plasma and blood cells after ingestion of beta-carotene. Lipids. 1995;30:493–498.
- Morinobu T, Tamai H, Murata T, et al. Changes in beta-carotene levels by long-term administration of natural beta-carotene derived from Dunaliella bardawil in humans. J Nutr Sci Vitaminol. 1994;40:421–430.
- Stahl W, Schwarz W, Sies H. Human serum concentrations of all-trans beta- and alpha-carotene but not 9-cis beta-carotene increase upon ingestion of a natural isomer mixture obtained from Dunaliella salina (Betatene). J Nutr. 1993;123:847–851.
- You CS, Parker RS, Goodman KJ, et al. Evidence of cis-trans isomerization of 9-cis-beta-carotene during absorption in humans. Am J Clin Nutr. 1996;64:177–183.
- Asai A, Yonekura L, Nagao A. Low bioavailability of dietary epoxyxanthophylls in humans. Br J Nutr. 2008;100:273–277.
- Yonekura L, Kobayashi M, Terasaki M, et al. Keto-carotenoids are the major metabolites of dietary lutein and fucoxanthin in mouse tissues. J Nutr. 2010;140:1824–1831.
- Sangeetha RK, Bhaskar N, Divakar S, et al. Bioavailability and metabolism of fucoxanthin in rats: structural characterization of metabolites by LC-MS (APCI). Mol Cell Biochem. 2010;333:299–310.
- During A, Hussain MM, Morel DW, et al. Carotenoid uptake and secretion by CaCo-2 cells: beta-carotene isomer selectivity and carotenoid interactions. J Lipid Res. 2002;43:1086–1095.
- During A, Dawson HD, Harrison EH. Carotenoid transport is decreased and expression of the lipid transporters SR-BI, NPC1L1, and ABCA1 is downregulated in Caco-2 cells treated with ezetimibe. J Nutr. 2005;135:2305–2312.
- van Bennekum A, Werder M, Thuahnai ST, et al. Class B scavenger receptor-mediated intestinal absorption of dietary beta-carotene and cholesterol. Biochemistry. 2005;44:4517–4525.
- Kotake-Nara E, Nagao A. Effects of mixed micellar lipids on carotenoid uptake by human intestinal Caco-2 cells. Biosci Biotechnol Biochem. 2012;76:875–882.
- Lobo GP, Hessel S, Eichinger A, et al. ISX is a retinoic acid-sensitive gatekeeper that controls intestinal beta,beta-carotene absorption and vitamin a production. Faseb J. 2010;24:1656–1666.
- Murakami K, Honda M, Takemura R, et al. The thermal Z-isomerization-induced change in solubility and physical properties of (all-E)-lycopene. Biochem Biophys Res Commun. 2017;491:317–322.
- Ferruzzi MG, Lumpkin JL, Schwartz SJ, et al. Digestive Stability, micellarization, and uptake of beta-carotene isomers by Caco-2 human intestinal cells. J Agric Food Chem. 2006;54:2780–2785.