ABSTRACT
Di-N-acetylchitobiase (Ctbs) degrades β-1,4 glycoside bonds of the chitobiose core of free asparagine-linked glycan. This study examined whether Ctbs degrades chitin-oligosaccharides to GlcNAc in mammals. We analyzed Ctbs mRNA and protein expression in mouse tissues and characterized enzymatic activity using recombinant mouse Ctbs expressed in Escherichia coli. Ctbs mRNA and protein were expressed in various tissues of mouse, including the stomach. Optimal conditions for recombinant Ctbs were pH 3.0 and 45°C, and the recombinant enzyme was retained more than 94% activity after incubation at pH 3.0–7.0 and below 37°C. The recombinant Ctbs hydrolyzed (GlcNAc)3 and (GlcNAc)6 at pH 3.0 and produced GlcNAc. The Km of Ctbs was lowest with (GlcNAc)3 as a substrate. kcat/Km was fourfold as high with (GlcNAc)3 and (GlcNAc)4 as substrates than with (GlcNAc)2. These results suggest that Ctbs digests chitin-oligosaccharides or (GlcNAc)2 of reducing-end residues of oligosaccharides and produces GlcNAc in mouse tissues.
GRAPHICAL ABSTRACT
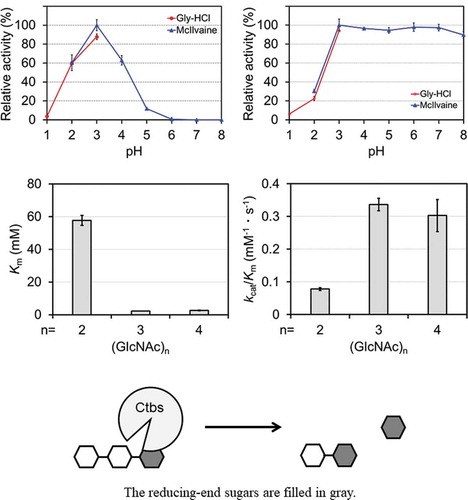
Di-N-acetylchitobiase (Ctbs) is most active at pH 3.0 and retained more than 94% activity after incubation at pH 3.0–7.0 and below 37°C. The preferred substrate of recombinant Ctbs is (GlcNAc)3.
Chitin, a linear β-1,4-linked polymer of N-acetyl-D-glucosamine (GlcNAc), is a major structural component of crustaceans, insects, and fungi; these organisms express chitin synthases [Citation1]. Chitinolytic enzymes hydrolyze the β-1,4 glycoside bonds of chitin. These enzymes are widely distributed not only in animals but also in plants and are involved in morphogenesis, digestion, defense, and pathogenesis [Citation1–3]. In general, chitin-containing organisms exhibit a chitin metabolic pathway and degrade chitin via several chitinolytic enzymes, such as chitinases and chitobiases. Chitinases hydrolyze chitin to GlcNAc oligomers larger than the GlcNAc dimer, i.e. (GlcNAc)2, whereas chitobiases degrade chitin-oligosaccharides to GlcNAc.
Unlike arthropods, mammals do not biosynthesize chitin because of the loss of genes encoding its synthase [Citation1]. However, mammals express the chitinolytic enzymes chitotriosidase (Chit1), acidic mammalian chitinase (Chia/AMCase), and di-N-acetylchitobiase (Ctbs) [Citation4–6]. In mice, mRNA levels of Chit1 are highest in the stomach, followed by the eye and lung [Citation7]. Chit1 degrades chitin and primarily releases (GlcNAc)2 under physiological conditions (pH 4–8 and 37°C) [Citation8]. Mouse Chia is produced in large quantities in the stomach and is most active at pH 2 [Citation5,Citation7,Citation9–12]. Recently, we reported, using in vitro assays, that Chia is expressed as a major protease-resistant glycosidase and primarily degrades chitin to (GlcNAc)2 in the stomachs of omnivorous animals, such as mice, pigs, and chickens, that eat chitin-containing foods, but not carnivores or herbivores [Citation13–16]. These findings suggest that mammals have a chitin metabolic system. Little is known, however, about the enzymes that degrade (GlcNAc)2 produced by mammalian chitinases.
Ctbs was purified from human and rat livers and characterized as an exo-glycoside hydrolase that degrades β-1,4 glycoside bonds of the chitobiose core of free asparagine (N)-linked glycan [Citation6,Citation17–19]. Ctbs hydrolyzes oligosaccharides, including (GlcNAc)2 on the reducing end, and produces a single GlcNAc [Citation19,Citation20]. Ctbs is classified to the glycoside hydrolase (GH) family 18 in the carbohydrate-active enzymes database (CAZy, http://www.cazy.org/), which is defined based on amino acid sequence similarity, together with two mammalian chitinases [Citation5,Citation21,Citation22]. Enzymes of GH 18 family hydrolyze the glycoside bond by a substrate-assisted catalysis mechanism [Citation23,Citation24]. The reaction mechanism for Ctbs with (GlcNAc)2 has been shown that substrate with α-anomeric structure binds to the enzyme and reducing-end GlcNAc of the substrate is released as α-D-GlcNAc by the substrate-assisted catalytic mechanism [Citation20]. However, it is unclear whether Ctbs degrades chitin-oligosaccharides to GlcNAc. In the present study, to understand the role of Ctbs in the degradation of chitin-oligosaccharides, we examined the expression of Ctbs in various mouse tissues. Then, we expressed recombinant mouse Ctbs in Escherichia coli and characterized its enzymatic properties and substrate specificities toward chitin-oligosaccharides. Our results improve understanding of the physiological functions of Ctbs in mammals.
Materials and Methods
RNA and cDNA preparation
Total RNA was prepared from various tissues of 3-month-old male and female C57BL/6 J mice. Mice were sacrificed and small pieces of the tissues (liver, heart, lung, stomach, spleen, kidney, intestine, testis, ovary, brain, and skeletal muscle) were quickly removed. All animal experiments were approved by the Animal Research Committee of the Faculty of Agriculture of Iwate University and were performed in compliance with institutional guidelines. We prepared total RNA from these tissues using TRI Reagent (Cosmo Bio, Kyoto, Japan) according to the manufacturer’s instructions. Total RNA (1 μg) was subjected to reverse transcription with ReverTra Ace® qPCR RT Master Mix with gDNA Remover (Toyobo, Osaka, Japan) according to the manufacturer’s instructions.
qPCR
The mouse Ctbs forward primer (5′-CCCACAGAGCATCTCACTAAAG-3′) and reverse primer (5′-GAGCATCATCGGAGTAGTCAAG-3′) for real-time PCR were designed with PrimerQuest Input (Integrated DNA Technologies, Coralville, IA, USA) and synthesized commercially (Eurofins Genomics, Tokyo, Japan). PCRs were performed in a final volume of 10 µL containing PowerUp SYBR Green Master Mix (Thermo Fisher Scientific, Waltham, MA, USA), 50 ng mouse cDNA, and 3.33 pmol primers. Standard real-time PCR conditions for the Step-one Real-time PCR System (Thermo Fisher Scientific) were as follows: initial denaturation and polymerase activation at 95°C for 10 min, followed by 40 cycles of denaturation at 95°C for 30 s, annealing at 55°C for 30 s, and polymerization at 72°C for 10 s. Melting curves were generated after amplification. Ctbs mRNA levels in murine tissues were determined with the ΔΔCt method and normalized to GAPDH [Citation25]. Real-time PCR of GAPDH was performed with the following primers: forward, 5′-TGAACCACGAGAAATATGACAACT-3′; reverse, 5′-AGTCTTCTGGGTGGCAGTGAT-3′.
Preparation of tissue protein extracts
Tissue protein extracts were prepared from various tissues of 3-month-old male and 4-month-old female C57BL/6 J mice. Ten tissues (liver, heart, lung, stomach, spleen, kidney, intestine, testis, ovary, and brain) were homogenized in 10 volumes of ice-cold 10 mM Tris-HCl (pH 7.4) containing protease inhibitors (Complete Protease Inhibitor Cocktail, Roche Diagnostics, Mannheim, Germany) with a Teflon/glass homogenizer. The homogenates were centrifuged at 15,000 × g for 20 min at 4°C, and the supernatants were pooled. We determined protein concentrations of each fraction with the Quick Start™ Bradford Protein Assay (Bio-Rad Laboratories, Hercules, CA, USA) using a Model 680 Series Microplate Reader (Bio-Rad Laboratories), with bovine serum albumin as a standard.
E. coli expression vector
The mature mouse Ctbs cDNA region without its signal sequence (GenBank Accession Number DQ100384, nucleotides 79–1107) was amplified from the mouse cDNA mixture with KOD Plus DNA polymerase (Toyobo) and oligonucleotide primers (Eurofins Genomics) anchored with the restriction sites EcoRI and XhoI. PCR was performed with the following primers: the forward primer (5′-CATGGAATTCGGACTGCCCGTGCTCCGAGGCTGCT-3′) contained an EcoRI recognition sequence (underlined) and the Ctbs sequence corresponding to Ctbs cDNA; the reverse primer (5′-GTGACCTCGAGCCAGCCTTGGTTTTAAGGCTCCCC-3′) contained an XhoI recognition sequence (underlined) and the Ctbs sequence. The signal sequence and mature sequence of mouse Ctbs were based on NCBI reference sequence: NM_001293672.1. We confirmed that predicted signal peptide sequence of NP_001280601.1 was correspondence with result of Signal IP 5.0 Server (https://web.expasy.org/protparam/). The PCR products were digested with EcoRI and XhoI. To add the V5-His sequence, we subcloned this DNA fragment into pEZZ18/AMCase-V5-His that is removed AMCase sequence [Citation11]. The nucleotide sequence of the resulting plasmid was confirmed by sequencing (Eurofins Genomics).
Preparation of recombinant mouse Ctbs expressed in E. coli
Transformed E. coli BL21 (DE3) (Invitrogen, Carlsbad, CA, USA) strains were grown in LB medium containing 100 µg/mL ampicillin at 37°C for 18 h. Cells were harvested by centrifugation at 7,000 × g for 20 min at 4°C. Cells were suspended in TS buffer (20 mM Tris-HCl [pH 7.6] and 150 mM NaCl) containing a protease inhibitor (Complete Protease Inhibitor Cocktail), sonicated on ice for 10 min, and centrifuged at 10,000 × g for 20 min at 4°C; the supernatant was pooled. This fraction was applied to an IgG Sepharose (GE Healthcare, Buckinghamshire, UK) column equilibrated with TS buffer. After extensive washing, proteins were eluted with 0.1 M Gly-HCl (pH 2.5) followed by neutralization with 1 M Tris-HCl (pH 7.6). Subsequently, recombinant Ctbs-containing fractions were pooled and applied to a hydrophobic interaction chromatography column (TOYOPEARL Butyl-650 M, Tosoh, Tokyo, Japan) equilibrated with 20 mM Tris-HCl (pH 7.6) containing 1 M (NH4)2SO4. Stepwise elution was used with decreasing concentrations of (NH4)2SO4 (0.5, 0.3, 0.2, and 0 M) dissolved in 20 mM Tris-HCl (pH 7.6). Finally, recombinant Ctbs-containing fractions were pooled and subjected to size exclusion chromatography (Sephadex 75 10/300, GE Healthcare). Protein A-Ctbs-V5-His was dialyzed against TS buffer and stored at –80°C.
Antibody preparation
The peptide AREQTQEMWGALKPRL, which corresponds to the C-terminus of mouse Ctbs, was synthesized with an automatic peptide synthesizer (PSSM-8, Shimadzu, Kyoto, Japan). A rabbit polyclonal antibody against this synthetic peptide was generated by T. K. Craft (Gunma, Japan).
SDS-PAGE, CBB staining, and Western blotting
Protein concentrations were determined as described above. The proteins were analyzed with standard SDS-PAGE. The proteins in the gel were visualized by staining with EzStain AQua (ATTO, Tokyo, Japan). Separated proteins were electrophoretically transferred to polyvinylidene fluoride membranes (Immobilon-P, Merck Millipore, Billerica, MA, USA), and the membranes were probed with an anti-V5-HRP monoclonal antibody (Invitrogen), polyclonal anti-mouse Ctbs antibody, or anti-β-actin antibody (Abcam, Cambridge, UK), followed by peroxidase-conjugated goat anti-rabbit IgG (H + L) (Invitrogen). Bound antibodies were detected with ECL Prime Western Blotting Detection Reagent (GE Healthcare) and analyzed with the Luminescent Image Analyzer (ImageQuant LAS 4000, GE Healthcare).
Ctbs activity assays
We measured enzymatic activity of recombinant Ctbs using the synthetic chromogenic substrate, 4-nitrophenyl N-acetyl-β-D-glucosaminide (4NP-GlcNAc; Sigma-Aldrich, St. Louis, MO, USA) at a concentration of 200 µM; The reaction mixture (total 50 µL) contained 1 µL of the substrate solution [10 mM 4NP-GlcNAc in distilled water], McIlvaine buffer at pH 4.0 (0.1 M citric acid and 0.2 M Na2HPO4) [Citation26], and 4 µL of recombinant Ctbs-containing solution (6.29 pmol). The reaction was performed at 37°C for 30 min and terminated by 50 µL of 1 M sodium carbonate. Released 4-NP was measured at 405 nm with the UVmini-1240 (Shimadzu).
We measured recombinant Ctbs activity at 37°C for 30 min using N,N’-diacetylchitobiose, i.e. (GlcNAc)2 (Tokyo Chemical Industry, Tokyo, Japan), as a substrate at a final concentration of 4 mM; The reaction mixture (total 25 µL) contained 2.5 µL of the substrate solution [40 mM (GlcNAc)2 in distilled water], McIlvaine’s buffer (pH 3.0), and 1 µL of recombinant Ctbs. We stopped the reaction by adding 8.3 µL 0.8 M potassium tetraborate. Liberated GlcNAc was measured with the Morgan–Elson reaction [Citation27]. Samples were heated to 100°C for 3 min and cooled in ice, and 250 µL freshly diluted 4-dimethylaminobenzaldehyde (DMAB) reagent was added. DMAB regent was prepared by dissolving 1 g DMAB in 1 mL of concentrated HCl and 9 mL of acetic acid and diluting this reagent 10 times with acetic acid. The samples were incubated at 37°C for 10 min, and absorbance was measured at 585 nm with the UVmini-1240. One unit of enzyme produces 1 μmol of GlcNAc from (GlcNAc)2 per minute. The protein concentration of recombinant Ctbs was measured as described above.
The pH dependence of recombinant Ctbs activity was measured at 37°C for 30 min with (GlcNAc)2 in two buffers as follows: 0.1 M Gly-HCl buffer (pH 1.0–3.0) and McIlvaine’s buffer (pH 2.0–8.0). The reaction mixture (total 25 µL) contained 2.5 µL of the substrate solution [40 mM (GlcNAc)2 in distilled water], 0.1 M Gly-HCl buffer (pH 1.0–3.0) or McIlvaine’s buffer (pH 2.0–8.0), and 1 µL of recombinant Ctbs (1.57 pmol). The reaction was stopped and liberated GlcNAc was measured as described above.
We examined the temperature dependence of Ctbs activity by measuring at 30°C, 37°C, 45°C, 50°C, 55°C, and 60°C for 30 min in McIlvaine’s buffer (pH 3.0). The reaction mixture (total 25 µL) contained 2.5 µL of the substrate solution [40 mM (GlcNAc)2 in distilled water], McIlvaine’s buffer (pH 3.0), and 1 µL of recombinant Ctbs (1.57 pmol). The reaction was stopped and liberated GlcNAc was measured as described above.
To study the pH stability of Ctbs, we placed the enzyme solution (3.14 pmol) in various buffers (pH 1.0–8.0) at 0°C or 37°C for 60 min and measured activity at pH 3.0 in McIlvaine’s buffer, as described above.
Degradation of colloidal chitin and GlcNAc trimer and hexamer by Ctbs
Colloidal chitin was prepared from shrimp shell chitin (Sigma-Aldrich) as described previously and used as a substrate to determine Ctbs activity [Citation5]. Enzymatic reactions using colloidal chitin as a substrate at a final concentration of 2 mg/mL were performed in a volume of 50 μL containing recombinant Ctbs (0.348 nmol) in McIlvaine’s buffer (pH 3.0) for 24 h at 37°C. Enzymatic reactions using GlcNAc trimer or hexamer as substrates at a final concentration of 2 mM were performed in a volume of 50 μL containing recombinant Ctbs in McIlvaine’s buffer (pH 3.0) for 1 h at 37°C. The chitin fragments generated from these reactions were analyzed by FACE as originally described by Jackson [Citation28] and recently improved by us [Citation29]. N-acetyl chito-oligosaccharides (Seikagaku Co., Tokyo, Japan) were used as standards.
Enzyme kinetics
The kinetics of recombinant Ctbs were estimated with different concentrations of (GlcNAc)2 (4–200 mM), (GlcNAc)3 (1–24 mM), and (GlcNAc)4 (1–10 mM) in McIlvaine’s buffer (pH 3.0). Reactions were performed for 30 min at 37°C following the addition of a suitable enzyme aliquot. The Michaelis–Menten constant (Km) was calculated from the Hanes–Woolf plot. The turnover number (kcat) and catalytic efficiency (kcat/Km) were also calculated.
Results and Discussion
Expression of Ctbs in tissues of male and female mice
To determine whether Ctbs plays a role in chitin-oligosaccharide degradation in vivo, we analyzed the expression of mouse Ctbs mRNA in 10 different tissues of five male and five female mice using quantitative PCR (qPCR). Ctbs mRNA was widely expressed in the mouse tissues ( and ). However, a large individual difference was observed in male lung and testis (). Western blotting analysis revealed that Ctbs protein was expressed in the 10 tissues, including the stomach; the Ctbs protein was detected with an anti-Ctbs antibody as a signal of approximately 40 kDa ( and ). Anti-mouse Ctbs antibody recognized a robust protein band in the male and female kidney, followed by male and female spleen, male brain, testis, and ovary. However, the Ctbs protein expression of mouse 10 tissues was not consistent with our data obtained at the mRNA level. Although Ctbs mRNA highly expressed in the testis, a protein band detected at 40 kDa in testis was less than kidney (). In female tissues, Ctbs protein was strongly detected in kidney, followed by ovary and spleen even though the expression of Ctbs mRNA was similar level (). These discrepancies regarding the correlations between mRNA and protein expression of Ctbs may be due to unique localization in each tissue, but further investigations are needed for clarification.
Figure 1. Expression of di-N-acetylchitobiase (Ctbs) in mouse tissues. Ctbs expression was analyzed in different tissues in five male (a) and five female (b) mice by qPCR and are represented in box plot. We quantified the relative expression of Ctbs by qPCR using the ΔΔCt method as described in Materials and Methods. Gene expression of Ctbs is indicated as fold increases with respect to the male liver. (c, d) Ten and five micrograms of soluble proteins were analyzed by Western blotting using anti-mouse Ctbs and anti-β-actin antibodies, respectively

Recombinant Ctbs is expressed in an active form in E. coli
To understand the enzymatic properties of Ctbs, we produced mouse Ctbs in E. coli as a fusion protein containing Staphylococcus aureus Protein A and a V5-His tag ( and Supplemental Figure 1). This mature fusion protein was purified as described in Materials and Methods. Purified recombinant Ctbs was detected by sodium-dodecyl sulfate-polyacrylamide gel electrophoresis (SDS-PAGE) followed by Coomassie Brilliant Blue (CBB) staining as a single protein band with an approximate molecular mass of 56 kDa (). Western blotting analysis with an anti-V5-HRP antibody confirmed that the 56 kDa band was recombinant Ctbs ().
Figure 2. Expression of mouse Ctbs in Escherichia coli. (a) Schematic representation of the E. coli–expressed Protein A-Ctbs-V5-His. Coomassie Brilliant Blue staining (b) and Western blotting (c) of the recombinant protein. Crude, crude extract; IgG, IgG Sepharose fraction; HIC-gel, hydrophobic interaction chromatography and Sephadex G-75 size exclusion chromatography fraction. (d) Recombinant Ctbs was incubated with colloidal chitin at pH 3.0 and 37°C for 24 h. Degradation products were analyzed by fluorophore-assisted carbohydrate electrophoresis (FACE) as described in Materials and Methods

Purification of the recombinant Ctbs yielded approximately 0.096 mg protein per liter of culture. Its enzymatic activity toward 4-nitrophenyl N-acetyl-β-D-glucosaminide (4NP-GlcNAc), which is modified with 4-nitrophenol at the reducing end of GlcNAc, was examined at 37°C and pH 4.0 for 30 min. The recombinant Ctbs did not hydrolyze 4NP-GlcNAc but degraded (GlcNAc)2 under the same conditions. These results are consistent with previous reports that Ctbs was inactive toward substrates modified reducing-end [Citation17,Citation19].
Next, the recombinant Ctbs was incubated with colloidal chitin at 37°C and pH 3.0 for 24 h, and the resulting products were analyzed by fluorophore-assisted carbohydrate electrophoresis (FACE) as described in Materials and Methods. The monomer GlcNAc was the only product detected (). The recombinant Ctbs was inactive toward 4NP-GlcNAc but hydrolyzed colloidal chitin as a natural substrate. These data indicate that the recombinant Ctbs expressed by E. coli is the reducing end GlcNAc-releasing exoglycosidase. These degradation characteristics are consistent with native Ctbs derived from rat liver [Citation6]. Specific activity with (GlcNAc)2 as a substrate was determined to be 2.08 ± 0.44 U/mg.
Although the deduced amino acid sequence of mouse Ctbs has five potential N-glycosylation sites [Citation30], the recombinant Ctbs can exhibit its activity without N-glycosylation. Our results indicate that N-glycosylation as a posttranslational modification is not necessary to express the activated form of Ctbs, consistent with other E. coli–expressed lysosomal enzymes, such as β-glucocerebrosidase, N-acetylgalactosamine-6-sulfatase, and iduronate-2-sulfatase [Citation31–33]. In this study, we expressed mouse Ctbs as fusion protein with Protein A in E. coli. We previously succeeded to express functional mouse AMCase and Chit1 as fusion protein with Protein A in E. coli [Citation8,Citation11]. These recombinant enzymes showed properties similar to the native enzyme from mice or CHO-expressed AMCase and Chit1. In this study, recombinant Ctbs showed properties similar to native enzyme from rat liver [Citation6]. Therefore, it is considered that Protein A has no effect on enzymatic function of Ctbs. Thus, we successfully expressed and purified active recombinant mouse Ctbs in E. coli.
Enzymatic properties of recombinant mouse Ctbs
To characterize recombinant mouse Ctbs, we examined the effects of pH and temperature on enzyme activity using (GlcNAc)2 as a substrate. As shown in , the recombinant Ctbs showed maximum activity at pH 3.0 and 60% maximum activity at pH 2.0 and pH 4.0. Its enzyme activity was low at pH 5.0, at approximately 10%, and was completely lost at pH > 6.0. This result is consistent with previous observations using native Ctbs derived from rats [Citation6].
Figure 3. Characterization of the activity of E. coli–expressed Ctbs. Effects of pH (a) and temperature (b) on recombinant Ctbs activity and the pH stability profile at 0°C (c) and 37°C (d). Values are percentages of the maximum activity obtained in each series of experiments. Values represent means ± standard deviation of triplicate experiments

We determined the effects of temperature on the activity of recombinant Ctbs at temperatures ranging from 30°C to 60°C using McIlvaine buffer at pH 3.0. Maximum activity was observed at 45°C, and activity declined at temperatures above 55°C (). Next, we determined the pH stability of the recombinant Ctbs. It was retained more than 94% activity incubation at pH 1.0–8.0 and 0°C (). By contrast, at 37°C, the recombinant Ctbs was inactivated at pH < 2.0 but was retained more than 94% activity at pH 3.0–7.0 ().
Gastric pH in the mouse is approximately pH 3 and pH 4 in fed and fasted states, respectively [Citation34]. Enzymatic profiling of the recombinant Ctbs suggests that Ctbs can be stable and active in the acidic environment of the mouse stomach in fed and fasted states. The acid dependence and stability of the mouse Ctbs allows it to be most active in the stomach and degrade chitin-containing foods and pathogens in the mouse stomach. Thus, mouse Ctbs has the potential to digest chitin-oligosaccharides produced by Chia in the stomach. However, Ctbs expression in the stomach was lower than kidney (). It is likely that Ctbs functions not so much in stomach as kidney. In kidney of lysosomal diseases, undegraded oligosaccharides are accumulated [Citation35]. Ctbs has been considered that it is responsible for complete degradation of N-linked oligosaccharides [Citation36]. Thus, Ctbs may play important role in degrading (GlcNAc)2 of oligosaccharides in kidney.
Substrate specificities of recombinant mouse Ctbs toward chitin-oligosaccharides
The enzymatic activities of recombinant Ctbs were examined with (GlcNAc)3 and (GlcNAc)6 as substrates. Recombinant Ctbs produced (GlcNAc)2 and (GlcNAc) from (GlcNAc)3 and produced (GlcNAc)5, (GlcNAc)4, (GlcNAc)3, and GlcNAc from (GlcNAc)6 ( and ). These results indicate that Ctbs can hydrolyze chitin-oligosaccharides longer than (GlcNAc)3 and produce GlcNAc.
Figure 4. Degradation of chitin-oligosaccharides by recombinant Ctbs. Recombinant Ctbs was incubated at pH 3.0 and 37°C with (GlcNAc)3 (a) or (GlcNAc)6 (b) for 1 h. Degradation products were analyzed by FACE as described in Materials and Methods

Finally, we determined the kinetic parameters of the recombinant Ctbs for chitin-oligosaccharides, i.e. (GlcNAc)2, (GlcNAc)3, and (GlcNAc)4, at pH 3.0 (). kcat and Km were lowest with (GlcNAc)3 as a substrate. kcat was higher with (GlcNAc)2 as a substrate than with (GlcNAc)3 or (GlcNAc)4. Km was approximately 20 times lower with (GlcNAc)3 and (GlcNAc)4 as substrates than with (GlcNAc)2. kcat/Km with (GlcNAc)3 and (GlcNAc)4 as substrates were approximately fourfold that of (GlcNAc)2. Thus, Ctbs can degrade not only (GlcNAc)2 of free N-glycans but also chitin-oligosaccharides and prefers to degrade (GlcNAc)3 as a substrate.
Table 1. Steady-state kinetic parameters of recombinant Ctbs at pH 3.0 and 37°C
Chitin-oligosaccharides are likely to be produced by Chia from chitin-containing material in the stomach [Citation8,Citation13–16]. Our results suggest that Ctbs digests chitin-oligosaccharides produced by Chia to GlcNAc in the mouse stomach. The degradation product of chitin, GlcNAc, may be a source of carbon, nitrogen, and energy. We are currently conducting in vivo experiments to determine whether Ctbs digests chitin along with Chia in the stomach.
Author contributions
M.O., M.M., and F.O. conceived of the presented idea. M.O., M.M., M.K., M.S., and T.Y. designed the experiments. M.O., M.K., and Y.M. performed the experiments. M.O. analyzed data. M.O., M.M., and T.Y. wrote the manuscript. M.O., M.M., M.K., Y.M., M.S., F.O., and T.Y. contributed to the critical appraisal of the manuscript and approved the final version.
Supplementary_Figure_BBB.pdf
Download PDF (60.3 KB)Acknowledgments
We are grateful to Dr. Kazuaki Okawa, Dr. Akinori Kashimura, Dr. Satoshi Wakita, Dr. Shotaro Honda, and Dr. Yasutada Imamura for valuable suggestions.
Disclosure statement
No potential conflict of interest was reported by the authors.
Supplementary material
Supplemental data for this article can be accessed here
Additional information
Funding
References
- Koch BE, Stougaard J, Spaink HP. Keeping track of the growing number of biological functions of chitin and its interaction partners in biomedical research. Glycobiology. 2015;25:469–482.
- Cohen-Kupiec R, Chet I. The molecular biology of chitin digestion. Curr Opin Biotechnol. 1998;9:270–277.
- Shaikh SA, Deshpande MV. Chitinolytic enzymes: their contribution to basic and applied research. World J Microbiol Biotechnol. 1993;9:468–475.
- Hollak CE, van Weely S, van Oers MH, et al. Marked elevation of plasma chitotriosidase activity. A novel hallmark of Gaucher disease. J Clin Invest. 1994;93:1288–1292.
- Boot RG, Blommaart EF, Swart E, et al. Identification of a novel acidic mammalian chitinase distinct from chitotriosidase. J Biol Chem. 2001;276:6770–6778.
- Kuranda MJ, Aronson NN Jr. A di-N-acetylchitobiase activity is involved in the lysosomal catabolism of asparagine-linked glycoproteins in rat liver. J Biol Chem. 1986;261:5803–5809.
- Ohno M, Tsuda K, Sakaguchi M, et al. Chitinase mRNA levels by quantitative PCR using the single standard DNA: acidic mammalian chitinase is a major transcript in the mouse stomach. PLoS One. 2012;7:e50381.
- Kimura M, Wakita S, Ishikawa K, et al. Functional properties of mouse chitotriosidase expressed in the periplasmic space of Escherichia coli. PLoS One. 2016;11:e0164367.
- Boot RG, Bussink AP, Verhoek M, et al. Marked differences in tissue-specific expression of chitinases in mouse and man. J Histochem Cytochem. 2005;53:1283–1292.
- Ohno M, Togashi Y, Tsuda K, et al. Quantification of chitinase mRNA levels in human and mouse tissues by real-time PCR: species-specific expression of acidic mammalian chitinase in stomach tissues. PLoS One. 2013;8:e67399.
- Kashimura A, Okawa K, Ishikawa K, et al. Protein A-mouse acidic mammalian chitinase-V5-His expressed in periplasmic space of Escherichia coli possesses chitinase functions comparable to CHO-expressed protein. PLoS One. 2013;8:e78669.
- Kashimura A, Kimura M, Okawa K, et al. Functional properties of the catalytic domain of mouse acidic mammalian chitinase expressed in Escherichia coli. Int J Mol Sci. 2015;16:4028–4042.
- Ohno M, Kimura M, Miyazaki H, et al. Acidic mammalian chitinase is a proteases-resistant glycosidase in mouse digestive system. Sci Rep. 2016;6:37756.
- Tabata E, Kashimura A, Wakita S, et al. Protease resistance of porcine acidic mammalian chitinase under gastrointestinal conditions implies that chitin-containing organisms can be sustainable dietary resources. Sci Rep. 2017;7:12963.
- Tabata E, Kashimura A, Wakita S, et al. Gastric and intestinal proteases resistance of chicken acidic chitinase nominates chitin-containing organisms for alternative whole edible diets for poultry. Sci Rep. 2017;7:6662.
- Tabata E, Kashimura A, Kikuchi A, et al. Chitin digestibility is dependent on feeding behaviors, which determine acidic chitinase mRNA levels in mammalian and poultry stomachs. Sci Rep. 2018;8:1461.
- Stirling JL. Human N-acetyl-β-hexosaminidases: hydrolysis of N, N’ diacetylchitobiose by a low molecular weight enzyme. FEBS Lett. 1974;39:171–175.
- Stirling JL. NN’-diacetylchitobiase activity in Tay-Sachs disease and Sandhoff’s disease. Biochem J. 1974;141:597–599.
- Aronson NN Jr., Backes M, Kuranda MJ. Rat liver chitobiase: purification, properties, and role in the lysosomal degradation of Asn-linked glycoproteins. Arch Biochem Biophys. 1989;272:290–300.
- Aronson NN Jr., Halloran BA. Optimum substrate size and specific anomer requirements for the reducing-end glycoside hydrolase di-N-acetylchitobiase. Biosci Biotechnol Biochem. 2006;70:1537–1541.
- Boot RG, Renkema GH, Strijland A, et al. Cloning of a cDNA encoding chitotriosidase, a human chitinase produced by macrophages. J Biol Chem. 1995;270:26252–26256.
- Liu B, Ahmad W, Aronson NN Jr. Structure of the human gene for lysosomal di-N-acetylchitobiase. Glycobiology. 1999;9:589–593.
- Terwisscha van Scheltinga AC, Armand S, Kalk KH, et al. Stereochemistry of chitin hydrolysis by a plant chitinase/lysozyme and x-ray structure of a complex with allosamidin evidence for substrate assisted catalysis. Biochemistry. 1995;34:15619–15623.
- van Aalten DMF, Komander D, Synstad B, et al. Structural insights into the catalytic mechanism of a family 18 exo-chitinase. Proc Nat Acad Sci. 2001;98:8979–8984.
- Livak KJ, Schmittgen TD. Analysis of relative gene expression data using real-time quantitative PCR and the 2(-Delta Delta C(T)) Method. Methods. 2001;25:402–408.
- McIlvaine TC. A buffer solution for colorimetric comparison. J Biol Chem. 1921;49:183–186.
- Reissig JL, Storminger JL, Leloir LF. A modified colorimetric method for the estimation of N-acetylamino sugars. J Biol Chem. 1955;217:959–966.
- Jackson P. The use of polyacrylamide-gel electrophoresis for the high-resolution separation of reducing saccharides labelled with the fluorophore 8-aminonaphthalene-1,3,6-trisulphonic acid. Detection of picomolar quantities by an imaging system based on a cooled charge-coupled device. Biochem J. 1990;270:705–713.
- Wakita S, Kimura M, Kato N, et al. Improved fluorescent labeling of chitin oligomers: chitinolytic properties of acidic mammalian chitinase under somatic tissue pH conditions. Carbohydr Polym. 2017;164:145–153.
- Balducci C, Bibi L, Berg T, et al. Molecular cloning and structural organization of the gene encoding the mouse lysosomal di-N-acetylchitobiase (ctbs). Gene. 2008;416:85–91.
- Hantzopoulos PA, Calhoun DH. Expression of the human alpha-galactosidase A in Escherichia coli K-12. Gene. 1987;57:159–169.
- Mosquera A, Rodríguez A, Soto C, et al. Characterization of a recombinant N-acetylgalactosamine-6-sulfate sulfatase produced in E. coli for enzyme replacement therapy of Morquio A disease. Process Biochem. 2012;47:2097–2102.
- Morales-Álvarez ED, Rivera-Hoyos CM, Baena-Moncada AM, et al. Low-scale expression and purification of an active putative iduronate 2-sulfate sulfatase-Like enzyme from Escherichia coli K12. J Microbiol. 2013;51:213–221.
- McConnell EL, Basit AW, Murdan S. Measurements of rat and mouse gastrointestinal pH, fluid and lymphoid tissue, and implications for in-vivo experiments. J Pharm Pharmacol. 2008;60:63–70.
- Stinchi S, Lüllmann-Rauch R, Hartmann D, et al. Targeted disruption of the lysosomal α-mannosidase gene results in mice resembling a mild form of human α-mannosidosis. Hum Mol Genet. 1999;8:1365–1372.
- Park C, Meng L, Stanton LH, et al. Characterization of a human core-specific lysosomal α1,6-mannosidase involved in N-glycan catabolism. J Biol Chem. 2005;280:37204–37216.