ABSTRACT
The number of Alzheimer’s disease (AD) affected patients is increasing without any effective cure and the etiology remains to be understood. Inflammations, oxidative stress, Aβ, and tauopathy are associated factors of AD. Sodium butyrate (NaB) is an HDAC inhibitor profoundly found to be neuroprotective. We have investigated the neuroprotective effects of NaB in SH-SY5Y cells stimulated with TNF-α/Aβ in SH-SY5Y cells and LPS-induced BV-2 cells. The cell viability and NO production were also investigated by MTT and Griess reagent assay. The expressions of APP/BACE, Aβ, and tau phosphorylation and the apoptotic regulators, including P-53, and caspase-1 were analysed by western blot analysis. Our findings exerted that NaB ameliorated cell death and inhibited NO production in Aβ-induced SH-SY5Y cells and LPS in BV-2 cells. NaB notably decreased the expression of tau hyperphosphorylation in TNF-α-stimulated SH-SY5Y and LPS-induced BV-2 cells. NaB remarkably attenuated APP/BACE and Aβ expressions in TNF-α-induced SH-SY5Y cells. Cell viability was restored by NaB and downregulated apoptotic proteins p-53, caspase-1 level in aggregated Aβ-induced SH-SY5Y cells. NaB increased Nrf-2/HO-1 expressions and substantially reversed the reactive oxygen species in Aβ-induced SH-SY5Y cells. Altogether, our results suggest that NaB could be a potential therapeutic agent against AD.
GRAPHICAL ABSTRACT
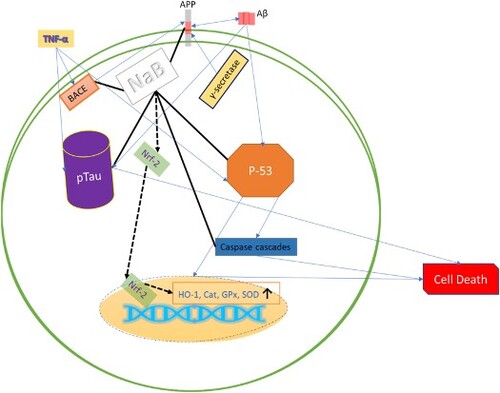
1. Introduction
Alzheimer’s disease is a progressive neurodegenerative disease (NDD) and the most common form of dementia. Dementia describes a pattern of deterioration in an individual’s memory and thinking ability that affects at least two areas of cognition. Most cases occur after age 65 years, representing late-onset AD, but cases occurring before age 65 years are much smaller, less than 5% of all cases, and are termed early-onset AD (Bellenguez et al., Citation2022; Long & Holtzman, Citation2019). Above 25 million population have been reported for affected by dementia from AD worldwide in 2022. AD is an age-related progressive disease with many other risk factors (smoking, obesity, diabetes, CVD, lifestyle, and so on), and the aged population with AD is increasing (Qiu et al., Citation2009). There is often a discrepancy between the clinical diagnosis of AD and the neuropathological findings. Therefore, non-invasive indicators that can improve confidence in such lifelong decisions are paramount. This biological indication is also of great importance in the preclinical stages of the continuum of AD. Although amyloid beta (Aβ) pathology of the brain is accumulating, the individual does not have cognitively unimpaired (CU). However, it remains unclear how blood biomarkers influence the preclinical assessment of AD. As anti-Aβ treatment trials move towards preclinical evaluation, inexpensive tools to reduce the number of lumbar punctures and positron emission tomography (PET) imaging during recruitment are needed. In addition, blood biomarkers will reduce recruitment time and enhance the participation of a more diverse population that is a better representative of the global aging population. In fact, blood measures tau phosphorylation. Neurofilament lumen (NFL) and Aβ have been shown to be altered in preclinical AD and may distinguish this condition from pathologically altered CU patients without AD (Milà-Alomà et al., Citation2022). AD is found one of the most affected diseases due to neurodegeneration without any effective cure.
SH-SY5Y is a neuronal cell line which widely studied for in vitro AD models (Fernandes et al., Citation2018; Riegerová et al., Citation2021) and BV-2 murine microglia cell lines are highly associated with to study of mechanisms of AD. Neuroinflammation and neuronal cell death are highly associated with AD (Heneka et al., Citation2015), inflammatory cytokines and mediators (LPS, TNF-α, ILs and etc.) activates microglia, produce inflammatory cytokines, and shows autocrine and paracrine activity, which affects healthy cells in the nervous system, and leads to inflammatory cell death. Anti-inflammatory effects in SH-SY5Y and BV-2 cells could be a remarkable indication of their ability to combat AD.
Tau hyperphosphorylation and Aβ are the hallmarks of AD pathogenesis. Tau act as a stabiliser of the cellular skeleton of neuronal cells and tau phosphorylation causes dissociation from the microtubule and leads to cell death. Moreover, dissociated tau plaque forms the neurotoxic neurofibrillary tangle (NFT) (Hamilton et al., Citation2016; Ittner et al., Citation2010; Karaahmet et al., Citation2022). On the other hand, Aβ produces by the cleaving of beta-amyloid secretase enzyme (BACE) from a native cellular protein called amyloid beta precursor protein (APP). The Aβ mechanisms are not fully understood yet. Hence, Aβ could form aggregated, fibrils, which have shown neurotoxic effects (Chételat, Citation2013; Lee et al., Citation2022; Oguchi et al., Citation2017; Wilson et al., Citation1999), and increased Aβ level has found in AD patients. Nevertheless, Aβ and tauopathy are two etiologies of AD that could be interrelated (Bayazid & Lim, Citation2022; Bayazid, Kim, et al., Citation2022; Ittner et al., Citation2010). Therefore, neutralising the abnormal Aβ production process, tau phosphorylation and attenuation of Aβ-induced neurotoxicity by aggregation, fibrils, and senile plaques envisaged a promising outcome against AD progression (Graphical Abstract). Moreover, Aβ is a ligand of TREM2, which is surface receptor and plays vital role in AD progression (Carmona et al., Citation2018) and leads to inflammation and cell death (Gratuze et al., Citation2018).
Since there is no effective therapeutic agent to cure AD. NaB showed neuroprotective effects with antioxidant and anti-inflammatory activities in our previous study in vitro and in vivo (Bayazid, Kim, et al., Citation2022). Intriguingly, NaB alleviates the blood–brain barrier (BBB), which is disrupted by inflammation, TBI, stroke, and so on (Alpino et al., Citation2022; Dong & Cui, Citation2022). NaB potentiates monocarboxylate transporter 1 (MCT1) and Sodium-coupled monocarboxylate transporter 1 (SMCT1) to promote cell metabolism (Bayazid, Jeong, et al., Citation2022) and agonist of GPR41, GPR43, and GPR109a (Luo et al., Citation2020; Mirzaei et al., Citation2021). NaB is a well-known histone acetylase, access to the BBB, neuroprotective, and mitigates disrupted BBB. Taken altogether, these activities could be a strong prediction for therapeutic agent against AD along with other NDDs.
2. Materials and methods
2.1. Chemicals and reagents
Amyloid Beta (25–35) (CAS No: 131602-53-4), 3-(4,5-dimethylthiazol-2-yl)-2,5 diphenyltetrazolium bromide (MTT), NaB (CAS No: 156-54-7) were purchased from Sigma Aldrich. DMEM (Dulbecco’s Modified Eagle’s Medium), DPBS, Penicillin Streptomycin (P.S.), and Trypsin EDTA were purchased from WELGENE Inc. (Seoul, Korea). Western blot primary and secondary antibodies were collected from Cell Signaling Technology and were purchased from Abcam (Cambridge, UK). All other chemicals were analytical grade.
2.2. Cell culture
Human neuroblastoma cell line SH-SY5Y and murine microglia BV-2 cells were purchased from the Korean cell line bank. SH-SY5Y and BV-2 cells were maintained in DMEM supplemented with 10% fetal bovine serum (FBS) and 1% P.S. in a humidified cell incubator.
2.3. Cell viability assay
The cell viability was investigated by MTT assay as previously mentioned (Al Mijan et al., Citation2019; Bayazid et al., Citation2021; Bayazid, Park, et al., Citation2022; Jaradat et al., Citation2023) with slight modifications. BV-2 cells were seeded as 5 × 104 per well in a 96-well plate. After 24 h of incubation, cells were treated with various concentrations of NaB with or without LPS for another 24 h. Then, 0.5 mg/mL MTT solution (final) was added and incubated for 2 h to form formazan blue and dissolved in DMSO. Finally, the absorbance was taken in a microplate reader at 570 nm.
2.4. Nitric oxide assay
Nitric Oxide (NO) assay was performed by Griess reagent. BV-2 cells were seeded as 5 × 104 per well in a 96-well plate (Bayazid, Jeong, et al., Citation2022). After 24 h of incubation, cells were treated with different concentrations of LPS with or without NaB for another 24 h. The 80 μL of supernatant from each well was taken into another 96-well plate an equal amount of Griess reagent was added and the absorbance was taken at 540 nm.
2.5. Aβ (25–35) preparation
The Aβ (25–35) was purchased from Sigma and dissolved in PBS. The aggregated Aβ was formed according to the previously mentioned (Lin et al., Citation2020). Briefly, 1 mM of Aβ in PBS was incubated at 37°C for 5 days before cell treatments. After getting the aggregated form of Aβ, the SH-SY5Y cells were treated with Aβ with or without NaB.
2.6. Aβ-induced cell viability and NO assay
The human neuroblastoma cell line SH-SY5Y cells were treated with Aβ with/without NaB. Briefly, the SH-SY5Y cells were seeded as 5 × 104 per well in a 96-well plate. Then, aggregated Aβ was treated and following MTT and NO assay were conducted as previously mentioned (Awada et al., Citation2013; Bayazid & Jang, Citation2021; Jia et al., Citation2021).
2.7. Aβ-induced ROS assay
The ROS of Aβ-induced SH-SY5Y cells were measured by DCFH-DA methods. The SH-SY5Y cells were treated with Aβ with/without NaB. Briefly, the SH-SY5Y cells were seeded as 5 × 104 per well in a black 96-well plate. Then, aggregated Aβ was treated as previously mentioned (Jia et al., Citation2021) with slight modifications. After treatment of 24 h, the cells were treated with 10 μM of DCFH-DA for 30 min and the fluorescence was taken (excitation at 485 nm and emission at 530 nm).
2.8. Western blot analysis
After treating the cells, washing the cells with ice-cold PBS and the protein from the cells was isolated by PRO PREP (iNITRON) lysis buffer. Then, an equal amount of protein was loaded into the SDS gel and electrophoresis. Afterward, the protein was transferred into the PVDF membrane and cut according to the corresponding primary antibodies kDa (Bayazid, Kim, et al., Citation2022; Lin et al., Citation2020). After blocking the membrane with 5% BSA, the membranes were incubated with primary antibodies overnight at 4°C and then incubated with secondary antibody for 1 h at room temperature. Finally, the images of bands were detected by ChemiDoc XRS + (Bio-Rad) using enhanced chemiluminescence (ECL) kits. The bands were analysed by ImageJ.
2.9. Statistical analysis
All data are presented as the mean ± standard deviation for at least 3 independent experiments and statistical analyses were performed using Microsoft Excel 2016 edition and GraphPad Prism 5.0 software (GraphPad Software, Inc., San Diego, CA, United States). The significance of differences between groups was determined by using one-way analysis of variance.
3. Results
3.1. Effects of NaB on cell viability and NO production in LPS-induced BV-2 cells
The cell viability of NaB was previously investigated in SH-SY5Y cells. As previously, NaB did not show any toxic effects up to 5 mM in BV-2 cells and ameliorated cell viability in LPS-induced BV-2 cells (). NaB fully recovered the cell viability from the concentration of 0.5 mM in LPS-induced BV-2 cells. LPS drastically increased NO about 15 μM in BV-2 cells and NaB significantly reduced NO in a dose-dependent manner.
Figure 1. (a) The effect of cell viability sodium butyrate (NaB) in the BV-2 cells, (b) the effect NaB on cell viability in LPS-induced BV-2 cells, and (c) NO production against in LPS-induced BV-2 cells. Histograms were presented as mean ± SD, where n = 3. The p-value is determined by ANOVA with Tukey’s multiple test comparison and p-value less than 0.05 was expressed as statistically significant. *p < 0.05, **p < 0.001, and ***p < 0.0001 compared to the only TNF-α-treated group.

3.2. Effects of NaB on tau phosphorylation
Tau protein holds the microtubule which is the skeleton of a cell and phosphorylation of tau dissociates tau protein from the microtubule and loss of the integrity of cells and leads to cell death. LPS in BV-2 and TNF-α in SH-SY5Y cells drastically increased tau phosphorylation. NaB attenuated tau phosphorylation in LPS-induced BV-2 cells and TNF-α induced SH-SY5Y cells (). Whereas TNF-α or LPS drastically increased tau phosphorylation and reversed remarkably by NaB treatment.
Figure 2. The effect of NaB on tau phosphorylation in the TNF-α-induced SH-SY5Y cells and LPS-induced BV-2 cells, respectively. Histograms were presented as mean ± SD, where n = 3. The p-value is determined by ANOVA with Tukey’s multiple test comparison and p-value less than 0.05 was expressed as statistically significant. *p < 0.05, **p < 0.001, and ***p < 0.0001 compared to the only TNF-α-treated group.

3.3. Effects of NaB on APP/BACE and Aβ in TNF-α induced SH-SY5Y cells
Aβ plays the potent role in AD progression. Excessive Aβ secretion may form oligomer, fibril, and aggregated Aβ which shows disruption of cellular function and have toxic effects. Wherein, BACE cleaved in APP and produces Aβ and Aβ is a pivotal biomarker of AD. NaB decreased APP in TNF-α induced SH-SY5Y cells (). Aβ is produced from the APP by BACE activity and NaB reversed BACE expression and Aβ production in TNF-α induced SH-SY5Y cells. NaB ameliorated Aβ expression by downregulating APP/BACE signalling in TNF-α induced SH-SY5Y cells.
Figure 3. The effect of NaB on the expression of APP, BACE, and Aβ in the TNF-α-induced SH-SY5Y cells. Histograms were presented as mean ± SD, where n = 3. The p-value is determined by ANOVA with Tukey’s multiple test comparison and a p-value less than 0.05 was expressed as statistically significant. Where *p < 0.05, **p < 0.001, and ***p < 0.0001 compared to the only TNF-α-treated group.

3.4. Effects of NaB on Aβ-induced cytotoxicity and APP/BACE expression
NaB ameliorated aggregated Aβ-induced cell death in . Cell viability had been decreased by Aβ about 62% at 20 μM and 60% at 50 μM of Aβ respectively compared to the control group. 1 mM of NaB treatment ameliorated cell viability in Aβ-induced SH-SY5Y cells. Excessive Aβ may cause AD by neurodegeneration and increased NO in SH-SY5Y cells. NaB reduced the NO production of different concentrations of Aβ-induced SH-SY5Y cells. Aβ drastically increased APP and BACE expression (Lee et al., Citation2018) and was reversed by NaB treatment. Furthermore, NaB also restored pro-apoptotic regulator P-53 and cleaved caspase-1, whereas Aβ increased P-53 and cleaved caspase-1 about 2 folds. NaB also reversed inflammatory markers like TNF-α, IL-6, and GR expressions in aggregated Aβ-induced SH-SY5Y cells illustrated in the Supplementary Figure (Figure S1).
Figure 4. The effect of NaB on cell viability, apoptotic, and APP, BACE protein expressions in the aggregated Aβ(25–35)-induced SH-SY5Y cells. Histograms were presented as mean ± SD, where n = 3. The p-value is determined by ANOVA with Tukey’s multiple test comparison and p-value less than 0.05 was expressed as statistically significant. *p < 0.05, **p < 0.001, and ***p < 0.0001 compared to the only Aβ-treated group.

3.5. Effects of NaB on Nrf-2/HO-1 expressions and ROS production in Aβ-induced SH-SY5Y cells
Nrf-2 and HO-1 signalling are cellular antioxidant defense mechanisms. Aβ disrupted endogenous antioxidant mechanisms and reduced Nrf-2 to about 0.4 foldchange and NaB upregulated Nrf-2 expressions. Moreover, NaB restored HO-1 expression in aggregated Aβ-induced SH-SY5Y cells. ROS plays a pivotal role in maintaining cell growth, survival, and so on. Excessive ROS leads to cell death. Aβ increased ROS generation by about 140% of the control group. Hence, NaB treatment remarkably restored ROS generation in Aβ-induced SH-SY5Y cells ().
Figure 5. The effect of NaB on Nrf-2 and HO-1 protein expressions and ROS production in the aggregated Aβ(25–35)-induced SH-SY5Y cells. Histograms were presented as mean ± SD, where n = 3. The p-value is determined by ANOVA with Tukey’s multiple test comparison and p-value less than 0.05 was expressed as statistically significant. *p < 0.05, **p < 0.001, and ***p < 0.0001 compared to the only Aβ-treated group.

4. Discussions
The causes of AD include Aβ accumulation, tau pathology, oxidative stress, inflammation, etc. Aggregated Aβ is known to exhibit neurotoxicity that contributes to neuronal cell death, and this event is implicated as a key factor initiating the pathogenesis of AD. Once tau is hyperphosphorylated, as observed after Aβ treatment in this study, it is unable to maintain cellular structure and leads to apoptosis (Jia et al., Citation2021; Lin et al., Citation2020).
Apoptosis plays a crucial role in the progression of NDDs by neuronal cell loss (Radi et al., Citation2014). We evaluated the protective actions of NaB against LPS-induced microglia cells that represent many features of AD. LPS is a product of the membrane from gram-negative bacteria. NaB remarkably ameliorated cell death and significantly reduced NO productions in LPS-induced BV-2 cells that represent many features of NDDs. Tau protein is the stabiliser of the cytoskeleton (microtubule) and phosphorylation of tau makes the neuronal cell dysfunctional (Ittner et al., Citation2010). NaB restored tau phosphorylation in SH-SY5Y and BV-2 cells, whereas LPS or TNF-α drastically increased tau phosphorylation. That could be significant potentialities of NaB against AD. Aβ from APP could form aggregated, fibrils, which have shown neurotoxic effects and have detrimental effects on autophagic clearance (Chételat, Citation2013; Choi et al., Citation2023; Oguchi et al., Citation2017; Wilson et al., Citation1999), and Aβ level increases in AD patients. Nevertheless, Aβ and tauopathy are two major biomarkers of AD and both could be interrelated (Henrı́quez et al., Citation2020; Ittner et al., Citation2010). NaB significantly reduced APP, BACE, and Aβ in TNF-α-induced SH-SY5Y cells.
We have previously illustrated the neuroprotective role of NaB in vitro and in vivo (Bayazid, Kim, et al., Citation2022). Butyrate is a postbiotic, found in the gut from the fermentation of undigested carbohydrates. NaB is considered a histone deacetylase (HDAC) inhibitor and histone acetyltransferase (HAT) agonist, that regulates cell survivability. Thus, NaB showed neuroprotective effects and NaB restored the cytotoxicity by Aβ. NaB also downregulated pro-apoptotic regulator P-53 and cleaved caspase 1 in Aβ-induced SH-SY5Y cells. Moreover, NaB also restored the APP and BACE signalling pathway by aggregated Aβ stimulation (Koh et al., Citation2018; Lee et al., Citation2018; Pang et al., Citation2022) and butyrate has been reported as negatively correlated with Aβ in the clinical studies (Marizzoni et al., Citation2020; Nagpal et al., Citation2019).
NaB (1 mM) treatment ameliorated cell viability at different concentrations of aggregated Aβ-induced SH-SY5Y cells. Aggregated Aβ also changed the cell morphology in light microscopy images and NaB alleviated the cell morphology. Moreover, NaB restored NO in different concentrations and inflammatory markers of aggregated Aβ-induced SH-SY5Y cells. Aggregated Aβ stimulated upregulated pro-apoptotic regulators and APP/BACE signalling pathway, whereas NaB showed remarkable alleviation of the apoptotic regulators and APP/BACE expressions. NaB also restored excessive ROS production by upregulated Nrf-2/HO-1 in Aβ-induced SH-SY5Y cells. Altogether, our data suggest NaB could be a potential therapeutic agent against AD.
Authors contributions
A. B. Bayazid designed the study, conducted experiments, and wrote the manuscript. A. B. Bayazid, Y. H. Jeong, and S. -A. Jeong reviewed the manuscript. B. O. Lim reviewed and supervised the study. All authors have read and agreed to the published version of the manuscript.
Supplemental Material
Download JPEG Image (54.6 KB)Acknowledgements
This paper was written as part of Konkuk University's research support program for its faculty on sabbatical leave in 2022.
Disclosure statement
No potential conflict of interest was reported by the author(s).
Additional information
Funding
References
- Al Mijan, M., Kim, J. Y., Moon, S.-Y., Choi, S.-H., Nah, S.-Y., & Yang, H.-J. (2019). Gintonin enhances proliferation, late stage differentiation, and cell survival from endoplasmic reticulum stress of oligodendrocyte lineage cells. Frontiers in Pharmacology, 10, 1211. https://doi.org/10.3389/fphar.2019.01211
- Alpino, G. D. C. Á., Pereira-Sol, G. A., Dias, M. D. M. E., Aguiar, A. S. D., & Peluzio, M. D. C. G. (2022). Beneficial effects of butyrate on brain functions: A view of epigenetic. Critical Reviews in Food Science and Nutrition, 1–10. https://doi.org/10.1080/10408398.2022.2137776
- Awada, R., Parimisetty, A., & Lefebvre d’Hellencourt, C. (2013). Influence of obesity on neurodegenerative diseases. In U. Kishore (Ed.), Neurodegenerative diseases (pp. 381–401). IntechOpen.
- Bayazid, A. B., & Jang, Y. A. (2021). The role of andrographolide on skin inflammations and modulation of skin barrier functions in human keratinocyte. Biotechnology and Bioprocess Engineering, 26(5), 804–813. https://doi.org/10.1007/s12257-020-0289-x
- Bayazid, A. B., Jang, Y. A., Kim, Y. M., Kim, J. G., & Lim, B. O. (2021). Neuroprotective effects of sodium butyrate through suppressing neuroinflammation and modulating antioxidant enzymes. Neurochemical Research, 46(9), 2348–2358. https://doi.org/10.1007/s11064-021-03369-z
- Bayazid, A. B., Jeong, S. A., Park, C. W., Kim, D. H., & Lim, B. O. (2022). The anti-inflammatory activities of fermented curcuma that contains butyrate mitigate DSS-induced colitis in mice. Molecules, 27(15), 4745. https://doi.org/10.3390/molecules27154745
- Bayazid, A. B., Kim, J. G., Azam, S., Jeong, S. A., Kim, D. H., Park, C. W., & Lim, B. O. (2022). Sodium butyrate ameliorates neurotoxicity and exerts anti-inflammatory effects in high fat diet-fed mice. Food and Chemical Toxicology, 159, 112743. https://doi.org/10.1016/j.fct.2021.112743
- Bayazid, A. B., & Lim, B. O. (2022). Quercetin is an active agent in berries against neurodegenerative diseases progression through modulation of Nrf2/HO1. Nutrients, 14(23), 5132. https://doi.org/10.3390/nu14235132
- Bayazid, A. B., Park, C., Jeong, S., Jang, Y., Lee, J., & Lim, B. (2022). FB alleviates fine dust-induced inflammation in SH-SY5Y cells and improves cognitive impairment of intranasal instillation of PM2.5 in mice. The Korean Society of Medicinal Crop Science.
- Bellenguez, C., Küçükali, F., Jansen, I. E., Kleineidam, L., Moreno-Grau, S., Amin, N., Naj, A. C., Campos-Martin, R., Grenier-Boley, B., Andrade, V., Holmans, P. A., Boland, A., Damotte, V., van der Lee, S. J., Costa, M. R., Kuulasmaa, T., Yang, Q., de Rojas, I., Bis, J. C., … Sánchez-Arjona, M. B. (2022). New insights into the genetic etiology of Alzheimer’s disease and related dementias. Nature Genetics, 54(4), 412–436. https://doi.org/10.1038/s41588-022-01024-z
- Carmona, S., Zahs, K., Wu, E., Dakin, K., Bras, J., & Guerreiro, R. (2018). The role of TREM2 in Alzheimer’s disease and other neurodegenerative disorders. The Lancet Neurology, 17(8), 721–730. https://doi.org/10.1016/S1474-4422(18)30232-1
- Chételat, G. (2013). Aβ-independent processes – Rethinking preclinical AD. Nature Reviews Neurology, 9(3), 123–124. https://doi.org/10.1038/nrneurol.2013.21
- Choi, I., Wang, M., Yoo, S., Xu, P., Seegobin, S. P., Li, X., Han, X., Wang, Q., Peng, J., Zhang, B., & Yue, Z. (2023). Autophagy enables microglia to engage amyloid plaques and prevents microglial senescence. Nature Cell Biology, 25(7), 963–974. https://doi.org/10.1038/s41556-023-01158-0
- Dong, Y., & Cui, C. (2022). The role of short-chain fatty acids in central nervous system diseases. Molecular and Cellular Biochemistry, 477(11), 2595–2607. https://doi.org/10.1007/s11010-022-04471-8
- Fernandes, F., Barbosa, M., Pereira, D. M., Sousa-Pinto, I., Valentão, P., Azevedo, I. C., & Andrade, P. B. (2018). Chemical profiling of edible seaweed (Ochrophyta) extracts and assessment of their in vitro effects on cell-free enzyme systems and on the viability of glutamate-injured SH-SY5Y cells. Food and Chemical Toxicology, 116, 196–206. https://doi.org/10.1016/j.fct.2018.04.033
- Gratuze, M., Leyns, C. E. G., & Holtzman, D. M. (2018). New insights into the role of TREM2 in Alzheimer’s disease. Molecular Neurodegeneration, 13(1), 66. https://doi.org/10.1186/s13024-018-0298-9
- Hamilton, A., Vasefi, M., Vander Tuin, C., McQuaid, R. J., Anisman, H., & Ferguson, S. S. G. (2016). Chronic pharmacological mGluR5 inhibition prevents cognitive impairment and reduces pathogenesis in an Alzheimer disease mouse model. Cell Reports, 15(9), 1859–1865. https://doi.org/10.1016/j.celrep.2016.04.077
- Heneka, M. T., Carson, M. J., Khoury, J. E., Landreth, G. E., Brosseron, F., Feinstein, D. L., Jacobs, A. H., Wyss-Coray, T., Vitorica, J., Ransohoff, R. M., Herrup, K., Frautschy, S. A., Finsen, B., Brown, G. C., Verkhratsky, A., Yamanaka, K., Koistinaho, J., Latz, E., Halle, A., … Kummer, M. P. (2015). Neuroinflammation in Alzheimer’s disease. The Lancet Neurology, 14(4), 388–405. https://doi.org/10.1016/S1474-4422(15)70016-5
- Henrı́quez, G., Mendez, L., Varela-Ramirez, A., Guerrero, E., & Narayan, M. (2020). Neuroprotective effect of Brazilin on amyloid β (25–35)-induced pathology in a human neuroblastoma model. ACS Omega, 5(23), 13785–13792. https://doi.org/10.1021/acsomega.0c00396
- Ittner, L. M., Ke, Y. D., Delerue, F., Bi, M., Gladbach, A., van Eersel, J., Wölfing, H., Chieng, B. C., Christie, M. J., Napier, I. A., Eckert, A., Staufenbiel, M., Hardeman, E., & Götz, J. (2010). Dendritic function of tau mediates amyloid-β toxicity in Alzheimer’s disease mouse models. Cell, 142(3), 387–397. https://doi.org/10.1016/j.cell.2010.06.036
- Jaradat, N., Abualhasan, M., Hawash, M., Qadi, M., Al-Maharik, N., Abdallah, S., Mousa, A., Zarour, A., Arar, M., Sobuh, S., Hussein, F., Issa, L., Jaber, A., Hamduni, H., & Alshahatit, S. (2023). Chromatography analysis, in light of vitro antioxidant, antidiabetic, antiobesity, anti-inflammatory, antimicrobial, anticancer, and three-dimensional cancer spheroids’ formation blocking activities of Laurus nobilis aromatic oil from Palestine. Chemical and Biological Technologies in Agriculture, 10(1), 25. https://doi.org/10.1186/s40538-023-00396-6
- Jia, G., Diao, Z., Liu, Y., Sun, C., & Wang, C. (2021). Neural stem cell-conditioned medium ameliorates Aβ25–35-induced damage in SH-SY5Y cells by protecting mitochondrial function. Bosnian Journal of Basic Medical Sciences, 21(2), 179–186. https://doi.org/10.17305/bjbms.2020.4570
- Karaahmet, B., Le, L., Mendes, M. S., Majewska, A. K., & O’Banion, M. K. (2022). Repopulated microglia induce expression of Cxcl13 with differential changes in tau phosphorylation but do not impact amyloid pathology. Journal of Neuroinflammation, 19(1), 173. https://doi.org/10.1186/s12974-022-02532-9
- Koh, E.-J., Kim, K.-J., Choi, J., Kang, D.-H., & Lee, B.-Y. (2018). Spirulina maxima extract prevents cell death through BDNF activation against amyloid beta 1–42 (Aβ1–42) induced neurotoxicity in PC12 cells. Neuroscience Letters, 673, 33–38. https://doi.org/10.1016/j.neulet.2018.02.057
- Lee, A. Y., Lee, M.-H., Lee, S., & Cho, E. J. (2018). Alpha-linolenic acid regulates amyloid precursor protein processing by mitogen-activated protein kinase pathway and neuronal apoptosis in amyloid beta-induced SH-SY5Y neuronal cells. Applied Biological Chemistry, 61(1), 61–71. https://doi.org/10.1007/s13765-017-0334-4
- Lee, J.-H., Yang, D.-S., Goulbourne, C. N., Im, E., Stavrides, P., Pensalfini, A., Chan, H., Bouchet-Marquis, C., Bleiwas, C., Berg, M. J., Huo, C., Peddy, J., Pawlik, M., Levy, E., Rao, M., Staufenbiel, M., & Nixon, R. A. (2022). Faulty autolysosome acidification in Alzheimer’s disease mouse models induces autophagic build-up of Aβ in neurons, yielding senile plaques. Nature Neuroscience, 25(6), 688–701. https://doi.org/10.1038/s41593-022-01084-8
- Lin, C.-I., Chang, Y.-C., Kao, N.-J., Lee, W.-J., Cross, T.-W., & Lin, S.-H. (2020). 1,25(OH)2D3 alleviates Aβ(25–35)-induced tau hyperphosphorylation, excessive reactive oxygen species, and apoptosis through interplay with glial cell line-derived neurotrophic factor signaling in SH-SY5Y cells. International Journal of Molecular Sciences, 21(12), 4215. https://doi.org/10.3390/ijms21124215
- Long, J. M., & Holtzman, D. M. (2019). Alzheimer disease: An update on pathobiology and treatment strategies. Cell, 179(2), 312–339. https://doi.org/10.1016/j.cell.2019.09.001
- Luo, Q.-J., Sun, M.-X., Guo, Y.-W., Tan, S.-W., Wu, X.-Y., Abassa, K.-K., Lin, L., Liu, H.-L., Jiang, J., & Wei, X.-Q. (2020). Sodium butyrate protects against lipopolysaccharide-induced liver injury partially via the GPR43/β-arrestin-2/NF-κB network. Gastroenterology Report, 9(2), 154–165. https://doi.org/10.1093/gastro/goaa085
- Marizzoni, M., Cattaneo, A., Mirabelli, P., Festari, C., Lopizzo, N., Nicolosi, V., Mombelli, E., Mazzelli, M., Luongo, D., Naviglio, D., Coppola, L., Salvatore, M., & Frisoni, G. B. (2020). Short-chain fatty acids and lipopolysaccharide as mediators between gut dysbiosis and amyloid pathology in Alzheimer’s disease. Journal of Alzheimer’s Disease, 78(2), 683–697. https://doi.org/10.3233/JAD-200306
- Milà-Alomà, M., Ashton, N. J., Shekari, M., Salvadó, G., Ortiz-Romero, P., Montoliu-Gaya, L., Benedet, A. L., Karikari, T. K., Lantero-Rodriguez, J., Vanmechelen, E., Day, T. A., González-Escalante, A., Sánchez-Benavides, G., Minguillon, C., Fauria, K., Molinuevo, J. L., Dage, J. L., Zetterberg, H., Gispert, J. D., … Blennow, K. (2022). Plasma p-tau231 and p-tau217 as state markers of amyloid-β pathology in preclinical Alzheimer’s disease. Nature Medicine, 28(9), 1797–1801. https://doi.org/10.1038/s41591-022-01925-w
- Mirzaei, R., Bouzari, B., Hosseini-Fard, S. R., Mazaheri, M., Ahmadyousefi, Y., Abdi, M., Jalalifar, S., Karimitabar, Z., Teimoori, A., Keyvani, H., Zamani, F., Yousefimashouf, R., & Karampoor, S. (2021). Role of microbiota-derived short-chain fatty acids in nervous system disorders. Biomedicine & Pharmacotherapy, 139, 111661. https://doi.org/10.1016/j.biopha.2021.111661
- Nagpal, R., Neth, B. J., Wang, S., Craft, S., & Yadav, H. (2019). Modified Mediterranean-ketogenic diet modulates gut microbiome and short-chain fatty acids in association with Alzheimer’s disease markers in subjects with mild cognitive impairment. EBioMedicine, 47, 529–542. https://doi.org/10.1016/j.ebiom.2019.08.032
- Oguchi, T., Ono, R., Tsuji, M., Shozawa, H., Somei, M., Inagaki, M., Mori, Y., Yasumoto, T., Ono, K., & Kiuchi, Y. (2017). Cilostazol suppresses Aβ-induced neurotoxicity in SH-SY5Y cells through inhibition of oxidative stress and MAPK signaling pathway. Frontiers in Aging Neuroscience, 9, 337. https://doi.org/10.3389/fnagi.2017.00337
- Pang, K., Jiang, R., Zhang, W., Yang, Z., Li, L.-L., Shimozawa, M., Tambaro, S., Mayer, J., Zhang, B., Li, M., Wang, J., Liu, H., Yang, A., Chen, X., Liu, J., Winblad, B., Han, H., Jiang, T., Wang, W., … Lu, B. (2022). An App knock-in rat model for Alzheimer’s disease exhibiting Aβ and tau pathologies, neuronal death and cognitive impairments. Cell Research, 32(2), 157–175. https://doi.org/10.1038/s41422-021-00582-x
- Qiu, C., Kivipelto, M., & von Strauss, E. (2009). Epidemiology of Alzheimer’s disease: Occurrence, determinants, and strategies toward intervention. Dialogues in Clinical Neuroscience, 11(2), 111–128. https://doi.org/10.31887/DCNS.2009.11.2/cqiu
- Radi, E., Formichi, P., Battisti, C., & Federico, A. (2014). Apoptosis and oxidative stress in neurodegenerative diseases. Journal of Alzheimer’s Disease, 42(Suppl. 3), S125–S152. https://doi.org/10.3233/JAD-132738
- Riegerová, P., Brejcha, J., Bezděková, D., Chum, T., Mašínová, E., Čermáková, N., Ovsepian, S. V., Cebecauer, M., & Štefl, M. (2021). Expression and localization of AβPP in SH-SY5Y cells depends on differentiation state. Journal of Alzheimer’s Disease, 82(2), 485–491. https://doi.org/10.3233/JAD-201409
- Wilson, C. A., Doms, R. W., & Lee, V. M.-Y. (1999). Intracellular APP processing and Aβ production in Alzheimer disease. Journal of Neuropathology & Experimental Neurology, 58(8), 787–794. https://doi.org/10.1097/00005072-199908000-00001