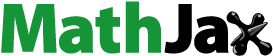
ABSTRACT
We investigated the hypothesis that a daily-variation exists for a 4-km time-trial, where a standardised-approach has been employed. Twenty-six male students completed a 30-s Wingate test, a O2 peak test: and then three 4-km familiarisations at 12:00 h. Two further time-trials counterbalanced for start at 07:30 or 17:30 h were undertaken. There was 72 h between each session. Heart rate, rectal and skin temperature values, rating-of-perceived-exertion, effort, pacing perception and split-times were measured every 1-km during the time-trial. Blood lactate, urine osmolarity, rectal and skin temperature were taken at rest and immediately after each time-trial. Evening finishing times, resting rectal temperatures, pre-post time-trial blood lactate were faster, higher with a greater rise (~10 s, 0.48°C, 0.7mmol.L) than morning values (p<0.05) with no other interactions. In summary, where a standardised approach has been employed 4-km performance was 2.6 % faster in the evening than the morning (ES = 0.34, power = 0.8).
Introduction
Physiological variables related to gross motor performance follow a daily variation with greater muscle force production and power output in the evening, compared to the morning (Drust et al. Citation2005; Edwards et al. Citation2013; Pullinger et al. Citation2019; Ravindrakumar et al. Citation2022). Time-trials are an exemplar of measuring sporting performance and the criterion for performance is predominantly distance (fixed) and the time to finish (variable). Currently, the scheduling of start time for time-trials can be at any time of day dependent on a number of factors, mostly outside that of the control of the athlete (availability of space, allocation of start by the organisation, influenced by maximal viewing by broadcasting companies).
An effect of time of day on time-trial and maximal performance has been reported since the early 1970’s (Conroy and O’Brien Citation1973). In elite sport the current advice is to schedule the finals (Olympic or World championships) in the evening, to maximise the chance of athletes breaking records (Lok et al. Citation2020). The only caveat to this is for long duration competition, where environmental temperature may be high and the risk of heat stroke is prevalent, thus advocating a start time in the morning (Drust et al. Citation2005). Although evidence for an evening superiority and hence scheduling of competition start time reflect this (Lok et al. Citation2020), a significant improvement in the evening compared to the morning is not consistently found in the literature (Bommasamudram et al. Citation2022; Knaier et al. Citation2022). In laboratory-based research this lack of agreement is thought to be partially explained due to a lack of rigor and standardisation in method employed, number and choice of population, a lack of control for important factors which specifically relate to investigations of chronobiological nature and other considerations. With a surge in systematic and meta-analytical reviews, differences in research findings can now be considered in a systematic way, with a critical appraisal tool used to assess the methodological quality of studies and risk of bias. Bruggisser et al. (Citation2023) highlighted that the main biases in individual studies are “bias in measurement of the outcome” and “bias in selection of the reported result”; with a lack of large and well powered studies. These concerns are not new in exercise related chronobiological studies (Winget et al. Citation1985; Youngstedt and O’Connor Citation1999; Reilly et al. Citation2000; Drust et al. Citation2005; Araujo et al. Citation2011; Knaier et al. Citation2022) and a recent systematic review highlights a rigorous, standardised approach needs to be adopted by future investigations regarding measuring a possible diurnal variation in time-trial performance (Bommasamudram et al. Citation2022).
Although there is currently no consensus about what exactly a standardised approach should entail for investigation or adopted practical guidelines. Youngstedt and O’Connor (Citation1999) attributed a number of factors to explain daily variation in performance: differences in nutritional status where participants are generally fasted in the morning; decreased flexibility and increased stiffness in the morning; insufficient time to recover from sleep inertia in the morning; habitual training time conveying an advantage; differences in the amount of rest between test sessions; smaller changes in body temperature in the morning; and differences in motivation and expectancy effects. Further, Drust et al. (Citation2005) stated that to find daily variations the signal to noise ratio had to be reduced by ensuring the measurement techniques are accurate (that the gold standard methods to measure the outcomes are used), by ensuring the sample size is appropriate. Where the design of the experiment incorporates familiarisation of the performance tests, randomisation should be used to eliminate selection bias and balance the groups with respect to many known and unknown confounding or prognostic variables. A counter balanced order design should be employed, to statistically distribute any residual learning effects; and there should be enough time between each test to recover from fatigue (see reviews Knaier et al. Citation2022; Bruggisser et al. Citation2023). And lastly, Yousefzadehfard et al. (Citation2022) in a recent review on circadian rhythms research in humans, recommended participant screening (sleep routines, medication use and rhythm disturbance) and protocol considerations (including light settings, posture, exercise undertaken prior to and during the research period and dietary habits) as well as practical guidelines for inclusion/exclusion criteria intended to reduce confounding variables.
Whilst it is difficult for one study to address all the factors listed above, we sought to consider some of these methodological concerns as well as remove others by participant selection and protocol design to reduce bias (See ). We aimed to test the hypothesis that a daily variation exists for a 4-km cycling time-trial in a healthy population of highly motivated male adults, using a standardised protocol. We hypothesised that finishing times would be lower in the evening than the morning.
Table 1. Checklist of considerations in chronobiological studies on humans and sporting performance for participant (1–3), methodological and equipment (4–8) and environmental (9) considerations and general comments and insights into decision for our study.
Materials and methods
Participants
Twenty-six “recreationally active” adult males as classified by the “Participant Classification Framework” (McKay et al. Citation2021) took part in the study (mean ± SD) and [range]: age = 22.0 ± 2.6 [18–28] yrs, body mass = 73.4 ± 7.8 [56–91] kg, height = 179.7 ± 12.4 [163–188] cm, O2 peak = 48.5 ± 7.8 [35–62] mL.kg.min−1, 30 s peak power output from a Wingate test of 950 ± 150 [601–1300] W and habitual total sleep time = 8.6 ± 0.7 [7–10] h. All recruited from the student population of the University Sport Science department, with criteria for selection >5 years of cycling competition experience, injury-free with no diagnosed sleep disorders and not completed shiftwork or travel outside the local time-zone in the past month. Participants had to be healthy males (18–30 years), habitually retire between 22:00–23:30 h and rise at 06:00–07:30 h and agree to retire to bed at 22:30 and rise at 06:30 h; which is not too dissimilar to their natural sleep patterns. None of the participants were receiving any pharmacological treatment (including non-steroidal anti-inflammatory drugs, NSAIDs) throughout the study period. Habitual caffeine consumption was assessed using the caffeine consumption questionnaire (CCQ) and those with >150 mg per day were excluded (Landrum Citation1992). Further, all participants expressed no preference to training regarding time of day by a weekly self-reported 2-week training diary. The circadian chronotype of the participants was assessed using a composite “morningness questionnaire” by Barton et al. (Citation1990). The participants’ mean “chronotype” score on a 13–52 scale was 34 ± 4 [28–39]; hence, all the participants were “intermediate types”. Participants were required to arrive fasted and abstain from alcohol, caffeine and exercise 24 h preceding a testing session. All participants gave their written informed consent. The study was conducted in accordance with the Local Ethics committee at JMU (approval number U19SPS024), the ethical standards of the journal and complied with the Declaration of Helsinki.
Experimental design
The participants attended the environmentally controlled chamber (Edge, Nottingham, UK) on seven occasions, the chamber was held at a dry temperature, humidity, barometric pressure and ambient light of 19°C, 35–45%, 750–760 mmHg and 750 lux, respectively for all sessions. Only one person completed the time trial in the chamber at a given time. Each participant completed with a minimum of 72 h to ensure recovery between trials a) three familiarisation sessions (detailed below); b) a O2 peak/max test on a cycle ergometer’; c) Wingate cycle test; and lastly d) two experimental sessions three days apart: a morning and an evening trial (07:30 and 17:30 h; M and E; see ), with volunteers allocated into two groups (named M and E) equally based on physical ability for first and second session allocation. Practically this entailed stacking the finishing times for the last familiarisation session from fastest to slowest in Microsoft Excel, then pasting the words M or E respectively so the first cell (fastest participant) was M and next was E all the way down the column. The experimental sessions were then counterbalanced in order of administration to minimise any potential learning effects (Monk and Leng Citation1982). All experiments were completed between the months of October to December (Autumn to Winter in the UK) to ensure the individual’s exposure to sunlight in the mornings when entering the laboratories was <80 Lux (Light-watch, Neurotechnologies, Cambridge, UK), with sunrise and sunset range from start to the end of the experiment being 07:29 to 08:01 h and 18:01 to 19:50 h respectively.
Figure 1. Schematic of the research design and protocol for conditions undertaken in the morning (07:00 h) or evening (17:00 h). Rectal and skin (Tr and Tsk) temperature and ratings of perceive exertion (RPE) were measured after the participants had reclined for 30-in at the start of the protocol and after the warm-up. Tr and tsk, RPE and HR values were also measured prior to taken every 1-km and the 4 km-time trial in the environmental chamber; with pacing asked every 2-km. Black dotted lines indicate blood taken. * indicates sleep questionnaires administered. BOP =Bridget’s optimal pacing scale.
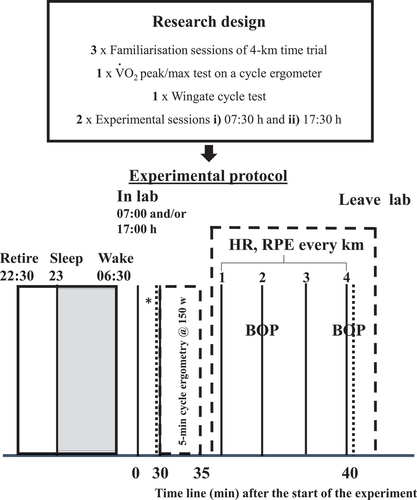
Protocol and measurements
Familiarisation: Participants were accepted as familiarised when 4-km TT finishing times varied by no more than coefficient of variance 5% to reduce the chances of a learning effect affecting performance. The random error between the three initial reliability trials was heteroscedastic and the data were therefore expressed as 95% ratio limits of agreement. For simplicity, only the 95% ratio limits of agreement for the second and third 4-km time-trial performance were investigated for the 26 participants; these were */÷ 1.053 (about 5.3 %) and coefficient of variance of 2.71 %, indicating little measurement error (Atkinson et al. Citation1998; Atkinson and Nevill Citation1998). Paired t-test showed no difference (t25 = −0.519, p=0.608) and practically this was a 0.4 % increase in finishing time. One week after these preparatory tests, volunteers underwent the experimental data collection sessions.
Subsequently, participants completed a O2 peak/max test using the Metalyser 3B (Cortex GmbH, Leipzig, Germany). Tests were conducted on cycling ergometers (Excalibur Sport, Lode, Groningen, Netherlands) with reusable masks (7450 Series Silicone V2, Hans-Rudolph Inc., KS, U.S.A.) following the BASES guidelines (Hale et al. Citation1988). Five min of resting data were collected before participants completed a 5 min warm-up (WU) at 150 W, which also served as the first testing stage. Resistance was then increased by 30 W every 3 min until volitional exhaustion. The test will be referred to a “peak” rather than “max” if the participants did not reach the criteria of a plateau in the
O2 data between the last and penultimate stage. To assess anaerobic capacity volunteers also participated in a Wingate test (Ayalon et al. Citation1974) using Wattbike cycle ergometers (Wattbike Pro, Nottingham, UK). The protocol included a 5 min WU at 150 W with three phases of 6 s at 60, 70 and 80% of maximal perceived cadence. Following the WU participants completed the 30 s Wingate test adjusted for body mass.
Protocol for the experimental sessions
The main experiment consisted of two visits: a M and an E trial (07:30 and 17:30 h) in the environmental chamber. Participants woke at 06:30 h to come into the laboratory at 07:00 h for both conditions having fasted and then completed the sleep questionnaires. If they were in the evening condition, they then were able to and were asked not to consume food 4 h before the start of the evening session (Atkinson and Reilly Citation1996). In all sessions the participants retired at around 22:30 h and rose around 06:30 h, having had a text message which they had to respond to. Compliance was high, with all confirming they had adhered to the schedule as assessed by the text response and verbal check in the morning and evening of the experimental sessions. The volunteers were free to live a “normal life”, between sessions sleeping at home at night; and attending lectures and doing light office work in the day. They were asked to refrain from any other training or heavy exertion for the 48 h before the experiments or during them. Participants recorded the type, amount, and timing of the food they ate for the period of 24 h before the day of the first session and were asked to replicate this diet for the days before the second experimental condition. Water and non-caffeinated/non-alcoholic, calorie-free beverages were allowed ad libitum up to a total volume of 3 L per day.
Measurement procedures
On arrival to the laboratory, 30 min before the tests, participants were asked to insert a flexible soft rectal probe (mini-thermistor, Grant Instruments, Shepreth, UK) ∼10 cm beyond the external anal sphincter. Individuals then rested in a supine position to measure resting rectal temperature (Tr). To assess skin temperature (Tsk) during the 30 min resting period, skin thermistors (Grant Instruments, Squirrel 2010 series, UK) were attached at four different locations on the left side of the body (chest[ch], forearm[f], thigh[th] and calf[ca]). Mean Tsk was calculated using the equation proposed by Ramanathan (Citation1964): Tsk = (0.34 x Tch) + (0.33 x Tth) + (0.18 x Tca) + (0.15 x Tf). Mean body temperature (Tmb) was calculated as [(0.64 x Tr) + (0.36 x Tsk), (Burton Citation1935)]. Resting temperatures for Tr and Tsk were recorded as the mean of the last 5 min of the 30 min of rest.
Near-infrared spectroscopy (NIRS) measures relative changes in oxy- and deoxyhaemoglobin and can be used during both passive and exercise activities. This non-invasive procedure (using small devices that are tapped to the skin over the belly of the muscle under investigation) provides information on skeletal muscle oxygenation and oxidative energy metabolism during exercise, as well as training-induced adaptations (Jones et al. Citation2016). Due to equipment failure only 17/26 participants had NIRS devices (Moxy Monitor, Hutchinson, MN, U.S.A.) placed on the opposite side of the body to the skin thermistors, over the belly of the brachioradialis (forearm), vastus lateralis (thigh) and medial head of the gastrocnemius (calf) to measure muscle oxygenation (SmO2). All participants also provided urine samples upon entering the laboratory and after the TT. To determine volunteer’s hydration status urine samples were analysed using an osmometer (Pocket PAL-OSMO, Vitech Scientific, Japan). During the resting period participants completed questionnaires on thermal comfort (TC; Bakkevig and Nielsen Citation1994), rate of perceived exertion (RPE; Borg Citation1973), effort (on a 0–10 cm scale “0” meaning no and “10” maximal effort), profile of mood states (POMS; McNair and Lorr Citation1971) and their subjective ratings of sleep (sleep questions taken from Liverpool Jet lag Questionnaire; Waterhouse et al. Citation2005). Participants were also asked to put on a heart rate (HR) monitor (HRM duel, Garmin, China) and a pulse oximeter on their index finger (Carescape V100, GE Healthcare, UK) to measure peripheral oxygen saturation (SpO2). Lactate levels were assessed at rest and post TT (Biosen C-Line Glucose and Lactate Analysers, EKF Diagnostics, Cardiff, UK). Following the rest period, participants completed a 5 min WU at 150 W (Winter et al. Citation2007), on a cycle ergometer (Excalibur Sport, Lode, Groningen, Netherlands). During the last 30 s of the WU Tr, Tsk, RPE, TC, effort, HR and SpO2 were recorded. After the WU participants mounted a Wattbike Pro cycle ergometer to complete a 4 km TT which has a ±1% error in measuring distance. Initially the load was set at magnetically resistance control lever level 1 (represents a level road) and wind braked Resistance System 0 (low resistance) and the participants adjusted the wind braking levels as they deemed appropriate and had previously practiced. During the TT’s a fan (CAM5002, Clarke, Essex, UK) was positioned diagonally to the front right of the ergometer, 2 m away from the participants face. Airflow was determined by the cyclist and replicated for the subsequent trial. Strong and consistent verbal encouragement was provided throughout the TT and volunteers were instructed to finish as quickly as possible. Tr, Tsk, RPE, TC, effort, HR and SpO2 were recorded every 1 km completed. At 2 and 4 km participants were asked to verbally rate their pacing (Bridget’s Optimal Pacing scale; BOP) corresponding for that 2 km, relative to that required to finish as quickly as possible (−5, “too slow” to +5, “too fast”). Participants were blinded from the clock to limit competing against a previous finishing time, but were provided with feedback on distance covered, watts (W) and cadence (rev.min−1) via the display (Edwards et al. Citation2005; Gough et al. Citation2017).
Statistical analysis
Our primary outcome was the time to complete the 4 km time-trial. Such protocols have been found to be highly reliable with test-retest coefficients of variation (CV) < 2 % like our 2.7 % familiarisation data (Batterham and Atkinson Citation2005). Using the G*Power 3.1.9.6 software (Faul et al. Citation2007), it was estimated that 18 participants would facilitate the detection of a statistically significant difference between morning and evening time-trial of 6.8 % assuming an effect size (ES) of 0.630 (Fernandes et al. Citation2014), p < 0.05 and 80% power with a one-tailed paired t-test (where evening gross muscular performance is normally greater than morning) and 23 volunteers required with a 90% power. We took the more conservative approach and recruited 26 participants to allow for dropout and power of 90%. Data were analysed using the Statistical Package for Social Sciences version 29 (SPSS, Chicago, IL, U.S.A.). All data were checked for normality using the Shapiro-Wilk test. General linear models were used for all measurements collected. To correct sphericity violations the degrees of freedom were corrected using Greenhouse-Geisser (ε < 0.75) or Huynh-Feldt (ε > 0.75). Bonferroni pairwise and graphical comparisons were made where main effects were found. Results are presented as mean ± standard deviation (SD) unless otherwise stated. Significance was set at p < 0.05. Effect sizes were calculated from the ratio of the mean difference to the pooled standard deviation. The magnitude of the ES was classified as trivial (≤0.2), small (>0.2–0.6), moderate (>0.6–1.2), large (>1.2–2.0) and very large (>2.0) based on guidelines from Batterham and Hopkins (Citation2006). The results are presented as the mean ± the standard deviation throughout the text unless otherwise stated. Ninety-five percent confidence intervals are presented where appropriate as well as the mean difference between pairwise comparisons. Linear regression with Pearson correlation was used to verify the associations between all dependent variables.
Results
Rest and post warm-up variables
There was a significant time-of-day difference with higher Tr (mean difference = 0.44 °C, p < 0.0005, 95% CI: 0.34–0.64 °C; ES = 1.44) and Tmb (mean difference = 0.36 °C, p < 0.0005, 95% CI: 0.26–0.45 °C; ES = 0.38) values in the E compared to the M condition. There was a significant pre-post effects of warm-up only in muscle oxygen values (SmO2) in the thigh which decreased post warm-up (mean difference = 11.7 %, p = 0.014, 95% CI: 2.7–20.6 °C; ES = 0.07). Tsk values were no different in E than the M (1.07 °C; p = 0.076; ). No interaction was observed for any variable such that the profiles for M or E values rose or fell in the same way from rest to post warm-up. The presence of a diurnal difference in rectal temperature agrees with other work.
Table 2. Mean (SD) values for morning (M) and evening (E) for resting or resting and post warm-up. Significance (P-value) for main effects for time of day (DV), pre-post warmup (WUP) and the interaction (DV x WUP) are given. Statistical significance (p < 0.05) is indicated in bold. * indicates a significant DV.
Responses to TT performance (Finishing time [FT], split times/km, mean power output/km [PO]), HR, TC, BOP, Effort, Tr, Tsk, Tbt, Urine osmolarity and blood lactate)
There was a significant main effect for time of day (TOD) for FT, split times and PO (p < 0.05, ). Participants were significantly faster in completing their time-trial in E than in M (∼10 s, 2.59 % difference, p = 0.001, 95% CI: 4.6–15.3 s; ES = 0.34; actual power = 80 %; See and ) with corresponding quicker split times per km (2.5 s difference, p = 0.001, 95% CI: 1.2–3.8 s; ES = 0.34) and greater PO (9.1 W difference, p = 0.013, 95% CI: 2.1–16.1 W; ES = 0.23). Blood lactate higher in E than M (0.68 mmol.L difference, p < 0.0005, 95% CI: 0.25–1.11 mmol.L; ES = 0.68). Rectal temperature was higher in E than M (0.32 °C difference, p < 0.0005, 95% CI: 0.21–0.44 °C; ES = 0.99), as was the Tmb values (0.27 °C difference, p < 0.0005, 95% CI: 0.16–0.38 °C; ES = 0.40). There were no time-of-day effects for the other variables (). The presence of a diurnal variation in finishing time addresses a principle aim of this research project.
Figure 2. Individual and mean (±95% CI) values for the finishing times in the evening and morning for a 4 km time-trial.
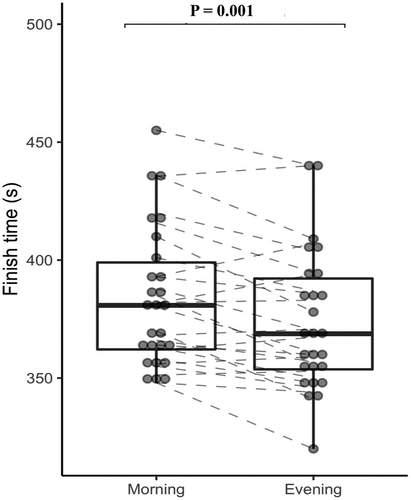
Figure 3. Mean (SD) values for pacing (s), power output (W), heart rate (BPM) and rating of perceived exertion (RPE) every 1-km for the 07:30 and 17:30 h for a 4-km time-trial.
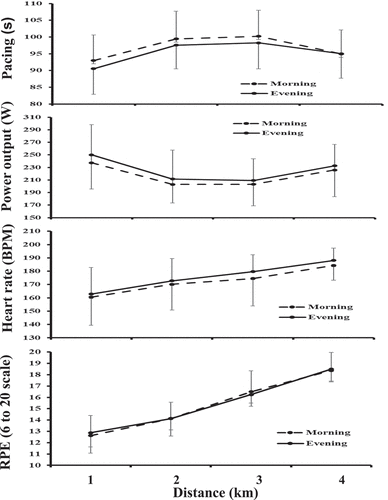
Table 3. Mean (SD) values for morning (M) and evening (E) for pre-post or during the 4 km TT. Significance (P-value) for main effects for time of day (DV), distance or pre-post and the interaction of (DV x distance or DV x pre-post) are given. Statistical significance (p < 0.05) is indicated in bold * indicates a significant DV. Where RPE = rating of perceived exertion and TC = thermal comfort.
There was a significant main effect for distance where split times and PO started high in the first km, dropped to plateaued from 2–3 km and then increase from 3–4 km (p < 0.05). Further HR, Tr, Tmb, RPE and rating of effort increased from the start to the finish of the TT ( and ). Pulse oximetry and SmO2 for forearm, thigh and calf values decreased from 2 km to 4 km (). Blood lactate and urine Osmol values were higher post than pre (11.43 mmol.L and 114 Osmol difference respectively, p < 0.05). A significant interaction between TOD and TT was only seen in blood lactate values with a greater rise in E post exercise than M (p = 0.012, ).
Resting POMS and sleep questionnaire variables
There was a significant TOD difference with lower ratings of mood state of fatigue (mean difference = 1.5, p = 0.049, 95% CI: 0.006–2.917; ES = 0.49) and higher rating of vigour values (mean difference = 1.9, p = 0.001, 95% CI: 0.81–2.96; ES = 0.79) in the E compared to the M condition (). There were no main effects for TOD for any other mood or responses to the sleep questionnaire (). We have previously reported similar values in mood-states across the day. That no difference was found in any subjective sleep questionnaires answered in both conditions at 07:00 h means 1) no difference between the protocol and normal habits and 2) no difference between sleep assessment between conditions.
The associations between all dependent variables change in M/E finishing time and average finishing time, O2 peak and Wingate/kg variables. Linear correlation analysis of the score of all the cyclists considered as a single group, showed significant correlations were not detected between diurnal variation finishing time (%) and i) average finishing time (r = −0.004, p = 0.771), ii)
O2 peak (r = 0.131, p = 0.069) also, iii) Wingate scores (r = 0.023, p = 0.463).
Discussion
We report that in a large and well powered study of highly motivated, male recreationally active cyclists the 4-km TT finishing times were significantly faster in the evening than in the morning (~ 10 s, Δ 2.59%; p = 0.001; actual power = 80%). These individuals followed a standardised protocol that incorporated some of the main concerns in the literature (), had a range of aerobic, anaerobic fitness and finishing times. This diurnal variation was accompanied by a decrease in split times per km and a corresponding increase in power output (Δ 2.5 s and Δ 9.1 W respectively). Rectal and Tmb temperature and blood lactate values were significantly higher at rest, and after exercise in the evening than the morning as expected and in agreement with the literature (Edwards et al. Citation2023). Considering only research that employed time-trials in a thermoneutral laboratory (~19–21°C), in adult males, where the distance is fixed and the time to finish is variable – our findings agree with 5/9 of the previous research (removing Baxter and Reilly Citation1983; Dalton et al. Citation1997, Boukelia et al. Citation2018; Boukelia et al. Citation2017; Silveira et al. Citation2020). Faster swimming (100 m Δ 2.6%, Martin et al. Citation2007), 1 km cycling (Δ 2.3%, Fernandes et al. Citation2014), 10 km cycling (Δ 2.3%, Souissi et al. Citation2021), 16.1 km cycling (Δ 3.6%, Atkinson et al. Citation2005), or 10-km time-trial running on a treadmill (Δ 6.3%, Edwards et al. Citation2008) was found in the evening than in the morning. Although, it is challenging to compare our findings directly with others as there are discrepancies between studies in approaches.
The idea of a standardised approach as we have undertaken has been recently called for in human chronobiology research (Yousefzadehfard et al. Citation2022; Knaier et al. Citation2022; Bruggisser et al. Citation2023); and specifically in diurnal variation in time-trial investigations (Bommasamudram et al. Citation2022). We collated the advice of several sources to reduce bias (of measurement, and reported result) by i) participant considerations, ii) methodological and equipment considerations, iii) environment considerations (see ). In the design of the current study, we applied this advice to best reduce the signal to noise ratio, to allow a diurnal variation should it exist to be found. For some recommendations, we have been pragmatic, so we did not attempt to blind the researchers as well as the participants to the conditions as suggested by others (Knaier et al. Citation2022), nor adopt time of days of measurement such as 04:00–05:00 h (body temperature minimum) that would cause sleep loss.
In the 9 studies from the literature (), sample sizes ranged from 8 to 22 with a priori power tests done on only 3/9 papers, training status is either quantified or just stated, a method of categorising fitness such as McKay et al. (Citation2021) would help comparison of populations between studies. Further only 5/9 papers recorded metrics of fitness such as O2 peak or Wingate power output data again enabling population comparisons between studies. The chronotype scores of the population were recorded in 6/9 studies, but habitual sleep time was only reported in one study; where recruiting a participant whose sleep habits are vastly different to those imposed by the protocol could cause detriments to the outcome measures. In more recent work it has been highlighted the inclusion/exclusion criteria to reduce confounding variables in studies should also include “not” receiving pharmacological treatment (including NSAIDs); a habitual caffeine “low” consumption, hence <150 mg per day assessed by the caffeine consumption questionnaire as well as drug and alcohol considerations (See Yousefzadehfard et al. Citation2022). The distance of the time-trials ranged from 100 m to 16.1 km and mode of exercise was either swimming, running or cycle ergometry. Allocation to groups was generally randomised and counterbalanced, with the time between sessions being 36 h to 5 days to allow recovery. Diet restrictions were considered in 5/9 studies following the general advise of Drust et al. (Citation2005), therefore we similarly chose to follow this where participants recorded the type, amount, and timing of the food they ate for the period of 24 h before the day of the first session and were asked to replicate this diet for the days before the second experimental condition. Individuals came in fasted for the morning sessions and for the evening, not having eaten 4 h before. Again, we took a pragmatic approach to diet as abstinence of food in the morning has shown no effect on performance compared to a breakfast (Bougard et al. Citation2009), however a more stringent control of diet where the effects of the timing (relative to the session), amount (energy yield), composition (carbohydrate, protein, and fat) of food consumed during a study may be a confounding factor to performance (Bruggisser et al. Citation2023). The measurement site for core body temperature in the research was generally intra-aural 4/9 or oral 1/9, these sites are subject to masking effects specifically in the morning and can confound any daily variation (Waterhouse et al. Citation2005a). Three studies did not report core temperature but relied on this as the main mechanism to describe diurnal variation in time-trial performance in their discussion. Time of day scheduling was generally from 06:30–10:00 h and 17:30–20:30 h with laboratory temperature, humidity and barometric pressure only recorded in five studies. The error in measurement of distance for the ergometers chosen for the time-trial were not reported. Time of year – hence exposure to daylight in the morning, coming into the laboratory and in the evening was poorly reported (1/9 studies respectively, see Spitschan et al. Citation2019).
Table 4. Comparison of current study and other authors to the check list for consideration for diurnal variation (DV) studies (table 1), only studies whose participants were male, used a time-trial where distance was set and were undertaken in a thermoneutral environment were included in this comparison. FT = finishing time, PO = power output and CT = core temperature. * indicates contacted authors for information.
Lastly, Familiarisation was either not done (5/9), or 1 session (3/9) only completed, except for 3 sessions for Edwards et al. (Citation2008). Zadow et al. (Citation2016) in highly trained cyclists of a similar O2 max (67 ± 8.7 mL.kg−1.min−1) reported that only one session was needed to fully familiarise their population to a 4 km TT, we have shown similar findings in recreational cyclists completing a 16.1 km cycling TT (
O2 max 49.2 ± 9.4 mL.kg−1.min−1, Edwards et al. Citation2005). The effect of familiarisation of the TT performance and the number of sessions/practice trials needed to make subsequent learning effects negligible is unclear even if the order of administration of the tests is counterbalanced using a cross over repeated measures design. If you measure it, you can quantify the random error and systematic bias of your population doing your specific protocol. In our case, between the third and second familiarisation we had little measurement error and systematic bias (Atkinson et al. Citation1998; Atkinson and Nevill Citation1998; CV=2.71 %, t25 = −0.52, p=0.608), which was not the case between the third and first familiarisation and second and first respectively (CV=9.57 %, t25 = −2.39, p=0.024 and CV=10.40 %, t25 = −2.017, p=0.054). The presentation of such data would aid in comparing our findings to others who used only one familiarisation in moderately to highly trained athletes.
It has been previously suggested that diurnal variations related to exercise are generally larger in amplitude in physically fit than sedentary individuals (Atkinson and Reilly Citation1996). However, O2 max is a stable function regardless of time of day (Drust et al. Citation2005) and highly trained cyclists are used to training at different times of day and at intensities close to 100%
O2 max. Hence time of day effects in TT performance may be smaller in highly trained than untrained individuals. This agrees with the literature, where for faster TT performance such as the more aerobically fitter cyclists in Zadow et al. (Citation2016) population (
O2 max = 62.1 ± 8.7 mL.kg−1.min−1), perhaps by virtue of past repetition of TTs, had no difference in morning and evening times. This may be because they paced themselves more closely (a stable pacing profile) thereby produced similar power outputs irrespective of time of day. Whereas in lest trained populations such as ours and others with lower
O2 max values this pacing strategy or the ability to produce power may be more affected by time of day (48.5 to 58 mL.kg−1.min−1; Atkinson et al. Citation2005; Fernandes et al. Citation2014; Souissi et al. Citation2021). To address this, we investigated the associations between the change in M/E finishing time and average finishing time, as we had a range in 4-km completion of 320–455 s in a large sample size (range in
O2 max of 35–62 mL.kg−1.min−1 and relative Wingate peak power 10.77–16.11 W/kg). Linear regression analysis showed no correlation between change in finishing times between morning and evening (%) and average finishing time (p = 0.771), such that the fastest finishers did not have either greater or lower diurnal variation than the slower ones. It was thought that as the 4 km TT is completed in ~5 min and the anaerobic and aerobic component to performance in this current population is unknown; the Wingate (anaerobic capacity) and
O2 peak (maximum aerobic ability) values for participants may predict a relationship with diurnal variation variability whether positive or negative. There was no association between Peak power in the Wingate test (W/kg) and diurnal variation (p = 0.463), nor significant positive correlation between DV in finishing time and
O2 peak (r = 0.131, p = 0.069). Although the highest values of
O2 peak in our population are still lower than those of Zadow et al. (Citation2016) population, hence there may be “threshold” where daily variation in TT becomes minimal. Alternatively, it may be more to do with habitual time of day of training with a temporal specificity (or “activity” for non-highly trained cyclists) rather than a threshold (Souissi et al. Citation2002). To explore this, future studies would have to recruit a large cohort of recreational up to elite performers (hence explore the full performer range).
The findings of diurnal variation in performance in the current study are underpinned by findings of key variables such as sleep, where there was no difference in any subjective sleep questionnaires answered in both conditions at 07:00 h, implying no difference between the protocol and normal habits and no difference between sleep taken the night before conditions. Like others, we reported lower ratings of the mood state fatigue and higher rating of vigour values in the evening compared to the morning condition (Hill and Smith Citation1991; Brotherton et al. Citation2019). Importantly, the diurnal variation in resting Tr and Tsk temperature values (0.48 and 0.26 °C), which has been well established by previous research was also observed (Atkinson and Reilly Citation1996; Edwards et al. Citation2002; Waterhouse et al. Citation2005a).
While elite cyclists’ speed progressively declines during 4-km TTs (Corbett Citation2009), participants in this study adopted an inverted U or reversed J-shaped pacing strategy (see ), with a marked increase in PO towards the end of the TT indicative of a sprint finish (Abbiss and Laursen Citation2008). It is thought that a diurnal difference in pacing strategy of endurance performance may be due to altered feedback mechanisms from factors previously covered (such as body clock input, core body and muscle temperature etc) and through perception of effort being easier for any given workload in the evening than the morning (Reilly and Garrett Citation1995). In our case the adopted pacing strategy was the same regardless of time of day (p=0.856) in agreement with others (Fernandes et al. Citation2014; Zadow et al. Citation2020), only in the current study the participants put in ~9 W higher power output in the evening. Further, participants were not able to detect morning-evening or distance differences in how well they had subjectively paced themselves to finish as quickly as possible over the first 2-km and the last 2-km. Heart rate and ratings of perceived exertion progressively increased over the 4-km with pulse oximetry decreasing by ~1.9 % and all measures of SmO2 (n=17) for forearm, thigh and calf decreasing by 6.9, 6.2 and 6.9 % (see ). The decrease in SmO2 was expected as the thigh and calf are the main muscles to produce force during cycling (Takagi et al. Citation2013). The drop in SmO2 levels of the forearm was unexpected but can likely be attributed to participants’ tight grip of the cycle ergometers handlebars or blood flow redistribution towards active muscles.
Limitations
Participants in this study were not elite, rather recreational cyclists and although we familiarised them 3 times, some had limited experience with cycling 4-km TTs. Future research should consider testing with a cohort of recreational up to elite performers to see whether the outcomes would be similar.
Conclusions
In a methodologically rigorous study where a (i) standardised approach has been employed, (ii) participants did not have time of day preference to exercise and (iii) were intermediate types, a diurnal variation was found in rectal temperature of 0.48 °C and 4-km TT performance was faster in the evening than the morning by 2.6 %. Discrepancies in findings of a time-of-day difference in time-trial performance between research could be related to fitness and level of athleticism of the population rather than methodological differences.
Highlights
Time-of-day effects on time-trial performance is not consistently reported, this lack of agreement may be explained by a lack of rigor and standardisation in method employed, population chosen and a lack of control. We propose a practical tick list for consideration to “standardise” research investigating diurnal variation in performance.
Resultant finishing times after a standardised protocol were faster in the evening by 2.6 % than the morning. Discrepancies in past findings of a daily difference in time-trial performance could be related to fitness and level of athleticism of the population rather than methodological differences.
Further work exploring the criteria for recommendations (standardisation) is suggested for time-of-day studies, a practical approach that gives a “strict”, “moderate” and “recommended” classification would be useful.
Acknowledgements
We would like to thank the participants who participated in the study, also the research assistants who helped with data collection Mr Adrian Markov, Miss Theresa Toussaint, Mr Tom Hall-Say, Mr Samuel Dickson, Mr Dylan Gill, Mr Kyle McCann and Mr Greg Stewart-Taylor.
Disclosure statement
No potential conflict of interest was reported by the author(s).
References
- Abbiss C, Laursen P. 2008. Describing and understanding pacing strategies during athletic competition. Sports Med. (Auckland, N.Z.). 38:239–252. doi: 10.2165/00007256-200838030-00004.
- Abt G, Boreham C, Davison G, Jackson R, Nevill A, Wallace E, Williams M. 2020. Power, precision, and sample size estimation in sport and exercise science research. J Sports Sci. 38(17):1933–1935. doi: 10.1080/02640414.2020.1776002.
- Araujo LG, Waterhouse J, Edwards B, Rosa Santos EH, Tufik S, de Mello MT. 2011. Twenty-four-hour rhythms of muscle strength with a consideration of some methodological problems. Biol Rhythm Res. 42(6):473–490. doi: 10.1080/09291016.2010.537444.
- Atkinson G, Nevill AM. 1998. Statistical methods for assessing measurement error (reliability) in variables relevant to sports medicine. Sports Med. 26:217–238. doi: 10.2165/00007256-199826040-00002.
- Atkinson G, Nevill AM, Edwards BJ. 1998. What is an acceptable amount of measurement error? The application of meaningful “analytical goals” to the reliability assessment of sports science measurements made on a ration scale. [Abstract] J Sports Sci. 17:18.
- Atkinson G, Reilly T. 1996. Circadian variations in sporting performance. Sports Med. 21(4):292–312. doi: 10.2165/00007256-199621040-00005.
- Atkinson G, Todd C, Reilly T, Waterhouse J. 2005. Diurnal variation in cycling performance: influence of warm-up. J Sports Sci. 23:321–329. PMID: 15966350. doi: 10.1080/02640410410001729919.
- Ayalon A, Inbar O, Bar-Or O. 1974. Relationships among measurements of explosive strength and anaerobic power. In: Nelson R Morehouse C, editors. Biomechanics IV. London: International Series on Sport Sciences. Palgrave; p. 572–577. doi: 10.1007/978-1-349-02612-8_85.
- Bakkevig MK, Nielsen R. 1994. Impact of wet underwear on thermoregulatory responses and thermal comfort in the cold. Ergonomics. 37(8):1375–1389. PMID: 7925261. doi: 10.1080/00140139408963681.
- Barton J, Folkard S, Smith LR, Spelten ER, Totterdell PA 1990. Standard shiftwork index manual. In: SAPU memo No: 1159 MRC/ESRC social and applied psychology unit. Sheffield: Department of Psychology, University of Sheffield; p. S10 2TN.
- Batterham AM, Atkinson G. 2005. How big does my sample need to be? A primer on the murky world of sample size estimation. Phys Ther Sport. 6(3):153–163. doi: 10.1016/j.ptsp.2005.05.004.
- Batterham AM, Hopkins WG. 2006. Making meaningful inferences about magnitudes. Int J Sports Physiol Perform. 1(1):50–57. PMID:19114737. doi: 10.1123/ijspp.1.1.50.
- Baxter C, Reilly T. 1983. Influence of time of day on all-out swimming. Br J Sports Med. 17:122–127. doi: 10.1136/bjsm.17.2.122.
- Bommasamudram T, Ravindrakumar A, Varamenti E, Tod D, Edwards BJ, Irene G, Peter IG. 2022. Daily variation on performance measures related to time-trials: a systematic review. Chronobiol Int. 39(9):1167–1182. Epub 2022 Jul 11. PMID: 35815685. doi: 10.1080/07420528.2022.2090373.
- Borg G. 1973. Perceived exertion: a note on history and methods. Med Sci Sporta Exe. 5(2):90–93. PMID: 4721012. doi: 10.1249/00005768-197300520-00017.
- Bougard C, Bessot N, Moussay S, Sesboue B, Gauthier A. 2009. Effects of waking time and breakfast intake prior to evaluation of physical performance in the early morning. Chronobiol Int. 26(2):307–323. PMID: 19212843. doi: 10.1080/07420520902774532.
- Boukelia B, Fogarty MC, Davison RC, Florida-James GD. 2017. Diurnal physiological and immunological responses to a 10-km run in highly trained athletes in an environmentally controlled condition of 6 °C. Eur J Appl Physiol. 117(1):1–6. doi: 10.1007/s00421-016-3489-5.
- Boukelia B, Gomes E, Florida-James G. 2018. Diurnal variation in physiological and immune responses to endurance sport in highly trained runners in a hot and humid environment. Oxid Med Cell Longev. 2:1–9. doi: 10.1155/2018/3402143.
- Boyett JC, Giersch GE, Womack CJ, Saunders MJ, Hughey CA, Daley HM, Luden ND. 2016. Time of day and training status both impact the efficacy of caffeine for short duration cycling performance. Nutrients. PMID: 27754419. 8:639. doi: 10.3390/nu8100639.
- Brotherton EJ, Moseley SE, Langan-Evans C, Pullinger SA, Robertson CM, Burniston JG, Edwards BJ. 2019. Effects of two nights partial sleep deprivation on an evening submaximal weightlifting performance; are 1 h powernaps useful on the day of competition? Chronobiol Int. 36(3):407–426. doi: 10.1080/07420528.2018.1552702.
- Bruggisser F, Knaier R, Roth R, Wang W, Qian J, FAJL S. 2023. Best time of day for strength and endurance training to improve health and performance? A systematic review with meta-analysis. Sports Med Open. 19(1):34. PMID: 37208462; PMCID: PMC10198889. doi: 10.1186/s40798-023-00577-5.
- Burton AC. 1935. Human calorimetry: the average temperature of the tissues of the body. J Nutr. 9(3):261–280. doi: 10.1093/jn/9.3.261.
- Conroy RTWL, O’Brien M 1973. Proceedings: diurnal variation in athletic performance. J Physiol 236(1):51. PMID: 4818520.
- Corbett J. 2009. An analysis of the pacing strategies adopted by elite athletes during track cycling. Int J Sports Physiol Perform. 4:195–205. PMID: 19567923. doi: 10.1123/ijspp.4.2.195.
- Dalton B, McNaughton L, Davoren B. 1997. Circadian rhythms have no effect on cycling performance. Int J Sports Med. 18(7):538–542. PMID: 9414078. doi: 10.1055/s-2007-972678.
- Drust B, Waterhouse J, Atkinson G, Edwards B, Reilly T. 2005. Circadian rhythms in sports performance—an update. Chronobiol Int. 22(1):21–44. doi: 10.1081/CBI-200041039.
- Edwards BJ, Boyle B, Doran D, Waterhouse J, Reilly T. 2008. Effect of time of day on 10-km time-trial running performance. abstract for the E.C.S.S. 13th annual congress. 9-12 July, 2008. Estoril, Portugal.
- Edwards BJ, Edwards W, Atkinson G, Waterhouse J, Reilly T. 2005. Can performance in a morning time-trial be improved by morning exercise the day before? Int J Sports Med. 26(8):651–656. PMID: 16158370. doi: 10.1055/s-2004-830439.
- Edwards BJ, Gallagher C, Pullinger SA, de Mello MT, Walsh NP. 2023. Athletic performance; effects of sleep loss. In: editorKushida CA. Encyclopedia of sleep and circadian rhythms Second. Netherlands: Academic Press: pp. 434–443. ISBN 9780323910941
- Edwards BJ, Pullinger SA, Kerry JW, Robinson WR, Reilly TP, Robertson CM, Waterhouse JM. 2013. Does raising morning rectal temperature to evening levels offset the diurnal variation in muscle force production? Chronobiol Int. 30(4):486–501. PMID: 23281719. doi: 10.3109/07420528.2012.741174.
- Edwards B, Waterhouse J, Reilly T, Atkinson G. 2002. A comparison of the suitability of rectal, gut and insulated axilla temperatures for measurement of the circadian rhythm of core temperature in field studies. Chronobiol Int. 19(3):579–597. PMID: 12069039. doi: 10.1081/cbi-120004227.
- Faul F, Erdfelder E, Lang AG, Buchner A. 2007. G*power 3: a flexible statistical power analysis program for the social, behavioral, and biomedical sciences. Behav Res Methods. 39(2):175–191. doi: 10.3758/BF03193146.
- Fernandes AL, Lopes-Silva JP, Bertuzzi R, Casarini DE, Arita DY, Bishop DJ, Lima-Silva AE, Shen W. 2014. Effect of time of day on performance, hormonal and metabolic response during a 1000-M cycling time trial. PloS One. 9(10):e109954. doi: 10.1371/journal.pone.0109954.
- Fiedler J, Altmann S, Chtourou H, Engel F, Neumann R, Woll A. 2022. Daytime fluctuations of endurance performance in young soccer players: a randomized cross-over trial. BMC Res Notes. 15:351. doi:10.1186/s13104-022-06247-1.
- Gough LA, Deb SK, Sparks A, McNaughton LR. 2017. The reproducibility of 4-km time trial (TT) performance following individualised sodium bicarbonate supplementation: a randomised controlled trial in trained cyclists. Sport Med Open. 3(1):34. doi: 10.1186/s40798-017-0101-4.
- Hale T, Armstrong N, Hardman A, Jakeman P, Sharp C, W. 1988. Position statement on the physiological assessment of the elite competitor. 2nd ed. Leeds: British Association of Sport and Exercise Sciences; p. 4.1.
- Hill DW, Smith JC. 1991. Effect of time of day on the relationship between mood state, anaerobic power, and capacity. Percept Mot Skills. 72(1):83–87. PMID: 2038539. doi: 10.2466/pms.72.1.83.
- Jones S, Chiesa ST, Chaturvedi N, Hughes AD. 2016. Recent developments in near-infrared spectroscopy (NIRS) for the assessment of local skeletal muscle microvascular function and capacity to utilise oxygen. Artery Res. 16:25–33. doi: 10.1016/j.artres.2016.09.001.
- Knaier R, Qian J, Roth R, Infanger D, Notter T, Wang W, Cajochen C, Scheer FAJL. 2022. Diurnal variation in maximum endurance and maximum strength performance: a systematic review and meta-analysis. Med Sci Sports Exerc. 54(1):169–180. doi: 10.1249/MSS.0000000000002773.
- Landrum RE. 1992. College students’ use of caffeine and its relationship to personality. Coll Stud J. 26:151–155.
- Lok R, Zerbini G, Gordijn MCM, Beersma DGM, Hut RA. 2020. Gold, silver or bronze: circadian variation strongly affects performance in Olympic athletes. Sci Rep. 10(1):16088. PMID: 33033271; PMCID: PMC7544825. doi: 10.1038/s41598-020-72573-8.
- Martin L, Nevill AM, Thompson KG. 2007. Diurnal variation in swim performance remains, irrespective of training once or twice daily. Int J Sports Physiol Perform. 2:192–200. PMID: 19124906. doi: 10.1123/ijspp.2.2.192.
- McKay AK, Stellingwerff T, Smith ES, Martin DT, Mujika I, Goosey-Tolfrey VL, Sheppard J, Burke LM. 2021. Defining training and performance calibre: a participant classification framework. Int J Sport Physiol. 17(2):317–331. doi: 10.1123/ijspp.2021-0451.
- McNair DM, Lorr M. 1971. Profile of mood states manual. San Diego (CA): Educational and Industrial testing services.
- Monk TH, Leng VC. 1982. Time of day effects in simple repetitive tasks: some possible mechanisms. Acta Psychol. 51(3):207–221. doi: 10.1016/0001-6918(82)90035-X.
- Pullinger SA, Oakley AJ, Hobbs R, Hughes M, Robertson CM, Burniston JG, Edwards BJ. 2019. Effects of an active warm-up on variation in bench press and back squat (upper and lower body measures). Chronobiol Int. 36(3):392–406. ID:1552702. doi: 10.1080/07420528.2018.1552596.
- Rae DE, Stephenson KJ, Roden LC. 2015. Factors to consider when assessing diurnal variation in sports performance: the influence of chronotype and habitual training time-of-day. Eur J Appl Physiol. 115:1339–1349.
- Ramanathan NL. 1964. A new weighting system for mean surface temperature of the human body. J Appl Physiol. 19:531–533. PMID: 14173555. doi: 10.1152/jappl.1964.19.3.531.
- Ravindrakumar A, Bommasamudram T, Tod D, Edwards BJ, Chtourou H, Pullinger SA. 2022. Daily variation on performance measures related to anaerobic power and capacity: a systematic review. Chronobiol Int. 39(3):421–455. PMID: 34978950. doi: 10.1080/07420528.2021.1994585.
- Reilly T, Atkinson G, Waterhouse J 2000. Chronobiology and physical performance. In: Garrett W Kirkendall D, editors. Exercise and sport science. Vol. 24, Philadelphia (PA): Lipcott Williams and Wilkins; pp. 351–372.
- Reilly T, Garrett R. 1995. Effects of time of day on self-paced performances of prolonged exercise. J Sports Med Phys Fitness. 35(2): 99–102. PMID: 7500634.
- Silveira A, Alves F, Teixeira AM, Rama L. 2020. Chronobiological effects on mountain biking performance. Int J Environ Res Public Health. PMID: 32899823; PMCID: PMC7558596. 17:6458. doi: 10.3390/ijerph17186458.
- Souissi N, Gauthier A, Sesboüé B, Larue J, Davenne D. 2002. Effects of regular training at the same time of day on diurnal fluctuations in muscular performance. J Sports Sci. 20:929–937. PMID: 12430993. doi: 10.1080/026404102320761813.
- Souissi W, Hammouda O, Ammar A, Ayachi M, Bardiaa Y, Daoud O, Hassen IB, Souissi M, Driss T. 2021. Higher evening metabolic responses contribute to diurnal variation of self-paced cycling performance. bs. 39(1):3–9. 102930. PMID: 35173357; PMCID: PMC8805356. doi: 10.5114/biolsport.2021.102930.
- Spitschan M, Stefani O, Blattner P, Gronfier C, Lockley SW, Lucas RJ. 2019. How to report light exposure in human chronobiology and sleep research experiments. Clocks Sleep. 1(3):280–289. doi: 10.3390/clockssleep1030024.
- Takagi S, Kime R, Niwayama M, Murase N, Katsumura T. 2013. Muscle oxygen saturation heterogeneity among leg muscles during ramp exercise. Adv Exp Med Biol. PMID: 22879044. 765:273–278. doi: 10.1007/978-1-4614-4989-8_38.
- Walsh NP, Halson SL, Sargent C, Roach GD, Nédélec M, Gupta L, Leeder J, Fullagar HH, Coutts AJ, Edwards BJ, et al. 2021. Sleep and the athlete: consensus statement. Br J Sports Med. 55(7):356–368. doi: 10.1136/bjsports-2020-102025.
- Waterhouse J, Drust B, Weinert D, Edwards B, Gregson W, Atkinson G, Kao S, Aizawa S, Reilly T. 2005a. The circadian rhythm of core temperature: origin and some implications for exercise performance. Chronobiol Int. 22(2):207–226. PMID: 16021839. doi: 10.1081/cbi-200053477.
- Waterhouse J, Nevill A, Finnegan J, Williams P, Edwards B, Kao S, Reilly T. 2005. Further assessments of the relationship between jet lag and its symptoms. Chronobiol Int. 22(1):107–122. doi: 10.1081/CBI-200036909.
- Winget CM, DeRoshia CW, Holley DC. 1985. Circadian rhythms and athletic performance. Med Sci Sports Exerc. 17(5):498–516. PMID: 3906341. doi: 10.1249/00005768-198510000-00002.
- Winter EM, Jones AM, Davison RC, Bromley PD, Mercer TH. 2007. Sport and exercise physiology testing guidelines. In: The British association of sport and exercise sciences guide. Vol. 1. Abington: Routledge.
- Youngstedt SD, O’Connor PJ. 1999. The influence of air travel on athletic performance. Sports Med. 28(3):197–207. PMID: 10541442. doi: 10.2165/00007256-199928030-00004.
- Yousefzadehfard Y, Wechsler B, DeLorenzo C. 2022. Human circadian rhythm studies: practical guidelines for inclusion/exclusion criteria and protocol. Neurobiol Sleep Circadian Rhythms. 13:1–18. ISSN 2451-9944. doi: 10.1016/j.nbscr.2022.100080.
- Zadow E, Fell J, Kitic C. 2016. The reliability of a laboratory-based 4 km cycle time trial on a wahoo KICKR power trainer. Journal Of Science And Cycling. 5:23–27. https://jscjournal.com/index.php/JSC/article/view/241
- Zadow EK, Fell JW, Kitic CM, Han J, Wu SSX. 2020. Effects of time of day on pacing in a 4-km time trial in trained cyclists. Int J Sports Physiol Perform. 15:1455–1459. PMID: 33017804. doi: 10.1123/ijspp.2019-0952.