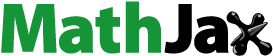
ABSTRACT
People often simultaneously expose to acceptable/tolerable daily intake of food additives and pesticides in daily life. Exposure in adult rodents to acceptable/tolerable daily intake of food additives and pesticides chemical mixtures (AFACM) is reported to cause health damage. Our recently study reported that AFACM exposure of pregnant mice resulted in neuroinflammation and abnormal emotional behaviours in offspring. Here, we found that neutralising IFN-γ with neutralising antibodies completely blocked the influences induced by maternal AFACM exposure, including the increased IFN-γ level in serum, increased IFN-γ, interleukin-6 and tumour necrosis factor-α levels in prefrontal cortex(PFC) in offspring on postnatal day(PND)7, the abnormal emotional behaviours in offspring on PND56, and the decreased of ATPase phospholipid flippase 8A2(ATP8A2) in the PFC of offspring on PND7. In sum, maternal AFACM exposure induced the PFC neuroinflammation, abnormal emotional behaviours and decreased PFC ATP8A2 expression through increasing systemic IFN-γ in offspring.
Abbreviations
AFACM | = | Acceptable/Tolerable daily intake of food additive and pesticide chemical mixtures |
ADI | = | Acceptable daily intake |
ATP8A2 | = | ATPase phospholipid flippase 8A2 |
ED | = | Embryonic day |
GD | = | Gestational day |
IFN-γ | = | Interferon-γ |
IL-6 | = | Interleukin-6 |
IL-12p70 | = | Interleukin-12p70 |
MCP-1 | = | Monocyte chemoattractant protein-1 |
NP-40 | = | Nonidet P 40 |
PFC | = | Prefrontal cortex |
PMSF | = | Phenylmethylsulfonyl fluoride |
PND | = | Postnatal day |
SDS | = | Sodium dodecyl sulfate |
SDS-PAGE | = | Sodium dodecyl sulfate polyacrylamide gel electrophoresis |
TDI | = | Tolerable daily intake |
TNF-α: | = | Tumour necrosis factor-α |
1. Introduction
People nowadays expose orally to various food additives and residues of pesticides via their daily diet (Docea et al., Citation2018). Although the dose of each of these chemicals in daily diet is under its acceptable daily intake(ADI) or tolerable daily intake(TDI) (Pisanello, Citation2014), simultaneous exposure to a variety of chemicals has been verified to cause health damage (Docea et al., Citation2018; Docea et al., Citation2019; Tsatsakis et al., Citation2019; Tsatsakis et al., Citation2017). Recently, Docea AO, et al. and Tsatsakis AM, et al. reported that exposure to safe doses of 13 mixtures of food additives and pesticides (carbaryl; calcium disodium ethylene diamine tetra-acetate; butylparaben; bisphenol A; acacia gum; dimethoate; glyphosate; triadimefon; aspartame; sodium benzoate; methomyl; methyl parathion; ethylparaben) lead to an increase in feed and water consumption, biliary obstruction, liver function damage and a significant increase in serum levels of pro-inflammatory cytokines such as tumour necrosis factor(TNF)-α and interferon(IFN)-γ in rats (Docea et al., Citation2018; Docea et al., Citation2019; Tsatsakis et al., Citation2019). AFACM exposure has also been suggested to induce adverse effects on the behaviour and central nervous system function such as abnormal emotional behaviours in rats (Tsatsakis et al., Citation2019).
Embryonic period is a vital developmental stage in which individuals are sensitive to environmental factors and maternal exposure to various environmental factors during pregnancy can increase the risk of health damage of offspring. Maternal diet during pregnancy is related to many abnormal emotional behaviours of offspring, which can induce anxiety, depression, hyperactivity and autism spectrum syndrome (Sullivan et al., Citation2012). Maternal immune activation has an irreversible effect on the developing brain of offspring (Madore et al., Citation2016), and immune activation is related to emotional abnormalities (Palazidou, Citation2012). In daily life, exposure to mixed chemicals is a high-frequency event for pregnant women (Li et al., Citation2019; Woodruff et al., Citation2011). We have recently reported that maternal exposure by liberally drinking water containing dissolved AFACM resulted in neuroinflammation and abnormal emotional behaviours in offspring mice (Song et al., Citation2021), but the mechanism underlying these effects is still not clear.
IFN-γ is one of the cytokines involved in abnormal emotional behaviours in humans and rodents (Himmerich et al., Citation2010; Lichtblau et al., Citation2013; O’Connor et al., Citation2009). Our previous study has found that IFN-γ was the only serum cytokine showing an altered level in the offspring born to the AFACM-exposed dams and that there was a significant positive correlation between serum IFN-γ level and the level of neuroinflammation in the brain (Song et al., Citation2021). Therefore, it is suggested that the increase of serum IFN-γ in offspring may be the key mediator of inflammation in the PFC and abnormal emotional behaviours caused by maternal AFACM exposure. In order to verify this scientific conjecture, neutralising anti-IFN-γ antibody was used to determine whether the effects of maternal AFACM exposure on the health status of offspring could be blocked.
In addition, our recent study reported that exposure to AFACM during pregnancy increased the levels of IFN-γ in serum and PFC in offspring (Song et al., Citation2021), and the expression of ATP8A2 can be decreased by increasing the level of IFN-γ (Deng et al., Citation2021; Shulzhenko et al., Citation2018). ATP8A2 is a key protein to maintain the stability of phospholipid components in neuronal cell membrane and plays a vital role in maintaining the normal function of cells (Andersen et al., Citation2016; Choi et al., Citation2019; Coleman et al., Citation2009). It is thereby essential for the normal neural development and function of the brain (Coleman & Molday, Citation2011; Xu et al., Citation2012). The down-regulation and functional inhibition of ATP8A2 are considered to be closely related to brain developmental abnormalities and dysfunction (Choi et al., Citation2019). Therefore, the current study also examined whether AFACM exposure during pregnancy affected the expression of ATP8A2 in PFC through the increase of IFN-γ.
2. Materials and methods
2.1. Animals
8-week-old C57BL/6 mice were purchased from Guangdong Medical Laboratory Animal Center. Animals were kept in a room with a 12 h light/dark cycle (08:00–20:00/20:00–08:00) with food and water available ad libitum. After adaptive feeding for one week, one male mouse and two female mice were raised in the same cage. Female mice were examined for vaginal suppository at 8:00 and 19:00 every day. The day when the vaginal suppository was found was defined as GD(gestational day)0. A total of 12 pregnant mice were prepared and randomly divided into two groups (6 per group) in the experiment shown in (a–e) and the experiment shown in Figure S1. A total of 30 pregnant mice were prepared and randomly divided into five groups (6 per group) in the experiment shown in , the experiment shown in , the experiment shown in and the experiment shown in Figure S2. A total of 18 pregnant mice were prepared and randomly divided into two groups (6 per group) in the experiment shown in . Pregnant mice were given free access to normal drinking water or AFACM dissolved in drinking water. Maternal AFACM exposure lasted from GD0 of pregnant mice to the time of sampling or delivery. In experiment-1, the pregnant mice were euthanized on GD18 when they had not given birth (See and detailed design in the sheet named Experiment-1 in Supplementary Tables file). In experiment-2, the pregnant mice were euthanized on GD12.5 when they had not given birth (See Figure S1 and detailed design in the sheet named Experiment-2 in Supplementary Tables file). In experiment-3, the pregnant mice were euthanized on the day, immediately after they had given birth (See Figure S2 and detailed design in the sheet named Experiment-3 in Supplementary Tables file). In experiment-4, the pregnant mice were euthanized on GD15.5 when they had not given birth (See detailed design in the sheet named Experiment-4 in Supplementary Tables file). In experiment-5, pregnant mice were not used for tests and they were euthanized on the weaning day (postnatal day 21) (See and detailed design in the sheet named Experiment-5 in Supplementary Tables file). In experiment-6, pregnant mice were not used for tests and they were euthanized on postnatal day 7 when their pups were used for WB or IF tests (See and and detailed design in the sheet named Experiment-6 in Supplementary Tables file).
Figure 1. Maternal serum levels of cytokines on GD18 and fetal levels of cytokines in serum and PFC on ED18. (a) The levels of IFN-γ in maternal serum. (b) The levels of IL-6 in maternal serum. (c) The levels of IL-12p70 in maternal serum. (d) The levels of TNF-α in maternal serum. (e) The levels of MCP-1 in maternal serum. (f) The levels of IFN-γ of fetal mice in serum. (g) The levels of TNF-α of fetal mice in serum. (h) The levels of IFN-γ of fetal mice in PFC. (i) The levels of IL-6 of fetal mice in PFC. (j) The level of MCP-1 of fetal mice in PFC. Data is expressed as mean ± standard deviation. Student's t test, n = 6, *P < 0.05, **P < 0.01. CON, control; AFACM, acceptable/tolerable daily intake of food additives and pesticides chemical mixtures.

Figure 2. Cytokine levels in serum and PFC of offspring on PND7. (a) Levels of IFN-γ in serum of offspring on PND7. (b) Levels of IFN-γ in the PFC of PND7 offspring. (c) Levels of IL-6 in the PFC of PND7 offspring. (d) Levels of TNF-α in the PFC of PND7 offspring. Data is expressed as mean ± standard deviation. One-way ANOVA, n = 6, *P < 0.05, **P < 0.01, ***P < 0.001. CON, control; AFACM, acceptable/tolerable daily intake of food additives and pesticides chemical mixtures.

Figure 3. Cytokine levels in serum and PFC of offspring on PND14. (a) Levels of IFN-γ in serum of offspring on PND14. (b) Levels of IFN-γ in the PFC of offspring on PND14. (c) Levels of IL-6 in the PFC of offspring on PND14. (d) Levels of TNF-α in the PFC of offspring on PND14. Data is expressed as mean ± standard deviation. One-way ANOVA, n = 6. CON, control; AFACM, acceptable/tolerable daily intake of food additives and pesticides chemical mixtures.

Figure 4. Results of behavioural tests in offspring. (a) Interaction time with unfamiliar mice in three-box social trial. (b) Interaction time with novel mice in three-box social trial. (c) Time to enter the central zone in the open field test. (d) The number of accesses to the central zone in the open field test. (e) Time to float in the forced swim test. (f) The time of hanging immobile in the tail suspension test. Data is expressed as mean ± standard deviation. Two-way ANOVA, n = 12/treatment, *P < 0.05, **P < 0.01, ***P < 0.001. CON, control; AFACM, acceptable/tolerable daily intake of food additives and pesticides chemical mixtures; M, male; F, female.

Figure 5. The expression level of ATP8A2 in the PFC of offspring was detected by Western Blot. (a) Statistical analysis results of Western Blot. Data is expressed as mean ± standard deviation. One-way ANOVA, n = 6, **P < 0.01, ***P < 0.001. (b) Expression level of ATP8A2 in PFC of offspring. CON, control; AFACM, acceptable/tolerable daily intake of food additives and pesticides chemical mixtures.

Figure 6. Immunofluorescence analyses indicated that IFN-γ mediated the decreased PFC ATP8A2 expression caused by maternal AFACM exposure. (a–i) Representative immunofluorescence staining for ATP8A2 (red)/NeuN (green) co-labelling in each group. (j) Representative high magnificent photos show ATP8A2/NeuN co-labeling in the PFC. Arrows indicates ATP8A2/NeuN co-labeling cells. (k) Statistical analysis results of the mean fluorescence intensity of ATP8A2 expression in different groups. (l) The red frame shows the area in the mouse brain atlas where is the area for observing the expression of ATP8A2. Data is expressed as mean ± standard deviation. One-way ANOVA, n = 6, **P < 0.01, ***P < 0.001. (A-I) Scale bar is 100 μm. (J) Scale bar is 20 μm. CON, control; AFACM, acceptable/tolerable daily intake of food additives and pesticides chemical mixtures.

This study was performed in strict accordance with the U.K. Animals (Scientific Procedures) Act, 1986 and was approved by the Institutional Animal Ethics Committee of Guangdong Pharmaceutical University (gdpulacSPF2017357).
2.2. AFACM preparation
The pregnant mice were exposed by drinking water to a mixture containing the following chemicals with the corresponding ADI/TDI (mg/kg bw/day): carbaryl (0.0075) (Cat. No.: R014224); calcium disodium ethylene diamine tetra-acetate (2.5) (Cat. No.: R003463); butylparaben (0.5) (Cat. No.: R005013); bisphenol A (0.004) (Cat. No.: R005000) and acacia gum (34) (Cat. No.: R003199) were purchased from Yien Chemical Technology Co., Ltd. (Shanghai, China). Dimethoate (0.001) (Cat. No.: D109819); glyphosate (0.5) (Cat. No.: P109919); triadimefon (0.03) (Cat. No.: T109946); aspartame (40) (Cat. No.: A113247) and sodium benzoate (5) (Cat. No.: S104124) were purchased from Aladdin Biochemical Technology Co., Ltd. (Shanghai, China). Methomyl (0.0025) (Cat. No.: CCPD100581) and methyl parathion (0.003) (Cat. No.: CCPD100646) were purchased from CATO Research Chemicals Inc. (Guangzhou, China). Ethylparaben (10) (Cat. No.: A-NH270) were purchased from Xianding Biotechnology Co., Ltd. (Shanghai, China). The dose of each of the 13 chemicals for oral exposure of mice was determined strictly according to the recently published literatures (Docea et al., Citation2018; Docea et al., Citation2019; Tsatsakis et al., Citation2019; Tsatsakis et al., Citation2017), which investigated and revealed the influences on health of AFACM exposure in non-pregnant rodents.
To be specific, a storage solution was prepared by dissolving each of the 13 chemical substances in 100 L of drinking water with the dose being 1000×ADI/TDI, that is, the dose of each of the 13 chemical substances in 100 mL stock solution is equivalent with the dose for 1 kg body weight/day which is consist to previous report. During the experiment, the body weight of pregnant mice on the day and the consumed volume of the AFACM-containing drinking water on the previous day were weighed and calculated at 8:00. These data were used in determining the volume of storage solution when preparing the AFACM-containing drinking water administrated to each pregnant mouse on the present day. The AFACM-containing drinking water was replaced every day at 8:30 with 20 mL fluid newly reconstituted according to the formulation shown here (take aspartame that has a dose per kg body weight/day at 40 mg for an example to illustrate the formulation):
In the formula, “BW” is the body weight of each pregnant mouse measured on the day, “V1” is the volume of storage fluid used for formulation on the day, “V2” is the volume of drinking water actually consumed on the previous day.
2.3. Administration of neutralising anti-IFN-γ mAb and isotype IgG1
Rat anti-IFN-γ neutralising mAb or rat-derived isotype IgG1 (Invitrogen, Waltham, USA) were intraperitoneally injected daily to mice from GD12.5 to PND0 according to the result of a preliminary experiment (see Section 3.1). The optimal dosage of neutralising anti-IFN-γ mAb is 0.1 mg/kg body weight according to the result of another preliminary experiment (see Section 3.2). The dosage of isotype IgG1 was also determined as 0.1 mg/kg body weight.
2.4. Determination of cytokines levels
The dams were over-anesthetized with 3% sodium pentobarbital intraperitoneally before the blood was collected from the right ventricle. Blood collected from each dam was put into a single centrifuge tube. The blood sample was allowed to stand at room temperature for 1 h. After centrifugation, the supernatant was taken and stored at −80°C for the following analysis. Immediately after blood collection, the uterus was dissected and the fetal mice were taken out of uterus. After observing and confirming the absence of pain response in each fetal mouse, decapitation was performed to collect blood. Blood collected from the six randomly selected fetal mice from the same pregnant litter was put into a same centrifuge tube. Unused mice were euthanized with an overdose of 3% sodium pentobarbital. Then the blood sample was allowed to stand at room temperature for 1 h. After centrifugation, the supernatant was taken and stored at −80°C for the following analysis. Immediately after blood collection, the PFC tissue of each fetal mouse was immediately isolated on ice. The PFC tissue collected from the same six randomly selected fetal mice described as above was put into a same centrifuge tube. Then the PFC tissue samples were homogenated with assay buffer (RIPA; 50 mM Tris (pH 7.4), 150 mM NaCl, 1% NP-40 (Nonidet P 40), 0.5% sodium deoxycholate, 0.1% SDS (Sodium dodecyl sulfate), protease inhibitor cocktail (Sigma), and 1 mM PMSF (Phenylmethylsulfonyl fluoride)) before the supernatant was taken and stored at −80°C for the following analysis.
Another cohort pregnant mice were prepared with the same procedure. On PND7, one pup from each litter was randomly selected and over-anesthetized. The blood was collected from the right ventricle and put into a centrifuge tube. Their serum samples were prepared the same way. Immediately after blood collection, the pup was performed with transcardiac perfusion with 0.9% NaCl. Then the PFC tissue was isolated and homogenated as above. On PND14, one pup from each litter was randomly selected and over-anesthetized. Their serum and the PFC tissue samples were obtained using the same method as on PND7. The rest pups in each litter continued to be raised and two of them (1 male and 1 female) were subjected to behavioural tests from PND56 (see Sections 2.5–2.8).
A mouse cytokine/chemokine magnetic bead panel (MCYTOMAG70 K; Millipore, Billerica, MA, USA) was employed to detect the levels of IFN-γ, TNF-α, interleukin(IL)−6, IL-12p70, monocyte chemoattractant protein(MCP)-1 both in the serum and PFC. The standard curve range for this kit is 3.2–10,000 pg/mL. Serum sample was diluted 1:2 in assay buffer. BCA protein detection kit (Bioworld, Atlanta, USA) was used to adjust the total protein concentration of each of the supernatant samples of PFC tissue homogenate to 4.5 mg/mL. The tests were conducted strictly according to the instructions for the multiplex assays. The data were collected on a Bio-Plex-200 system (Bio-Rad, Hercules, USA), then analysed using a professional software (Bio-Plex Manager). In the preliminary experiment described in Section 3.2, the concentrations of IFN-γ were detected with a commercial ELISA kit (Mlbio, Shanghai, China). In the preliminary experiment described in Section 3.2, the concentrations of anti-IFN-γ neutralising mAb were detected with another commercial ELISA kit (Elabscience, Wuhan, China).
2.5. Three-chambered social test
The testing procedure consists of three chronological phases: habituation, sociability, and social novelty. As reported previously (Tang et al., Citation2014), the mice were placed in the middle chamber with the entrances to both the side chambers open and they were allowed to freely explore all three chambers for 5 min. In the sociability phase, the test mouse was enclosed in the middle chamber, and a novel mouse was enclosed in a wire pencil cup placed in a side chamber. An empty wire pencil cup was placed the chamber on the opposite side to serve as a novel object control. Then, the entrances to both side chambers were reopened, and the test mouse was allowed to freely explore the entire apparatus for 10 min. The time each mouse spent sniffing the stranger mouse was calculated using the SuperMaze video analysis system (Shanghai Xinruan Information Technology Co., Ltd., China). In the social novelty phase, a second novel mouse was placed in the wire pencil cup that had been empty during the sociability test session. Each test mouse was further tested, and the time spent sniffing the novel mouse was calculated. After each trial, cages and chambers were cleaned with 70% ethanol (Wang et al., Citation2019).
2.6. Open field test
The open field test was performed 24 h after the end of the three-chambered social test. A single mouse was placed in a 50 cm × 50 cm × 50 cm apparatus and allowed to freely explore the apparatus for 10 min. The time each mouse spent in the central zone of the open field and the numbers of entries into the central zone were recorded using the TopScanTM 2.0 system (Clever Sys. Inc.). After each test, the test apparatus was cleaned with 70% ethanol (Wang et al., Citation2019).
2.7. Forced swimming test
Forced swimming test was performed 1 h after the end of the open field test, mice were individually placed into a 10 cm diameter plastic cylindrical cylinder with water depth of 20 cm and temperature is 22 ± 1°C, forced to swim for 6 min, and continuously monitored using the SuperFst High-throughput Forced Swim Test system (Shanghai Xinruan Information Technology Co., Ltd., China). The time of floating immobility within last 5 min was recorded. At the end of the forced swimming test, the mice were purged with a warm air machine for 5 min after drying with a towel and returned to their cages (Wang et al., Citation2019).
2.8. Tail suspension test
The suspended tail test was performed 3 h after the end of the forced swimming test. The test was performed as previously described (Wang et al., Citation2019). Each mouse was hung up upside down by tails 15 cm above the floor under the edge of the clamp. During a 6-min test period, the immobility time was recorded for the final 5 min period by using the SuperTst High-throughput Tail Suspension Test system (Shanghai Xinruan Information Technology Co., Ltd., China).
2.9. Western blot
After being over-anesthetized with 3% sodium pentobarbital, PFC tissues were immediately homogenised in RIPA lysate containing PMSF (Beyotime, Wuhan, China). The supernatant was taken after centrifuged at 13,000 g for 30 min at 4°C. The BCA detection kit (Bioworld, Atlanta, USA) was used for quantitation of the total protein content of the samples, and samples were mixed with 5×sodium dodecyl sulfate polyacrylamide gel electrophoresis(SDS-PAGE) loading buffer. Briefly, the samples were subjected to SDS-PAGE, 8% separation gel and 5% concentrated gel were prepared by using an SDS-PAGE Gel Quick Preparation Kit (Beyotime, Shanghai, China). Transfer the separated protein to PVDF membrane, then the PVDF membrane was transferred to 5% skimmed milk powder and sealed for 60 min. The membrane was incubated overnight with primary antibody (rabbit anti-ATP8A2, 1:500, Abcam, Cambridge, USA; rabbit anti-β-actin antibodies, 1:10,000, Bioworld, Atlanta, USA) at 4°C. The next day, PVDF membrane was evenly shaken with TBS-T for 10 min × 3 times, and then incubated in second antibody diluent (HRP goat anti-rabbit IgG, 1:10,000, Bioworld, Atlanta, USA) at room temperature for 60 min. Uniformly dripping ECL luminescent liquid (Meilun Biotech Co., Ltd., Dalian, China) on the membrane and using Carestream XBT X-ray Film (Rayco, Xiamen, China) for film development. The expression of each protein band was analysed by ImageJ software.
2.10. Immunofluorescence
Make paraffin sections of brain tissues, then immunofluorescence staining was performed. After the tissue sections were deparaffinised and immersed in citrate buffer for antigen retrieval, the sections were washed slowly with PBS for 5 min on a horizontal shaker × 3 times. BSA (Sigma-Aldrich, St. Louis, USA) was added dropwise on the tissue and blocked at 37°C for 30 min. The slices were incubated overnight with primary antibody (rabbit anti-ATP8A2, 1:200, Abcam, Cambridge, USA; mouse anti-NeuN, 1:1000, Abcam, Cambridge, USA) at 4°C in wet box. The next day, after the wet box was returned to room temperature, the specimens were washed with PBS for 5 min × 3 times. Then, the specimens were incubated with secondary antibodies (Alexa FluorTM 555 donkey anti-rabbit IgG (H + L), 1:500, Bioworld, Atlanta, USA; Alexa FluorTM 488 donkey anti-mouse IgG (H + L), 1:500, Bioworld, Atlanta, USA) for 1 h at 37°C in an incubator before being washed with PBS for 5 min on a shaker × 3 times. Dry the surrounding water with a piece of filter paper and seal the slices. Observed and photographed with a fluorescence microscope (Carl Zeiss AG, Oberkochen, Germany). The mean fluorescence intensity was analysed by ImageJ software.
2.11. Statistical analyses
Statistical analysis was performed using the SPSS 26.0 statistical software (Chicago, IL, USA). The data were described as mean ± standard deviation. Student’s t test, one-way ANOVA or two-way ANOVA was used where proper, and the significance level was set at α = 0.05. Further post-hoc test were performed using the Bonferroni method, and the significance level was set at α = 0.05. The normality test and homogeneity of variance test were performed using Kolmogorow-Smironov and Levene’s Test for Equality of Variances, and the significance level was set at α = 0.1. P < 0.05 was considered to be significant.
3. Results
3.1. AFACM exposure from GD0 to GD18 induced maternal and fetal inflammation after GD12.5/embryonic day(ED)12.5 indicated increased serum IFN-γ levels
In order to determine the starting time of neutralising anti-IFN-γ antibodies, we carried out a pre-experiment. Firstly, the effects of AFACM exposure from GD0 to GD18 on the levels of cytokines in maternal serum, fetal serum and PFC were evaluated (See detailed design in the sheet named Experiment-1 in Supplementary Tables). Then, the effects of AFACM exposure from GD0 to GD12.5 on the levels of cytokines in maternal serum, fetal serum and PFC were further evaluated (See detailed design in the sheet named Experiment-2 in Supplementary Tables).
Maternal serum cytokine levels were analysed from GD0 to GD18 when AFACM exposure occurred. The results showed that AFACM exposure from GD0 to GD18 resulted in increased serum IFN-γ levels in dams in the AFACM group compared with the CON group (t = −2.243, P < 0.05) ((a)). There was no significant difference in serum IL-6 levels between the AFACM and CON groups (t = −0.269, P > 0.05) ((b)). There was no significant difference in serum IL-12p70 levels between the AFACM group and the CON group (t = 0.613, P > 0.05) ((c)). There was no significant difference in serum TNF-α levels between the AFACM group and the CON group (t = 0.261, P > 0.05) ((d)). There was no significant difference in serum MCP-1 levels between the AFACM group and the CON group (t = 0.173, P > 0.05) ((e)). These results suggest that the AFACM exposure during pregnancy from GD0 to GD18 increased the levels of IFN-γ in maternal serum and led to a mild inflammatory state in GD18 dams.
Analysis of cytokine levels of ED18 fetal mice revealed that compared with CON group, the level of IFN-γ increased in the serum of AFACM group (t = −4.688, P < 0.01) ((f)), but the level of TNF-α had no significant change (t = −0.942, P > 0.05) ((g)). IL-6, IL-12p70 and MCP-1 in serum were not detectable. Analyses of cytokine levels in the PFC of ED18 fetal mice revealed that the levels of IFN-γ (t = −3.339, P < 0.01) ((h)) and IL-6 (t = −3.675, P < 0.01) ((i)) of AFACM group were significantly higher than those in CON group. The level of MCP-1 in PFC was not significantly changed (t = 0.970, P > 0.05) ((j)). TNF-α and IL-12p70 in PFC were not detectable. The results suggested that AFACM exposure sustained from GD0 to GD18 resulted in inflammation in fetal mice.
Analysis of cytokine levels in the serum of maternal mice on GD12.5 showed that, compared with the CON group, serum levels of IFN-γ (t = −1.734, P > 0.05) (Figure S1A), IL-6 (t = −0.282, P > 0.05) (Figure S1B), IL-12p70 (t = −0.173, P > 0.05) (Figure S1C), TNF-α (t = −0.167, P > 0.05) (Figure S1D) and MCP-1 (t = −0.792, P > 0.05) (Figure S1E) showed no significant changes. The results suggest that AFACM exposure during pregnancy lasting from GD0 to GD12.5 did not alter the levels of serum inflammatory factors in the maternal mice and did not induce the inflammatory state.
On ED12.5, inflammatory cytokines were undetectable both in serum and PFC of fetal mice, suggesting that AFACM exposure from GD0 to GD12.5 did not induce significant inflammatory states in fetuses.
On the basis of these results reported above, neutralising anti-IFN-γ antibodies were injected in the following experiments on GD12.5, that is, injected before detectable inflammation occurred in dams/offspring.
3.2. The optimal dosage of neutralising anti-IFN-γ antibodies was determined at 0.1 mg/kg by another preliminary experiment
Three different dosages of neutralising anti-IFN-γ antibodies were injected intraperitoneally once a day starting at GD12.5, and the levels of IFN-γ in maternal serum, offspring serum and PFC were measured on the day of delivery (See detailed design in the sheet named Experiment-3 in Supplementary Tables). Detection of serum IFN-γ levels in the dams revealed significant differences in IFN-γ levels between groups (F4,25 = 10.802, P < 0.001). IFN-γ levels were significantly higher in the AFACM group compared to the CON group (P < 0.01). IFN-γ levels were significantly higher in the AFACM + anti-IFN-γ (0.02 mg/kg) group compared with the CON group (P < 0.05). There was no significant change in IFN-γ levels in the AFACM + anti-IFN-γ (0.1 mg/kg) group compared with the CON group (P > 0.05), whereas IFN-γ levels were significantly decreased in the AFACM + anti-IFN-γ (0.1 mg/kg) group compared with the AFACM group (P < 0.05). IFN-γ levels were significantly decreased in the AFACM + anti-IFN-γ (0.5 mg/kg) group compared with the CON group (P < 0.01) (Figure S2A).
Detection of IFN-γ levels in serum of neonatal mice on PND0 revealed a significant difference in IFN-γ levels between groups (F4,25 = 6.594, P < 0.01). IFN-γ levels were significantly higher in the AFACM group compared to the CON group (P < 0.01). IFN-γ levels were significantly higher in the AFACM + anti-IFN-γ (0.02 mg/kg) group compared with the CON group (P < 0.05). There was no significant change in IFN-γ levels in the AFACM + anti-IFN-γ (0.1 mg/kg) group compared with the CON group (P > 0.05), whereas IFN-γ levels were significantly decreased in the AFACM + anti-IFN-γ (0.1 mg/kg) group compared with the AFACM group (P < 0.05). IFN-γ levels were significantly decreased in the AFACM + anti-IFN-γ (0.5 mg/kg) group compared with the CON group (P < 0.05) (Figure S2B).
Detection of IFN-γ levels in the PFC of neonatal mice on PND0 revealed a significant difference between groups (F4,25 = 10.478, P < 0.001). IFN-γ levels were significantly higher in the AFACM group compared to the CON group (P < 0.01). IFN-γ levels were significantly higher in the AFACM + anti-IFN-γ (0.02 mg/kg) group compared with the CON group (P < 0.05). There was no significant change in IFN-γ levels in the AFACM + anti-IFN-γ (0.1 mg/kg) group compared with the CON group (P > 0.05), whereas IFN-γ levels were significantly decreased in the AFACM + anti-IFN-γ (0.1 mg/kg) group compared with the AFACM group (P < 0.001). IFN-γ levels were significantly decreased in the AFACM + anti-IFN-γ (0.5 mg/kg) group compared with the CON group (P < 0.05) (Figure S2C).
The results suggest that the dosage of neutralising anti-IFN-γ antibodies was determined at 0.1 mg/kg.
In order to test whether the neutralising anti-IFN-γ antibodies could penetrate the placental barrier into the fetus circulation, the pregnant mice were intraperitoneally injected with neutralising anti-IFN-γ antibodies or PBS for three consecutive days starting from GD12.5, once a day (See detailed design in the sheet named Experiment-4 in Supplementary Tables). Blood was taken half an hour after the injection. The levels of neutralising antibodies in serum were detected by a commercial enzyme-linked immunosorbent assay kit. The levels of rat IgG1 in anti-IFN-γ group could be detected both in maternal serum (162.13 ± 24.14 ng/mL) (n = 6) and in fetal serum (5.69 ± 1.67 ng/mL) (n = 6). The level of rat IgG1 in PBS group was undectable.
3.3. Maternal AFACM-exposure induced inflammation in serum and PFC of offspring on PND7
In our previous study, we found that maternal AFACM-exposure increased the serum IFN-γ, PFC IFN-γ, PFC IL-6 and PFC TNF-α of offspring on PND0 (Song et al., Citation2021). In order to assess the duration of inflammation, we observed these changes of offspring on PND7 (See detailed design in the sheet named Experiment-5 in Supplementary Tables).
There was a significant difference in serum IFN-γ levels between groups (F4,25 = 10.095, P < 0.001). Serum IFN-γ levels were significantly higher in the AFACM group compared to the CON group (P < 0.05). The effect of AFACM exposure on serum IFN-γ levels was completely blocked by administration of neutralising anti-IFN-γ antibody (AFACM + anti-IFN-γ group vs. AFACM group: P < 0.01; AFACM + anti-IFN-γ group vs. CON group: P > 0.05). Administration of the isotype IgG1 did not have a significant effect on serum IFN-γ levels after AFACM exposure (AFACM + IgG1 group vs. AFACM group: P > 0.05; AFACM + IgG1 group vs. AFACM + anti-IFN-γ group: P < 0.01). Administration of only anti-IFN-γ under physiological conditions resulted in a significant decrease in IFN-γ levels (anti-IFN-γ group vs. CON group: P < 0.05) ((a)).
Detection of IFN-γ, IL-6 and TNF-α levels in the PFC of offspring on PND7 revealed a significant difference in IFN-γ levels between groups (IFN-γ: F4,25 = 8.682, P < 0.001; IL-6: F4,25 = 5.409, P < 0.01; TNF-α: F4,25 = 33.423, P < 0.001). Levels of IFN-γ, IL-6 and TNF-α were significantly higher in the AFACM group compared to the CON group (P < 0.01). The effect of AFACM exposure on IFN-γ, IL-6 and TNF-α were completely blocked by administration of neutralising anti-IFN-γ antibody (AFACM + anti-IFN-γ group vs. AFACM group: P < 0.05; AFACM + anti-IFN-γ group vs. CON group: P > 0.05). Administration of the isotype IgG1 did not have a significant effect on IFN-γ, IL-6 and TNF-α after AFACM exposure (AFACM + IgG1 group vs. AFACM group: P > 0.05; AFACM + IgG1 group vs. AFACM + anti-IFN-γ group: P < 0.05). Administration of only anti-IFN-γ under physiological conditions had no significant effect on IFN-γ, IL-6 and TNF-α in the PFC (anti-IFN-γ group vs. CON group: P > 0.05) ((b–d)).
The results suggest that maternal AFACM-exposure induced inflammation of offspring on PND7, and neutralising anti-IFN-γ antibody normalised the elevated pro-inflammatory cytokine levels after maternal AFACM-exposure, suggesting that IFN-γ is a key mediator of pro-inflammatory cytokine alterations after maternal AFACM-exposure.
3.4. Offspring borned to maternal AFACM-exposure dams showed no longer significant inflammation both in serum and PFC on PND14
Detection of cytokine levels in the serum and PFC of offspring on PND14 revealed no significant differences between groups IFN-γ levels in serum, IFN-γ, IL-6 and TNF-α levels in PFC (IFN-γ in serum: F4,25 = 0.594, P > 0.05; IFN-γ in PFC: F4,25 = 0.697, P > 0.05; IL-6 in PFC: F4,25 = 1.168, P > 0.05; TNF-α in PFC: F4,25 = 0.465, P > 0.05). Serum IFN-γ levels were not significantly altered in the AFACM group, AFACM + anti-IFN-γ group, AFACM + IgG1 group and anti-IFN-γ group compared with the CON group (P > 0.05) ((a)). The levels of IFN-γ, IL-6 and TNF-α in the PFC of the AFACM group, AFACM + anti-IFN-γ group, AFACM + IgG1 group and anti-IFN-γ group were not significantly altered compared with the CON group (P > 0.05) ((b–d). (See detailed design in the sheet named Experiment-5 in Supplementary Tables.) The results suggest that maternal AFACM-exposure did not alter the levels of inflammatory cytokines in the serum and PFC of offspring on PND14.
3.5. Neutralising anti-IFN-γ antibody prevented AFACM-induced abnormal emotional behaviours on PND56 in offspring
To test the effect of maternal AFACM-exposure on behaviours of offspring during adulthood, the mice were assessed for emotion-related behaviours by the three-chamber social test, the open field test, the forced swimming test and tail suspension test from PND56 (See detailed design in the sheet named Experiment-5 in Supplementary Tables).
The three-chamber social test can assess the social behaviour of the mice. In the three-chamber social test, the statistical analysis on the time of sniffing the stranger mouse showed that, compared with the CON group, both AFACM exposure during pregnancy and sex had significant main effects on the time of sniffing the stranger mouse, but there was no interaction between the two (AFACM exposure main effect: F4,50 = 16.522, P < 0.001; sex main effect: F1,50 = 5.563, P < 0.05; AFACM exposure × sex interaction: F4,50 = 0.116, P > 0.05). Subsequent post hoc comparisons showed that, offspring in the AFACM group for both males and females spent less time sniffing the stranger mouse in contrast to controls(P < 0.05). Administration of neutralising anti-IFN-γ antibody completely blocked the effect of AFACM exposure on the time of sniffing the stranger mouse in adult offspring (AFACM + anti-IFN-γ group vs. AFACM group: P < 0.05; AFACM + anti-IFN-γ group vs. CON group: P > 0.05). The results of the above comparisons among treatment groups showed the same trend between males and females ((a)). The statistical analysis on the time of sniffing to the novel mouse showed that, compared with the CON group, both AFACM exposure during pregnancy and sex had significant main effects on the time of sniffing the novel mouse, but there was no interaction between the two (AFACM exposure main effect: F4,50 = 18.762, P < 0.001; sex main effect: F1,50 = 4.619, P < 0.05; AFACM exposure × sex interaction: F4,50 = 0.849, P > 0.05). Subsequent post hoc comparisons showed that, offspring in the AFACM group for both males and females spent less time sniffing the novel mouse in contrast to controls (P < 0.01). Administration of neutralising anti-IFN-γ antibody prevented AFACM-induced novel social deficits in adult offspring (AFACM + anti-IFN-γ group vs. AFACM group: P < 0.01; AFACM + anti-IFN-γ group vs. CON group: P > 0.05). The results of the above comparisons among treatment groups showed the same trend between males and females ((b)). Administration of neutralising anti-IFN-γ antibody prevented AFACM-induced social deficits in adult offspring.
In the open field test, a decrease in time and frequency in the centre of the open field indicated an increase in anxiety. Compared with the CON group, there was a significant main effect of AFACM exposure and sex during pregnancy on the time for the tested mice to enter the central area, but there was no interaction between the two (AFACM exposure main effect: F4,50 = 18.170, P < 0.001; sex main effect: F1,50 = 9.839, P < 0.01; AFACM exposure × sex interaction: F4,50 = 0.292, P > 0.05). Subsequent post hoc comparisons showed that, the time to enter the central area of mice in the AFACM group was significantly reduced in both males and females in contrast to controls (P < 0.01). Administration of neutralising anti-IFN-γ antibody completely blocked the effect of AFACM exposure on the time to enter the central area(AFACM + anti-IFN-γ group vs. AFACM group: P < 0.05; AFACM + anti-IFN-γ group vs. CON group: P > 0.05). The results of the above comparisons among treatment groups showed the same trend between males and females ((c)). There was a significant main effect of AFACM exposure and sex during pregnancy on the number of mice entering the central area, but there was no interaction between the two (AFACM exposure main effect: F4,50 = 19.781, P < 0.001; sex main effect: F1,50 = 13.022, P < 0.01; AFACM exposure × sex interaction: F4,50 = 2.246, P > 0.05). Subsequent post hoc comparisons showed that, the number of entering central area of AFACM group was significantly reduced in both males and females in contrast to controls (P < 0.01). Administration of neutralising anti-IFN-γ antibody completely blocked the effect of AFACM exposure on the number of times of entering the central region (AFACM + anti-IFN-γ group vs. AFACM group: P < 0.01; AFACM + anti-IFN-γ group vs. CON group: P > 0.05). The results of the above comparisons among treatment groups showed the same trend between males and females ((d)). Administration of neutralising anti-IFN-γ antibody prevented AFACM-induced anxiety-like behaviours in adult offspring.
The forced swimming test and tail suspension test can assess depression-like behaviours in adult offspring. In forced swimming test, there was a significant main effect of AFACM exposure on the floating immobility time, the main effect of sex was not significant, and there was a significant interaction between the two (AFACM exposure main effect: F4,50 = 24.719, P < 0.001; sex main effect: F1,50 = 0.026, P > 0.05; AFACM exposure × sex interaction: F4,50 = 4.028, P < 0.01). Subsequent post hoc comparisons showed that the floating immobility time of AFACM group was significantly increased in both males and females in contrast to controls (P < 0.05). In the CON group, the floating immobility time of males was significantly lower than females (P < 0.05), while the floating immobility time of males in the AFACM group was not significantly different from that of females (P > 0.05). Administration of neutralising anti-IFN-γ antibody completely blocked the effect of AFACM exposure on the floating immobility time (AFACM + anti-IFN-γ group vs. AFACM group: P < 0.001; AFACM + anti-IFN-γ group vs. CON group: P > 0.05) ((e)).
In tail suspension test, there was a significant main effect of AFACM exposure on the hanging immobility time, the main effect of sex was not significant, and there was a significant interaction between the two (AFACM exposure main effect: F4,50 = 37.855, P < 0.001; sex main effect: F1,50 = 0.293, P > 0.05; AFACM exposure × sex interaction: F4,50 = 3.221, P < 0.05). Subsequent post hoc comparisons showed that the hanging immobility time of AFACM group was significantly increased in both males and females in contrast to controls (P < 0.01). In the CON group, the hanging immobility time of males was significantly lower than females (P < 0.05), while the hanging immobility time of males in the AFACM group was longer than that of females (P < 0.05). Administration of neutralising anti-IFN-γ antibody completely blocked the effect of AFACM exposure on the hanging immobility time (AFACM + anti-IFN-γ group vs. AFACM group: P < 0.01; AFACM + anti-IFN-γ group vs. CON group: P > 0.05). Administration of neutralising anti-IFN-γ antibody prevented AFACM-induced depression-like behaviours in adult offspring ((f)).
Behavioural experiment results suggested that the offspring of AFACM group showed significant abnormal emotional behaviours in adulthood, and the exposure to AFACM during pregnancy combined with the administration of neutralising anti-IFN-γ antibodies could normalise the emotional-related behaviours of the offspring.
3.6. IFN-γ mediated the PFC ATP8A2 down-regulation on PND7 caused by maternal AFACM-exposure
We further analysed the effect of maternal AFACM exposure on the PFC ATP8A2 (See detailed design in the sheet named Experiment-6 in Supplementary Tables). The expression levels of ATP8A2 in the PFC of offspring on PND7 were detected, significant differences were found between the groups (F4,25 = 7.561, P < 0.001). Compared with the CON group, the ATP8A2 expression level in the AFACM group was significantly decreased (P < 0.001). Under the condition that IFN-γ was neutralised by the administration of neutralising anti-IFN-γ antibody, the effect of AFACM exposure on ATP8A2 expression was completely blocked (AFACM + anti-IFN-γ group vs. AFACM group: P < 0.01; AFACM + anti-IFN-γ group vs. CON group: P > 0.05). The administration of the isotype IgG1 had no significant effect on the expression of ATP8A2 after exposure to AFACM (AFACM + IgG1 group vs. AFACM group: P > 0.05; AFACM + IgG1 group vs. AFACM + anti-IFN-γ group: P < 0.01). Under physiological conditions, administration of anti-IFN-γ had no significant effect on the expression of ATP8A2 in PFC (anti-IFN-γ group vs. CON group: P > 0.05) ((a)).
ATP8A2 expression levels in CON group, AFACM group and AFACM + anti-IFN-γ group were further detected by immunofluorescence, and immunofluorescence results supported the above WB results. The significant differences were found between the groups (F2,15 = 13.271, P < 0.001). Compared with the CON group, the ATP8A2 expression level in the AFACM group was significantly decreased (P < 0.01). Under the condition that IFN-γ was neutralised by the administration of neutralising anti-IFN-γ antibody, the effect of AFACM exposure on ATP8A2 expression was completely blocked (AFACM + anti-IFN-γ group vs. AFACM group: P < 0.001; AFACM + anti-IFN-γ vs. CON group: P > 0.05) (). These results suggested that IFN-γ mediated the down-regulation of ATPA2 in PFC caused by maternal AFACM exposure.
4. Discussion
The results showed that IFN-γ in blood is the key mediator of brain inflammation and abnormal emotional behaviours of offspring caused by AFACM exposure during pregnancy. After neutralising IFN-γ, the levels of inflammatory cytokines in the PFC and emotion-related behavioural performance were completely restored.
We found the effects of maternal AFACM-exposure on offspring: inflammation, abnormal emotional behaviours and the down-regulated expression of ATP8A2 in the PFC. Altered inflammation early in life induced abnormal emotional behaviours (Buehler, Citation2011; Depino, Citation2013; Salim et al., Citation2012), and abnormal emotional behaviour was reported to be associated with down-regulated expression of ATP8A2 in the PFC (Chen et al., Citation2017). Therefore, abnormal emotional behaviours and down-regulated expression of ATP8A2 may be down-stream events of inflammation. The results of our experiments support this deduction because neutralising IFN-γ normalised the emotional-related behaviours and ATP8A2 expression of offspring.
It is speculated here that inflammation in the PFC resulted from peripheral inflammation induced by maternal AFACM-exposure, basing on several in facts: (1) Our previous study has shown that three pro-inflammatory factors, including IFN-γ, in the PFC increased while only IFN-γ increased in circulating blood of offspring on PND0 after maternal AFACM-exposure (Song et al., Citation2021). (2) The previous study found that the level of IFN-γ in serum was positively correlated with the levels of each of the three cytokines that increased significantly in the PFC of offspring on PND0 after maternal AFACM-exposure (Song et al., Citation2021). (3) Neutralising the over-produced IFN-γ in serum of offspring after maternal AFACM-exposure completely normalised the levels of cytokines in the PFC, as well as the abnormal emotional behaviours and the down-regulated expression of ATP8A2 in the PFC ( and ).
Data in showed that administration of IgG1 made no effects on the levels of ATP8A2 in the PFC of offspring after maternal AFACM-exposure and that administration of mere neutralising anti-IFN-γ antibodies made no effects on the levels of ATP8A2 in the PFC of offspring who were born to normal dams. Therefore, only three groups, that is, CON group, AFACM group and AFACM + anti-IFN-γ group, were set in immunofluorescence test so as to comply with the requirements of ethics.
It has been reported that sex factor may affect brain development or be associated to neonatal immune activation (Cai et al., Citation2016). This is confirmed by the results of FST and TST tasks performances in the present study ((e and f) indicated by the observed significant treatment × sex interaction effects. However, no significant sex-related effects in neonates born to AFACM-exposed dams were found in our previous study (Song et al., Citation2021). This may be due to that sex factor makes difference mainly by gonadal hormones, which becomes vital after puberty period starts (Cai et al., Citation2016). Therefore, sex factor was not taken in consideration in the other experiments of the present study evaluating the effects of AFACM in offspring before puberty (Custodio et al., Citation2018; Kuo, Citation2016).
In conclusion, IFN-γ is a key mediator of the inflammatory state and down regulation of ATP8A2 expression in the PFC, as well as abnormal emotional behaviours of the offspring caused by maternal AFACM exposure. These findings suggest that maternal AFACM exposure may affect the development and health of offspring via elevated IFN-γ. This study enriched our understanding of fetal development damage caused by simultaneous exposure to multiple safe doses of food additives and pesticides during pregnancy, and thus provided clues for exploring possible treatment strategies.
Acknowledgements
We thank Mr. Wenzhi Wen (from GDPU) and Ms. Qianyi Zhong (from GDPU) for their valuable discussions and help with this investigation.
Disclosure statement
No potential conflict of interest was reported by the author(s).
Additional information
Funding
References
- Andersen, J. P., Vestergaard, A. L., Mikkelsen, S. A., Mogensen, L. S., Chalat, M., & Molday, R. S. (2016). P4-ATPases as phospholipid flippases—structure, function, and enigmas. Frontiers in Physiology, 7, 275. https://doi.org/10.3389/fphys.2016.00275
- Buehler, M. R. (2011). A proposed mechanism for autism: An aberrant neuroimmune response manifested as a psychiatric disorder. Medical Hypotheses, 76(6), 863–870. https://doi.org/10.1016/j.mehy.2011.02.038
- Cai, K. C., van Mil, S., Murray, E., Mallet, J. F., Matar, C., & Ismail, N. (2016). Age and sex differences in immune response following LPS treatment in mice. Brain, Behavior, and Immunity, 58, 327–337. https://doi.org/10.1016/j.bbi.2016.08.002
- Chen, J., Wang, Z., Zhang, S., Ai, Q., Chu, S., & Chen, N.-h. (2017). Possible target-related proteins of stress-resistant rats suggested by label-free proteomic analysis. RSC Advances, 7(65), 40957–40964. https://doi.org/10.1039/C7RA04212D
- Choi, H., Andersen, J. P., & Molday, R. S. (2019). Expression and functional characterization of missense mutations in ATP8A2 linked to severe neurological disorders. Human Mutation, 40(12), 2353–2364. https://doi.org/10.1002/humu.23889
- Coleman, J. A., Kwok, M. C., & Molday, R. S. (2009). Localization, purification, and functional reconstitution of the P4-ATPase Atp8a2, a phosphatidylserine flippase in photoreceptor disc membranes. Journal of Biological Chemistry, 284(47), 32670–32679. https://doi.org/10.1074/jbc.M109.047415
- Coleman, J. A., & Molday, R. S. (2011). Critical role of the β-subunit CDC50A in the stable expression, assembly, subcellular localization, and lipid transport activity of the P4-ATPase ATP8A2. Journal of Biological Chemistry, 286(19), 17205–17216. https://doi.org/10.1074/jbc.M111.229419
- Custodio, C. S., Mello, B. S. F., Filho, A., de Carvalho Lima, C. N., Cordeiro, R. C., Miyajima, F., Reus, G. Z., Vasconcelos, S. M. M., Barichello, T., Quevedo, J., de Oliveira, A. C., de Lucena, D. F., & Macedo, D. S. (2018). Neonatal immune challenge with lipopolysaccharide triggers long-lasting sex- and age-related behavioral and immune/neurotrophic alterations in mice: Relevance to autism spectrum disorders. Molecular Neurobiology, 55(5), 3775–3788. https://doi.org/10.1007/s12035-017-0616-1
- Deng, J., Song, L., Yang, Z., Zheng, S., Du, Z., Luo, L., Liu, J., Jin, X., & Yang, J. (2021). Neonatal LPS exposure reduces ATP8A2 level in the prefrontal cortex in mice via increasing IFN-γ level. Brain Research Bulletin, 171, 103–112. https://doi.org/10.1016/j.brainresbull.2021.03.015
- Depino, A. M. (2013). Peripheral and central inflammation in autism spectrum disorders. Molecular and Cellular Neuroscience, 53, 69–76. https://doi.org/10.1016/j.mcn.2012.10.003
- Docea, A. O., Gofita, E., Goumenou, M., Calina, D., Rogoveanu, O., Varut, M., Olaru, C., Kerasioti, E., Fountoucidou, P., Taitzoglou, I., Zlatian, O., Rakitskii, V. N., Hernandez, A. F., Kouretas, D., & Tsatsakis, A. (2018). Six months exposure to a real life mixture of 13 chemicals’ below individual NOAELs induced non monotonic sex-dependent biochemical and redox status changes in rats. Food and Chemical Toxicology, 115, 470–481. https://doi.org/10.1016/j.fct.2018.03.052
- Docea, A. O., Goumenou, M., Calina, D., Arsene, A. L., Dragoi, C. M., Gofita, E., Pisoschi, C. G., Zlatian, O., Stivaktakis, P. D., Nikolouzakis, T. K., Kalogeraki, A., Izotov, B. N., Galateanu, B., Hudita, A., Calabrese, E. J., & Tsatsakis, A. (2019). Adverse and hormetic effects in rats exposed for 12 months to low dose mixture of 13 chemicals: RLRS part III. Toxicology Letters, 310, 70–91. https://doi.org/10.1016/j.toxlet.2019.04.005
- Himmerich, H., Fulda, S., Sheldrick, A. J., Plumakers, B., & Rink, L. (2010). IFN-γ reduction by tricyclic antidepressants. The International Journal of Psychiatry in Medicine, 40(4), 413–424. https://doi.org/10.2190/PM.40.4.e
- Kuo, S. M. (2016). Gender difference in bacteria endotoxin-induced inflammatory and anorexic responses. PLoS One, 11(9), e0162971. https://doi.org/10.1371/journal.pone.0162971
- Li, J., Liu, W., Xia, W., Zhao, H., Zhou, Y., Li, Y., Wu, C., Liu, H., Zhang, B., Zhu, Y., Hu, J., Wang, X., Ye, D., Xu, S., & Cai, Z. (2019). Variations, determinants, and coexposure patterns of personal care product chemicals among Chinese pregnant women: A longitudinal study. Environmental Science & Technology, 53(11), 6546–6555. https://doi.org/10.1021/acs.est.9b01562
- Lichtblau, N., Schmidt, F. M., Schumann, R., Kirkby, K. C., & Himmerich, H. (2013). Cytokines as biomarkers in depressive disorder: current standing and prospects. International Review of Psychiatry, 25(5), 592–603. https://doi.org/10.3109/09540261.2013.813442
- Madore, C., Leyrolle, Q., Lacabanne, C., Benmamar-Badel, A., Joffre, C., Nadjar, A., & Laye, S. (2016). Neuroinflammation in autism: Plausible role of maternal inflammation, dietary omega 3, and microbiota. Neural Plasticity, 2016, 3597209. https://doi.org/10.1155/2016/3597209
- O’Connor, J. C., Andre, C., Wang, Y., Lawson, M. A., Szegedi, S. S., Lestage, J., Castanon, N., Kelley, K. W., & Dantzer, R. (2009). Interferon-gamma and tumor necrosis factor-alpha mediate the upregulation of indoleamine 2,3-dioxygenase and the induction of depressive-like behavior in mice in response to bacillus Calmette-Guerin. The Journal of Neuroscience, 29(13), 4200–4209. https://doi.org/10.1523/JNEUROSCI.5032-08.2009
- Palazidou, E. (2012). The neurobiology of depression. British Medical Bulletin, 101(1), 127–145. https://doi.org/10.1093/bmb/lds004
- Pisanello, D. (2014). Springerbriefs in molecular science. Chemistry of Foods: Eu Legal and Regulatory Approaches, 15–77. https://doi.org/10.1007/978-3-319-03434-8_2
- Salim, S., Chugh, G., & Asghar, M. (2012). Advances in protein chemistry and structural biology. Advances in Protein Chemistry and Structural Biology, 88, 1–25. https://doi.org/10.1016/B978-0-12-398314-5.00001-5
- Shulzhenko, N., Dong, X., Vyshenska, D., Greer, R. L., Gurung, M., Vasquez-Perez, S., Peremyslova, E., Sosnovtsev, S., Quezado, M., Yao, M., Montgomery-Recht, K., Strober, W., Fuss, I. J., & Morgun, A. (2018). CVID enteropathy is characterized by exceeding low mucosal IgA levels and interferon-driven inflammation possibly related to the presence of a pathobiont. Clinical Immunology, 197, 139–153. https://doi.org/10.1016/j.clim.2018.09.008
- Song, L., Wen, W., Liu, J., Jin, X., & Yang, J. (2021). Inflammatory state and autism-like behavioral phenotype of offspring induced by maternal exposure to low-dose chemical mixtures during pregnancy in mice. Zhejiang da Xue Xue Bao. Yi Xue Ban, 50(3), 279–289. https://doi.org/10.3724/zdxbyxb-2021-0182
- Sullivan, E. L., Nousen, E. K., Chamlou, K. A., & Grove, K. L. (2012). The impact of maternal high-fat diet consumption on neural development and behavior of offspring. International Journal of Obesity Supplements, 2(S2), S7–S13. https://doi.org/10.1038/ijosup.2012.15
- Tang, G., Gudsnuk, K., Kuo, S. H., Cotrina, M. L., Rosoklija, G., Sosunov, A., Sonders, M. S., Kanter, E., Castagna, C., Yamamoto, A., Yue, Z., Arancio, O., Peterson, B. S., Champagne, F., Dwork, A. J., Goldman, J., & Sulzer, D. (2014). Loss of mTOR-dependent macroautophagy causes autistic-like synaptic pruning deficits. Neuron, 83(5), 1131–1143. https://doi.org/10.1016/j.neuron.2014.07.040
- Tsatsakis, A. M., Docea, A. O., Calina, D., Buga, A. M., Zlatian, O., Gutnikov, S., Kostoff, R. N., & Aschner, M. (2019). Hormetic Neurobehavioral effects of low dose toxic chemical mixtures in real-life risk simulation (RLRS) in rats. Food and Chemical Toxicology, 125, 141–149. https://doi.org/10.1016/j.fct.2018.12.043
- Tsatsakis, A. M., Kouretas, D., Tzatzarakis, M. N., Stivaktakis, P., Tsarouhas, K., Golokhvast, K. S., Rakitskii, V. N., Tutelyan, V. A., Hernandez, A. F., Rezaee, R., Chung, G., Fenga, C., Engin, A. B., Neagu, M., Arsene, A. L., Docea, A. O., Gofita, E., Calina, D., Taitzoglou, I., … Tsitsimpikou, C. (2017). Simulating real-life exposures to uncover possible risks to human health: A proposed consensus for a novel methodological approach. Human & Experimental Toxicology, 36(6), 554–564. https://doi.org/10.1177/0960327116681652
- Wang, X., Yang, J., Zhang, H., Yu, J., & Yao, Z. (2019). Oral probiotic administration during pregnancy prevents autism-related behaviors in offspring induced by maternal immune activation via anti-inflammation in mice. Autism Research, 12(4), 576–588. https://doi.org/10.1002/aur.2079
- Woodruff, T. J., Zota, A. R., & Schwartz, J. M. (2011). Environmental chemicals in pregnant women in the United States: NHANES 2003-2004. Environmental Health Perspectives, 119(6), 878–885. https://doi.org/10.1289/ehp.1002727
- Xu, Q., Yang, G. Y., Liu, N., Xu, P., Chen, Y. L., Zhou, Z., Luo, Z. G., & Ding, X. (2012). P4-ATPase ATP8A2 acts in synergy with CDC50A to enhance neurite outgrowth. FEBS Letters, 586(13), 1803–1812. https://doi.org/10.1016/j.febslet.2012.05.018