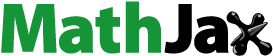
ABSTRACT
This study employed untargeted metabolomics to reveal the changes in the metabolic profile of walnut milk under two different processing methods. The walnut milk of the control group was not treated, which was fermented through L. plantarum in F group, while the walnut milk after proteolysis was fermented in EF group. The results showed that fermentation gradually reduced the pH of walnut milk to 4.70 and 3.61 in F and EF group respectively, for viable count which are 2.9 × 109 CFU/mL and 9.3 × 109 CFU/mL. The antioxidant activity of EF group is significantly higher than Con group (p < 0.05). Through UHPLC-Q-TOF MS and multivariate statistical analysis, the identified metabolites were mainly lipids and lipid-like molecules, as well as organic acids and derivatives. Compared with fermented walnut milk, fermentation after proteolysis significantly increased the functional metabolites contained in walnut milk which are included in antibacterial, antioxidant, anti-inflammatory.
1. Introduction
Given health issues including lactose intolerance, the market for wide range of plant milk substitutes, which include legumes, cereals, nuts, has been developing lately (Mäkinen et al., Citation2016). There is a tendency toward foods and beverages with clean tags (Tangyu et al., Citation2019). Natural plant-based milk replacement that replicate the food value and quality of milk produced by animals without integrating are of special interest which can be realized through the fermentation process (Asioli et al., Citation2017). As a functional food, walnut provide a variety of nutrients that humans need, including 14–17% high-quality protein with a digestibility of 87.2% (He et al., Citation2010; Wang et al., Citation2021b). An abundance of bioactive materials, including protein, unsaturated fatty acid, plant sterol, polyphenol, dietary fiber and others, have been found in walnuts in previous studies (Ni et al., Citation2022; Zhong et al., Citation2018). Walnuts are said to have many positive effects, including lowering the incidence of coronary heart disease and acting as an antiatherogen as well as having anti-inflammatory, antioxidant, and other biological activities (Bulló et al., Citation2010; Feldman, Citation2002; Grosso et al., Citation2020; Oliveira et al., Citation2008; Ros, Citation2010; Spence et al., Citation2023). At present, walnut-related fermented products on the market mainly include walnut milk mixed and walnut soy sauce, and research has shown that they have beneficial biological activity (İlyasoğlu & Yilmaz, Citation2019; Mao et al., Citation2022; Xu et al., Citation2020).
The widespread employ of lactic acid bacteria in food fermentation is universally acknowledged to be safe (GRAS-designated safe strains) (Das et al., Citation2017). Probiotics are bioactive dietary alternative treatments that support the host by preserving their natural intestinal bacteria, which have many health benefits for the host include regulation of serum cholesterol level, immune system improvement, and so on (Jayarathna et al., Citation2021; Wang, Zhang, et al., Citation2021). The addition of the fermentation process could lessen unpleasant flavors like beany and help to produce aroma in fermented food through further biotransformation (Bartkiene et al., Citation2015; Xiang et al., Citation2019; Yi et al., Citation2021). Moreover, fermentation has the capacity to boost the amounts of beneficial metabolites in order to create a clean-label fermented dairy alternative that is sufficiently appealing to consumers (Pua et al., Citation2022). Past research on the fatty acid composition of walnuts revealed that the fat content spans between 52% and 70%, and that approximately 90% of the total fatty acids in walnuts are unsaturated (Li et al., Citation2017), so the strain of L. plantarum selected by our laboratory has been found to have a strong fat decomposition ability through preliminary research, and can be well adapted to fermentation using walnut pulp as a substrate (Fiorino et al., Citation2023). Liu et al. fermented walnut milk with L. plantarum LP56 and added appropriate sucrose and emulsifiers,which showed that mild fermentation promoted the production of amino acids and fatty acids, which had a beneficial impact on walnut milk products (Liu et al., Citation2022).
Enzymatic hydrolysis technology can be more beneficial to the fermentation process. Protease treatment prior to fermentation for plant-based milk can digest protein to amino acids or peptides, which could speed up the development and metabolic process of lactic acid bacteria, increase the degree of substrate hydrolysis to improve the rate of raw material utilization, the speed of fermentation, the rich flavor of product, and also be advantageous for digestion and absorption (Ma et al., Citation2013; Yarlina et al., Citation2020). Now, several publications have discussed the technology of proteolysis before fermentation in relation to lupin protein, pea protein, soybean protein, and other food, but which they have not discussed in relation to walnut milk (García Arteaga et al., Citation2022; Meinlschmidt, Citation2016; Schlegel et al., Citation2021; Wang et al., Citation2020; Yang et al., Citation2020). In addition, Mao et al. found that the conversion of CLA and CLNA in walnut milk fermented by B. breve CCFM683 can be achieved by the addition of lipase (Mao et al., Citation2022).
In order to track metabolite changes throughout fermentation and assess the biological properties of the finished fermented food, metabolite profiling in which is performed (Yang et al., Citation2020). Fermentation is a complicated process that involves changing substrates through microbial activity (Adebo et al., Citation2017; Adebo & Gabriela Medina-Meza, Citation2020). Typical plant-based milk metabolites may include volatile compounds, amino acids, small peptides, fatty acids and some secondary metabolites depending on the substrate of fermentation (Park et al., Citation2019b; Verbeke et al., Citation2015). The use of metabolomics to identify and analyse small-molecule metabolites generated by microorganisms at a particular time under a specific climatic parameter has become a crucial instrument for gathering qualitative and quantitative profiling of biological systems if traditional techniques have some limitations such as it used to analyse a complex and multidimensional metabolic space (Li et al., Citation2020). When conducting comparative study, metabolomic investigations are important in terms of the comparative change in metabolite profusion (Das et al., Citation2017). According to numerous researches, metabolomics has been used extensively in the mechanism analysis of fermentation with plant-based milk such as soybean, oat, buckthorn juice, red sour soup (Bocchi et al., Citation2021; Mozzi et al., Citation2013; Shudong et al., Citation2022; Zhou et al., Citation2022). The potential of this metabolomics technology to enlighten the metabolome geographies of fermented product is illustrated by the rising utilize of metabolomics in the studies of food fermentation (Adebo et al., Citation2021).
But so far, the metabolic characteristics of the strain in the duration of walnut milk have not been clarified. In this research, walnut milk was developed using two different processing methods (F and EF group, walnut milk was fermented and walnut milk after proteolysis was fermented), and UHPLC-Q-TOF MS was used for untargeted metabolomic analysis to deeply explore the fermentation mechanism, in order to deepen our understanding of the process of fermented plant-based walnut milk. Potential mechanisms of beneficial metabolites production which has far-reaching significance for the functional investigation and development and industrialization of walnut high real worth products.
2. Materials and methods
2.1. Materials
Walnut (juglans regia cv.lvlin) was obtained from Hebei Yangyuan Zhihui Beverage Co. Ltd.; Papain (200 U/mg) was gained from Biotopped. (Beijing, China); The reagents used in this report were all purchased from Solabio Co. (Beijing, China) and were analytically pure; L. plantarum is obtained from laboratory.
2.2. L. plantarum culture conditions
In MRS medium broth, L. plantarum was further revived for 12 h under anaerobic incubation under stationary conditions at 37°C. After being revived, 2% (v/v) culture broth expanded for 10 h until it met the vitality standard (OD = 2.0). The bacterial sludge was centrifuged at 8000 r/min for 5 min, and then it was resuspended in 0.9% NaCl to serve as an inoculum for fermentation tests.
2.3. Preparation of samples
To create pure walnut slurry, walnut is peeled, crushed, powdered, and homogenized. The slurry was separated into 50 mL portions and pasteurized at 95°C for 10 min in conical flasks. After that, walnut milk was chilled to ambient temperature and kept at 4°C for later research, which was used as control called Con group.
The hegemonic L. plantarum with a live bacterial population of 108 CFU/mL were introduced to Con group and incubated for 24 h at 37°C in an incubator, which was called F group.
Add 3% (w/w) papain to Con group, The hydrolysis was maintained at 50°C for 2 h with constant stirring (150 rpm) in water bath. After pasteurization and natural cooling, the samples are kept at 4°C in the refrigerator and prepared for further testing. And the hegemonic L. plantarum with a live bacterial population of 108 CFU/mL were introduced and incubated for 24 h at 37 °C in an incubator, which was called EF group.
Samples were obtained every 2 h through fermentation process for microbiological bacterial and phsicochemical analysis. These samples mentioned above were held at −80°C until the additional analysis.
2.4. Examination of bacterial development throughout fermentation
Observe the development curve of live bacteria in F and EF group through plate dilution on MRS agar to measure the increase of L. plantarum. The units were calculated in colonies that formed per milliliter (CFU/mL). Total dishes were cultured aerobic conditions at 37°C for 48 ± 2 h.
2.5. Variation in physicochemical properties during fermentation
2.5.1. pH
Every 2 h while the samples were fermenting, the pH was measured. Using buffer solutions with pH values of 4 and 7 at room temperature, a digital pH meter (HI99163, HANNA, Woonsocket, USA) was calibrated before being used to measure the pH of test samples.
2.5.2. The degree of hydrolysis (DH)
The degree of hydrolysis (DH) of samples was determined by o-phthaldialdehyde (OPA) spectrophotometric method (García Arteaga et al., Citation2022). After the protein solution and OPA reagent reacted in the darkness, the fluorescence was measured at 340 nm using a spectrophotometer. The DH was determined by calculating according to the concerning equations:
(1)
(1) Serine-NH2 = meqvserine-NH2/g protein; Abssample = sample spectrophotometer value; Absblank = blank spectrophotometer value; Absstandard = standard spectrophotometer value; Vsample = volume of sample solution (L); msample = quantity of sample (g); and PCsample = protein content of sample (%).
(2)
(2) h = amount of hydrolyzed peptide bonds; β = linear regression is used to calibrate the slope; and α = degree of dissociation of the α-amino group;
(3)
(3) DH = degree of hydrolysis (%); htot = number of equivalent peptide bonds in all protein.
2.5.3. Analysis of basic components during fermentation
Proximate composition was determined in accordance with AOAC methods (2006) (İlyasoğlu & Yilmaz, Citation2019). The content of total protein is calculated by Kjeldahl method according to AOAC Official Method 950.48 as described earlier, which was calculated from protein nitrogen using appropriate conversion factors (Sathe et al., Citation2009). The content of total moisture is calculated by Direct drying method.
2.6. Antioxidant measurements
2.6.1. Free radical-scavenging assay
The effect of walnut milk on DPPH radicals was evaluated using the modified method by Shyu et al. (Wang et al., Citation2014). 800 μL of the 0.4 M DPPH solution in methanol was prepared, and different concentrations of this solution were added to 800 μL test samples. The reaction mixture was thoroughly shaken and allowed to sit at room temperature for 30 min before the absorbance of the resulting solution was measured at 517 nm in comparison to a control. In order to create a standard curve, several Vc concentrations were used.
2.6.2. ABTS scavenging activity
The spectrophotometric approach known as the ABTS radical cation decolorization test is commonly employed to assess the antioxidant activity of various compounds (Sandhu et al., Citation2016). ABTS (2,2’-azino-bis(3-ethylbenzothiazoline-6-sulphonic acid)) assay: ABTS radical scavenging capacity was determined according to Shumoy et al. with modified (Shumoy et al., Citation2019). In a disposable opening, 30 μL of extract was combined with 3 mL of ABTS cation solution. After 5 min of incubation, the reduction in absorbance was determined. In order to create a standard curve, several Vc concentrations were used.
2.7. UHPLC–MS/MS conditions for metabolome analysis
Methanol/acetonitrile/H2O (2:2:1, v/v) were added to the homogenized solution for metabolite extraction after the sample was gradually thawed at 4°C. The mixture was centrifuged at 14,000 × g for 20 min at 4°C. Vacuum centrifugation was used to dry the supernatant. The samples were redissolved in 100 μL of acetonitrile/H2O (1:1, v/v) solvent and centrifuged at 14,000 × g for 15 min at 4°C before the supernatant was injected for LC-MS analysis.
The samples were analysed using an UHPLC (1290 Infinity LC, Agilent Technologies) coupled to a quadrupole time-of-flight (AB Sciex TripleTOF 6600) in Shanghai Applied Protein Technology Co., Ltd. The injection volume was 2 μL per sample. An ACQUIY UPLC BEH Amide 1.7 µm column measuring 2.1 mm × 100 mm was used to examine the samples. The mobile phase contains A: 25 mM ammonium acetate with 25 mM ammonium hydroxide in H2O; B: acetonitrile which all in both modes of ESI+ and ESI-. The gradient was 95% B for 0.5 min and was linearly reduced to 65% in 6.5 min, and then was reduced to 40% in 1 min and kept for 1 min, and then increased to 95% in 0.1 min, with a 3 min re-equilibration period employed. Quality control (QC) samples were checked by aggregating 10 μL of every sample and analyzing them alongside the other samples in order to check the reliability and reproducibility of the instrument analysis. The QC samples were put in and checked at every five samples. The ESI source was tuned to the following parameters: 600 °C for the source temperature, ±5500 V for ion Spray Voltage Floating (ISVF), 60 for each of the ion source gases (Gas1 and Gas2), and 30 for the curtain gas (CUR). With a m/z range of 60–1000 Da and an accumulation time for TOF-MS scans of 0.20 s/spectra, the apparatus was equipped for MS-only acquisition. The system was installed for automated MS/MS gathering with a m/z range of 25–1000 Da and the product ion scan accumulation time of 0.05 s/spectra. With information dependent acquisition (IDA), the highly accurate parameter is chosen to obtain the product ion scan. The high precision selection is utilized to acquire the product ion scan using information dependent acquisition (IDA). The following guidelines were created: exclude isotopes below 4 Da; 60 V (+) and −60 V (−) are declustering potential (DP); testing every cycle with ions: 10; fixed at 35 V ± 15 eV was the collision energy (CE).
2.8. Metabolic pathway enrichment analysis
The metabolic pathways involved in several activities of walnut milk were examined using the KEGG pathway database(http://www.kegg.jp/kegg/pathway.html). KEGG stands for Kyoto Encyclopedia of Genes and Genomes. The significantly different metabolites discovered in the walnut milk samples were matched to the KEGG pathways which were then enriched and assessed, and the metabolic pathways with the highest connection with differential metabolites were discovered.
2.9. Statistical analysis
The generated data were subjected to multivariate data analysis using the R package (ropls), which included principal component analysis (PCA). Response permutation testing and seven-fold cross-validation were used to assess the resilience of the model. It was evaluated whether differences between distinctions among two groups of independent samples were significant using the Student's t-test. The screening thresholds for noteworthy altered metabolites were VIP > 1 and p < 0.05. To ascertain the association between two variables, Pearson's correlation analysis was used. Three samples were taken and discussed separately with each estimation, and then getting the average, standardizing deviation and error values where the estimated records were averaged. The chi-square test was used to get the p-value in order to confirm the statistical significance of the data collected.
3. Results and discussion
3.1. Bacterial development during fermentation
The development pattern of L. plantarum in samples of walnut milk was examined every few hours up to 24 h through the process of fermentation, and two development curves were produced among the incubation duration and the recorded cell number in connection to CFU/mL which is shown as (a). Viable count of 2.9 × 109 CFU/mL and 9.3 × 109 CFU/mL sample were determined in F and EF group respectively. At 12 h of incubation, L. plantarum reached its largest increase (based on the greatest CFU/mL measurement), and its population remained constant for the following 12–18 h. Over the whole fermentation process, soymilk showed almost identical development patterns which has an explosive growth graph with lag, log, stationary, and decline phases was seen (Sharma et al., Citation2021). After achieving their highest yield, the number of cells started to decrease as a result of inadequate intake such as protein and lipids, particularly in the EF group. A key metric that might connect to the ability of probiotics to promote health was their viable count. To ensure that ingesting functional dairy products has a health-promoting effect, it would be important to consume enough probiotics (Zha et al., Citation2021). For instance, it was recommended that the level should be greater than 106 CFU/mL during consumption (Lourens-Hattingh & Viljoen, Citation2001). Our research showed that L. plantarum remained abundant in walnut milk even after fermentation, indicating that it met the criteria for a possible effective probiotic strain.
Figure 1. (a) Charts illustrating shifts in cell counts (CFU/mL) and pH-value during fermentation using L. plantarum in F group and EF group. The findings are provided as mean ± standard deviation (SD) of observations made in triplicate (n = 3). (b) Changes in antioxidant activities (Free radical-scavenging assay) with Con, F and EF group. 0.2 g/mL Vc as positive control. (c) Changes in antioxidant activities (ABTS scavenging activity) with Con, F and EF groups. 0.2 g/mL Vc as positive control.

3.2. Variation in physicochemical properties during fermentation
Changes in the pH-value of F and EF group during fermentation was shown in (a). Early through the fermentation procedure, the pH value of F and EF groups were near neutral, the pH of EF group reached the dropped rapidly until the fermentation endpoint of pH 3.61, when the fermentation endpoint of F group is 4.70, which has a similar curve with EF group. The pH of the F and EF groups dropped fast in the first 8 h of fermentation, which may have been caused by the buildup of organic acids (Yang et al., Citation2022). Similar to other research where milk was fermented by L. plantarum P-8 (Dan et al., Citation2019), the pH values of the groups supplied with L. plantarum changed during fermentation. The degree of hydrolysis of walnut milk hydrolysates were measured by 2.6.3 which is 18.18%. Amino acids and peptides found in protein hydrolysates have the potential to enhance microbial growth, fermentation potential, and environmental tolerance (Li et al., Citation2021). It is speculated that the process of proteolysis can accelerate the growth and metabolism of L. plantarum, which can reduce pH even more.
3.3. Analysis of basic components during fermentation
From , there was no significant difference in protein and moisture between the F and EF groups, and both met national standards.
Table 1. Basic components.
3.4. Analysis of antioxidant capacity
3.4.1. Free radical-scavenging assay
Three sets of walnut milk (stock solution and gradient diluted samples) had their DPPH free radical scavenging rates analysed. The findings are visualized in (b). Only the EF group showed a noticeable improvement when compared to the Con group in terms of the DPPH free radical scavenging ability of the fermented walnut milk made using the two procedures (p < 0.05). The DPPH free radical scavenging ability of EF group can be as high as 94.92%, which is higher than Con group 81.54% (p < 0.05). Both methods produce fermented walnut milk that has a rate of DPPH free radical scavenging, which may have a potential positive effect on the functional activity of product. The antioxidant activity of fermented cabbage is demonstrably larger than that of untreated cabbage, according to research by Dase Hunaefi et al (Hunaefi et al., Citation2013). In addition, lactic acid bacteria are not only able to produce antioxidant metabolites using walnut milk matrix, but also have antioxidant activity themselves, which contain antioxidant mechanisms that are both enzymatic and non-enzymatic and lower the production of reactive oxygen species to a level that is safe for cells (Hur et al., Citation2014).
3.4.2. ABTS scavenging activity
Both lipophilic and hydrophilic antioxidants can be tested through using ABTS free radical cationic decolorization assay; the evaluation outcomes are shown in the picture (c). The relative % of ABTS free radical clearance in the F and EF group obviously increased in comparison to the control group (p < 0.05). The ABTS free radical scavenging ability of F and EF group can be as high as 47.37% and 90.99%, higher than Con group which is 43.34% (p < 0.05). It is suspected that this may be because L. plantarum produces more and different types of advantageous metabolites as a result of its improved proteolytic impact, which offers good nutrients for the bacteria's growth and utilization (Zhang et al., Citation2021). It can enhance their antioxidant activity in vitro. Due to differences in the mechanism of action, which could account for the variations and unsteady changes between the antioxidant capacity assays used in the current investigation (Ozcan et al., Citation2019). But from the results, it is still the EF group that has a more significant positive effect on product.
3.5. UHPLC–MS/MS conditions for metabolome analysis
3.5.1. Multidimensional statistical analysis of the metabolite profiles in Con, F and EF group
Fermented walnut milk contained 1821 metabolites in total, of which 1171 and 650 were identified in the ESI+ and ESI- modes, respectively which were grouped into 12 categories. As shown in (a). A hierarchical cluster analysis (HCA) was conducted to further expose the dynamic changes in the metabolites of groups, and the findings are displayed in Figure A1. The rows show the identical metabolites in each of the three groups of walnut milk represented by the columns. Red to blue, or high to low, color-coded scales are used to display the relative amount of metabolites. The six repeats of each group were grouped together, demonstrating good homogeneity, high dependability, and repeatability of the repetitions.
Figure 2. (a) Categorization of all metabolites (b) Principal Component Analysis (PCA) of metabolites with significant differences under the combination of ESI+ and ESI− modes (p < 0.05, VIP > 1). (c) Venn for the differential metabolites of three groups (Con, F and EF groups) by ESI+ and ESI− modes (p < 0.05, VIP > 1). Control group: walnut milk without proteolysis and fermentation; F group: Control sample treated with fermentation; and EF group: Control sample following proteolysis treated with fermentation.

PCA score plots of the L. plantarum-produced fermented walnut milk metabolomes. PCA was used to examine the variations in the walnut milk metabolome that occurred during two different processes. Datasets at the termination of fermentation, as well as fermentation of walnut milk following proteolysis, were analysed. Samples from the three different groups were represented by symbols that displayed distinct clustering characteristics and were dispersed around various quadrants ((b)), revealing apparent variations in the metabolite profiles across samples of fermented milk prepared with different processing conditions. Samples were separated into several zones based on the first principal component (PC1) and the second principal component (PC2), indicating the impact of proteolysis and fermentation. PC1 (54.8%) and PC2 (70.7%) combined explained the total variation under the combination of ESI+ and ESI− modes. indicating that these three groups had unique metabolisms that could be accurately used to screen for metabolite variations.
3.5.2. Differential numerous metabolites identified in F and EF group
Based on multidimensional statistical analysis such as PCA, combined with venn plots ((c)), 273 differential metabolites were identified from the modes of ESI+ and ESI- (p < 0.05, VIP > 1) in the comparison of metabolites between Con group and F group, which including 143 up-regulated metabolites (red points) and 130 down-regulated (blue points) metabolites. 581 differential metabolites were identified (p < 0.05, VIP > 1) in the comparison of metabolites between Con group and EF group, which including 464 up-regulated metabolites and 117 down-regulated metabolites like above. Moreover, 597 differential metabolites were identified (p < 0.05, VIP > 1) in the comparison of metabolites between F group and EF group, which including 477 up-regulated metabolites and 90 down-regulated metabolites. They show that fermentation with L. plantarum after proteolysis of walnut milk can further alter the metabolic patterns of walnut milk. It will be explained in detail. These results could be visualized better by volcano plots (Figure A2).
The contents of magnolol and indole-lactic acid in F group risen significantly (p < 0.05) compared with Con group ((a,b)). Magnolol has anti-oxidative, anti-inflammatory, anti-diabetic, anti-microbial and antiviral activity (Chen et al., Citation2011; Kim et al., Citation2021). By the activation of the human hydroxycarboxylic acid receptor 3 (HCA3), indole-lactic acid has been identified to be potentially modulators of human immunological function (Laursen et al., Citation2020). The results demonstrated that the fermentation of pure walnut milk by L. plantarum was able to produce beneficial metabolites that could enrich the quality and potential functionality of walnut-related products. Three metabolites all increased significantly after fermentation, but the results were not significantly affected by enzymatic hydrolysis. It is speculated that it may be due to changes in the fermentation substrate after proteolysis of walnut milk, resulting in degradation and transformation in the production of these metabolites by L. plantarum during growth and metabolism (Ru et al., Citation2022).
Figure 3. (a–s) Box and whisker graphs of a part of differential metabolites in the walnut milk (p < 0.05) (a, b) which in F group were significantly more than those in Con and EF group. (c–k) which in EF group were significantly more than those in F group and F group were significantly more than that in Con group. (l–s) which in EF group were significantly more than those in Con group and F group.

The contents of 13-hydroxy-9z,11e-octadecadienoic acid, 2-hydroxyisocaproic acid, 3-Phenyllactic acid, 4-hydroxyphenyllactic acid, propionic acid, 2-hydroxy-3-methylbutyricacid, 3-hydroxy-4-methoxycinnamic acid, diacetyl and pyruvate increased significantly in F group and more significantly in EF group when compared to Con group (p < 0.05) which is shown as (c–k). Walnut has a high content of poly-unsaturated fatty acids (Čelakovská et al., Citation2021). The most noticeable differentially abundant metabolite was fatty acids because the strain of L. plantarum selected by our laboratory has been found to have a strong fat decomposition ability through preliminary research, and can be well adapted to fermentation using walnut pulp as a substrate. However, lipid lipolysis and subsequent catabolism contribute to the flavor development of fermented foods, and may also lead to the release of biologically active fatty acid derivatives in appropriate circumstances (Ares-Yebra et al., Citation2019). When milk is fermented, fatty acids often develop from the biological processes of lipolysis, proteolysis, or other fermentation (Delgado et al., Citation2010). It is noteworthy that the relative concentrations of 13-hydroxy-9z,11e-octadecadienoic acid, 2-hydroxyisocaproic acid (HICA), 3-Phenyllactic acid (PLA), 4-hydroxyphenyllactic acid are increased significantly after fermentation of pure walnut milk, and is better for fermentation of walnut milk following proteolysis, which are of interest to the food industry because of its anti-bacterial activity in product (Mu et al., Citation2012; Nieminen et al., Citation2014; Sim et al., Citation2015; Zha et al., Citation2021). The expansion of the contents of HICA, PLA and 4-hydroxyphenyllactic acid is in harmony with the research results of Li et al. (Loh et al., Citation2021) on probiotic-fermented unhopped beers. In addition to being used as a food preservative (Yang & Choong, Citation2001). Intestinal epithelial cell proliferation is encouraged the expression of MYC by 2-hydroxy-3-methylbutyric acid through the MAFF/MBP1 pathway, which may aid in intestinal barrier restoration (Qiao et al., Citation2022). 3-hydroxy-4-methoxycinnamic acid has antioxidant activity (Wang et al., Citation2011). Studies have shown that the content of 3-hydroxy-4-methoxycinnamic acid in plant-based fermented milk with high antioxidant activity continues to increase during the fermentation process (Hunaefi et al., Citation2013). It can be inferred that lactic acid bacteria produce a large amount of pyruvate in walnut milk through the pathway of glycolysis. In addition to the substantial amount of lactic acid being generated, pyruvate can also have a significant increase of diacetyl through the pathway of butyrate metabolism. The accumulation of diacetyl derived from microorganisms during fermentation will produce the aroma of cream wine (Mink et al., Citation2014). Pyruvate is also associated with the TCA cycle (Christensen & Pederson, Citation1958), but oxaloacetic acid will participate in the metabolic pathway of amino acids such as alanine, resulting in a significant decrease in the content of fumarate and malate in the TCA cycle (p < 0.05). Previous research state that fermentation often uses short-term fermentation (Bocchi et al., Citation2021), which struggles to hydrolyze biological macromolecules entirely (particularly proteins and lipids) to generate functional compounds, as lactic acid bacteria lack the extensive hydrolyzing steps required for the degradation of target protein (Coda et al., Citation2012). Amino acids and peptides found in abundance in proteolysis have been shown to be potent factors for enhancing microbial growth, fermentation capability, and environmental tolerance (Li et al., Citation2021). The significant increase in the content of these beneficial metabolites mentioned above can show that the process of walnut proteolysis before fermentation can better improve the develpoment and metabolism of L. plantarum and give better play to the role of fermentation, so that the content of many beneficial metabolites in fermented walnut milk has been significantly increased.
Compared with Con group, The content of nobiletin, andrographolide, pristimerin, oleuropein, gartanin and cucurbitacin B in F group did not change significantly, but there was a very significant increase in EF group (p < 0.05) shown as (l–s). Nobiletin, andrographolide and pristimerin have anti-inflammatory activities (Bunbupha et al., Citation2020; Dai et al., Citation2019; Li et al., Citation2014; Zhao et al., Citation2021). With regard to NLRP3 inflammasome activation, pristimerin has shown selective and powerful suppressive action both in vitro and in vivo. This has led to get effects against NLRP3 inflammasome-related illness that are both therapeutic and preventative (Zhao et al., Citation2021). Studies are found that oleuropein, gartanin have antioxidant properties (Jung et al., Citation2006; Radha et al., Citation2021; Xie et al., Citation2015). With roles in both innate and adaptive immunity, cucurbitacin B has been identified as the compound that has been studied the majorly when it refers to the immunological response. The most commonly stated mechanisms were adjustment of acquired immunity proteins, repression of proinflammatory cytokines, suppression of COX-2 and NOS, lowering of oxidative stress (Silvestre et al., Citation2022). Moreover, compared with Con and F group, metabolites such as 6,7-dihydroxybergamottin and polydatin were markedly elevated in EF group (p < 0.05) only. 6,7-dihydroxybergamottin is the main furancoumarin in grapefruit and has antibacterial activity (Widelski et al., Citation2018). Recent in vitro and in vivo studies have exhibited that furacoumarin has additional biological activities, such as antioxidation and inflammation, which is same as polydatin for activities (Bian et al., Citation2022; Hung et al., Citation2017; Park et al., Citation2019a). These findings imply that the addition of the process of proteolysis walnut milk before fermentation can produce an increased variety and quantity of beneficial metabolites in walnut milk products than fermentation with pure walnut milk. And using a proteolysis process to treat walnut milk before fermentation can significantly improve the antioxidant activity of the product. This result is consistent with the increase in the content of beneficial metabolites in the above-related metabolite analysis, making it have more potential positive effects on the human body.
Figure 4. KEGG classifications and extraction for the significantly differential metabolites of each pairwise comparison of walnut milk. (a) Con vs. F (b) F vs. EF (c) Con vs. EF.

Some organic acids and derivatives such as guanidinosuccinic acid (p < 0.05) is down-regulated and generates hydroxyl radicals in aqueous solution which may cause free radical damage leading to some health manifestations as well as α-guanidinoglutaric acid (p < 0.05) in F group and EF group. This means that the content of metabolites which is harmful to human body can be reduced through proteolysis and fermentation (Yun-Fei et al., Citation1996).
The breakdown of proteins by the mechanisms of proteolysis and fermentation, which are intimately tied to the taste and purpose of food, could be the cause of changes in the peptide profile (Meng et al., Citation2022). More crucially, the inclusion of exogenous amino acids and peptides in the food matrix can increase the environmental toleration of the fermentation microorganisms and increase the efficiency of the fermentation procedure (Khavinson et al., Citation2016). Prior research used metabolomics to find short peptides with distinct positive health-promoting properties, such as VERGRRITSV and FVIEPNITPA can reduce blood pressure (Wang, Wang, et al., Citation2021a), LYGALGL can enhance antioxidant activity (Sun et al., Citation2022). EF group has more peptides than F group. Through the process of proteolysis walnut milk, the content and type of small peptides have significantly increased (p < 0.05), which will be more conducive to the development and metabolism of L. plantarum, and also means that the functional characteristics of the product are greater potential. Although many small peptides are produced, their functional characteristics need to be further studied.
3.5.3. KEGG
In determining the significant metabolic pathways and examine the dynamic changes in pathways during fermentation, the divergent metabolites were correlated with particular metabolic pathways using the KEGG database. Select the top 20 items with the highest significance based on p-value. As shown in (a–c). By analyzing the enrichment map of metabolic pathways, we found that the key pathway with the highest correlation of differential metabolites after proteolysis was identified, namely, lipid and amino acid metabolism such as steroid hormone biosynthesis, bile secretion, protein digestion and absorption, alanine, aspartate and glutamate metabolism and so on. Hence, the fermented walnut milk following proteolysis might be used to enhance functional components in fermented walnut milk and efficiently modify the ratio of nutrients in walnut milk, which would ultimately increase the bioavailability of walnut milk. However, the preliminary metabolic profiles could not explain the underlying mechanisms of synthesis or degradation of the different metabolites.
4. Conclusions
When the protein has been hydrolyzed, the walnut milk is processed into walnut milk. Walnut proteolysis effectively aided the development and fermentation of L. plantarum. The type and content of beneficial metabolites in fermented pure walnut milk cannot be greatly enhanced, whereas the fermented walnut milk following proteolysis can. Moreover, untargeted metabolomics and multivariate analysis were used to show how the metabolite pattern of walnut milk changed as a result of two differential processing techniques. The findings show that the differentially metabolites were primarily found in lipids and lipid-like compounds, as well as in amino acids, peptides, and analogues. These metabolite modifications have been shown to be crucial for the development of the potential function of walnut products. Most crucially, fermented walnut milk following proteolysis can considerably increase some functional metabolites and significantly improve its antioxidant activity in vitro as compared to fermented pure walnut milk with L. plantarum. The aforementioned findings demonstrate that using walnuts following proteolysis as a raw material to manufacture walnut milk is a successful process.
Disclosure statement
No potential conflict of interest was reported by the author(s).
Data availability statement
This is an Open Access article distributed under the terms of the Creative Commons Attribution-NonCommercial-NoDerivatives License, which permits non-commercial re-use, distribution, and reproduction in any medium, provided the original work is properly cited, and is not altered, transformed, or built upon in any way.
Additional information
Funding
References
- Adebo, O. A., & Gabriela Medina-Meza, I. (2020). Impact of fermentation on the phenolic compounds and antioxidant activity of whole cereal grains: A mini review. Molecules, 25(4), 927. https://doi.org/10.3390/molecules25040927
- Adebo, O. A., Njobeh, P. B., Adebiyi, J. A., Gbashi, S., & Kayitesi, E. (2017). Food metabolomics: A new frontier in food analysis and its application to understanding fermented foods. In M. C. Hueda (Ed.), Functional food - Improve health through adequate food (Vol. 11, pp. 211–234). InTech. https://doi.org/10.5772/intechopen.69171
- Adebo, O. A., Oyeyinka, S. A., Adebiyi, J. A., Feng, X., Wilkin, J. D., Kewuyemi, Y. O., Abrahams, A. M., & Tugizimana, F. (2021). Application of gas chromatography–mass spectrometry (GC-MS) -based metabolomics for the study of fermented cereal and legume foods: A review. International Journal of Food Science & Technology, 56(4), 1514–1534. https://doi.org/10.1111/ijfs.14794
- Ares-Yebra, A., Garabal, J. I., Carballo, J., & Centeno, J. A. (2019). Formation of conjugated linoleic acid by a Lactobacillus plantarum strain isolated from an artisanal cheese: Evaluation in miniature cheeses. International Dairy Journal, 90, 98–103. https://doi.org/10.1016/j.idairyj.2018.11.007
- Asioli, D., Aschemann-Witzel, J., Caputo, V., Vecchio, R., Annunziata, A., Næs, T., & Varela, P. (2017). Making sense of the “clean label” trends: A review of consumer food choice behavior and discussion of industry implications. Food Research International, 99, 58–71. https://doi.org/10.1016/j.foodres.2017.07.022
- Bartkiene, E., Krungleviciute, V., Juodeikiene, G., Vidmantiene, D., & Maknickiene, Z. (2015). Solid state fermentation with lactic acid bacteria to improve the nutritional quality of lupin and soya bean: Solid state fermentation to improve the nutritional quality of lupin and soya bean. Journal of the Science of Food and Agriculture, 95(6), 1336–1342. https://doi.org/10.1002/jsfa.6827
- Bian, H., Xiao, L., Liang, L., Xie, Y., Wang, H., Slevin, M., Tu, W.-J., & Wang, G. (2022). Polydatin prevents neuroinflammation and relieves depression via regulating Sirt1/HMGB1/NF-κB signaling in mice. Neurotoxicity Research, 40(5), 1393–1404. https://doi.org/10.1007/s12640-022-00553-z
- Bocchi, S., Rocchetti, G., Elli, M., Lucini, L., Lim, C.-Y., & Morelli, L. (2021). The combined effect of fermentation of lactic acid bacteria and in vitro digestion on metabolomic and oligosaccharide profile of oat beverage. Food Research International, 142, 110216. https://doi.org/10.1016/j.foodres.2021.110216
- Bulló, M., Nogués, M. R., López-Uriarte, P., Salas-Salvadó, J., & Romeu, M. (2010). Effect of whole walnuts and walnut-skin extracts on oxidant status in mice. Nutrition, 26(7–8), 823–828. https://doi.org/10.1016/j.nut.2009.09.002
- Bunbupha, S., Apaijit, K., Maneesai, P., Prasarttong, P., & Pakdeechote, P. (2020). Nobiletin ameliorates high-fat diet-induced vascular and renal changes by reducing inflammation with modulating AdipoR1 and TGF-β1 expression in rats. Life Sciences, 260, 118398. https://doi.org/10.1016/j.lfs.2020.118398
- Čelakovská, J., Bukač, J., Vaňková, R., Salavec, M., Krejsek, J., & Andrýs, C. (2021). Allergy to walnuts and hazelnuts in atopic dermatitis patients and analysis of sensitization to molecular components. Food and Agricultural Immunology, 32(1), 105–120. https://doi.org/10.1080/09540105.2021.1874883
- Chen, Y.-H., Huang, P.-H., Lin, F.-Y., Chen, W.-C., Chen, Y.-L., Yin, W.-H., Man, K.-M., & Liu, P.-L. (2011). Magnolol: A multifunctional compound isolated from the Chinese medicinal plant Magnolia officinalis. European Journal of Integrative Medicine, 3(4), e317–e324. https://doi.org/10.1016/j.eujim.2011.09.002
- Christensen, M. D., & Pederson, C. S. (1958). Factors affecting diacetyl production by lactic acid bacteria. Applied Microbiology, 6(5), 319–322. https://doi.org/10.1128/am.6.5.319-322.1958
- Coda, R., Rizzello, C. G., Pinto, D., & Gobbetti, M. (2012). Selected lactic acid bacteria synthesize antioxidant peptides during sourdough fermentation of cereal flours. Applied and Environmental Microbiology, 78(4), 1087–1096. https://doi.org/10.1128/AEM.06837-11
- Dai, Y., Chen, S.-R., Chai, L., Zhao, J., Wang, Y., & Wang, Y. (2019). Overview of pharmacological activities of Andrographis paniculata and its major compound andrographolide. Critical Reviews in Food Science and Nutrition, 59(sup1), S17–S29. https://doi.org/10.1080/10408398.2018.1501657
- Dan, T., Chen, H., Li, T., Tian, J., Ren, W., Zhang, H., & Sun, T. (2019). Influence of lactobacillus plantarum P-8 on fermented milk flavor and storage stability. Frontiers in Microbiology, 9, 3133. https://doi.org/10.3389/fmicb.2018.03133
- Das, G., Patra, J. K., Lee, S.-Y., Kim, C., Park, J. G., & Baek, K.-H. (2017). Analysis of metabolomic profile of fermented Orostachys japonicus A. Berger by capillary electrophoresis time of flight mass spectrometry. PLOS ONE, 12(7), e0181280. https://doi.org/10.1371/journal.pone.0181280
- Delgado, F. J., González-Crespo, J., Cava, R., García-Parra, J., & Ramírez, R. (2010). Characterisation by SPME–GC–MS of the volatile profile of a Spanish soft cheese P.D.O. Torta del Casar during ripening. Food Chemistry, 118(1), 182–189. https://doi.org/10.1016/j.foodchem.2009.04.081
- Feldman, E. B. (2002). The scientific evidence for a beneficial health relationship between walnuts and coronary heart disease. The Journal of Nutrition, 132(5), S1062–S1101. https://doi.org/10.1093/jn/132.5.1062S
- Fiorino, G. M., Tlais, A. Z. A., Losito, I., Filannino, P., Gobbetti, M., & Di Cagno, R. (2023). Triacylglycerols hydrolysis and hydroxy- and epoxy-fatty acids release during lactic fermentation of plant matrices: An extensive study showing inter- and intra-species capabilities of lactic acid bacteria. Food Chemistry, 412, 135552. https://doi.org/10.1016/j.foodchem.2023.135552
- García Arteaga, V., Demand, V., Kern, K., Strube, A., Szardenings, M., Muranyi, I., Eisner, P., & Schweiggert-Weisz, U. (2022). Enzymatic hydrolysis and fermentation of pea protein isolate and its effects on antigenic proteins, functional properties, and sensory profile. Foods (Basel, Switzerland), 11(1), 118. https://doi.org/10.3390/foods11010118
- Grosso, A. L., Riveros, C., Asensio, C. M., Grosso, N. R., & Nepote, V. (2020). Improving walnuts’ preservation by using walnut phenolic extracts as natural antioxidants through a walnut protein-based edible coating. Journal of Food Science, 85(10), 3043–3051. https://doi.org/10.1111/1750-3841.15395
- He, F., Liu, W., Wang, H., Zhang, Z., Gao, Y., Zhao, S., & Zhao, Y. (2010). The situation and prospects for further processing in China. Acta Horticulturae, 861(861), 79–82. https://doi.org/10.17660/ActaHortic.2010.861.9
- Hunaefi, D., Akumo, D. N., & Smetanska, I. (2013). Effect of fermentation on antioxidant properties of red cabbages. Food Biotechnology, 27(1), 66–85. https://doi.org/10.1080/08905436.2012.755694
- Hunaefi, D., Gruda, N., Riedel, H., Akumo, D. N., Saw, N. M. M. T., & Smetanska, I. (2013). Improvement of antioxidant activities in red cabbage sprouts by lactic acid bacterial fermentation. Food Biotechnology, 27(4), 279–302. https://doi.org/10.1080/08905436.2013.836709
- Hung, W.-L., Suh, J. H., & Wang, Y. (2017). Chemistry and health effects of furanocoumarins in grapefruit. Journal of Food and Drug Analysis, 25(1), 71–83. https://doi.org/10.1016/j.jfda.2016.11.008
- Hur, S. J., Lee, S. Y., Kim, Y.-C., Choi, I., & Kim, G.-B. (2014). Effect of fermentation on the antioxidant activity in plant-based foods. Food Chemistry, 160, 346–356. https://doi.org/10.1016/j.foodchem.2014.03.112
- İlyasoğlu, H., & Yilmaz, F. (2019). Characterisation of yoghurt enriched with polyunsaturated fatty acids by using walnut slurry. International Journal of Dairy Technology, 72(1), 110–119. https://doi.org/10.1111/1471-0307.12565
- Jayarathna, S., Priyashantha, H., Johansson, M., Vidanarachchi, J. K., Jayawardana, B. C., & Liyanage, R. (2021). Probiotic enriched fermented soy-gel as a vegan substitute for dairy yoghurt. Journal of Food Processing and Preservation, 45(1), 1. https://doi.org/10.1111/jfpp.15092
- Jung, H.-A., Su, B.-N., Keller, W. J., Mehta, R. G., & Kinghorn, A. D. (2006). Antioxidant xanthones from the pericarp of Garcinia mangostana (mangosteen). Journal of Agricultural and Food Chemistry, 54(6), 2077–2082. https://doi.org/10.1021/jf052649z
- Khavinson, V. K., Kuznik, B. I., Tarnovskaya, S. I., & Lin’kova, N. S. (2016). Short peptides and telomere length regulator hormone irisin. Bulletin of Experimental Biology and Medicine, 160(3), 347–349. https://doi.org/10.1007/s10517-016-3167-y
- Kim, H., Lim, C. Y., & Chung, M. S. (2021). Magnolia officinalis and its honokiol and magnolol constituents inhibit human norovirus surrogates. Foodborne Pathogens and Disease, 18(1), 24–30. https://doi.org/10.1089/fpd.2020.2805
- Laursen, M. F., Sakanaka, M., von Burg, N., Mörbe, U., Andersen, D., Moll, J. M., Pekmez, C. T., Rivollier, A., Michaelsen, K. F., Mølgaard, C., Lind, M. V., Dragsted, L. O., Katayama, T., Frandsen, H. L., Vinggaard, A. M., Bahl, M. I., Brix, S., Agace, W., Licht, T. R., … Roager, H. M. (2020). Breastmilk-promoted bifidobacteria produce aromatic amino acids in the infant gut. Microbiology, 1–39. https://doi.org/10.1101/2020.01.22.914994
- Li, M., Li, W., Wu, J., Zheng, Y., Shao, J., Li, Q., Kang, S., Zhang, Z., Yue, X., & Yang, M. (2020). Quantitative lipidomics reveals alterations in donkey milk lipids according to lactation. Food Chemistry, 310, 125866. https://doi.org/10.1016/j.foodchem.2019.125866
- Li, Q., Yin, R., Zhang, Q., Wang, X., Hu, X., Gao, Z., & Duan, Z. (2017). Chemometrics analysis on the content of fatty acid compositions in different walnut (Juglans regia L.) varieties. European Food Research and Technology, 243(12), 2235–2242. https://doi.org/10.1007/s00217-017-2925-z
- Li, S., Wang, H., Guo, L., Zhao, H., & Ho, C.-T. (2014). Chemistry and bioactivity of nobiletin and its metabolites. Journal of Functional Foods, 6, 2–10. https://doi.org/10.1016/j.jff.2013.12.011
- Li, Y.-C., Du, W., Meng, F.-B., Rao, J.-W., Liu, D.-Y., & Peng, L.-X. (2021). Tartary buckwheat protein hydrolysates enhance the salt tolerance of the soy sauce fermentation yeast Zygosaccharomyces rouxii. Food Chemistry, 342, 128382. https://doi.org/10.1016/j.foodchem.2020.128382
- Liu, W., Pu, X., Sun, J., Shi, X., Cheng, W., & Wang, B. (2022). Effect of lactobacillus plantarum on functional characteristics and flavor profile of fermented walnut milk. LWT-Food Science and Technology, 160, 113254. https://doi.org/10.1016/j.lwt.2022.113254
- Loh, L. X., Ng, D. H. J., Toh, M., Lu, Y., & Liu, S. Q. (2021). Targeted and nontargeted metabolomics of amino acids and bioactive metabolites in probiotic-fermented unhopped beers using liquid chromatography high-resolution mass spectrometry. Journal of Agricultural and Food Chemistry, 69(46), 14024–14036. https://doi.org/10.1021/acs.jafc.1c03992
- Lourens-Hattingh, A., & Viljoen, B. C. (2001). Yogurt as probiotic carrier food. International Dairy Journal, 11(1–2), 1–17. https://doi.org/10.1016/S0958-6946(01)00036-X
- Ma, X., Wang, J., Lu, X., & Qiao, C. (2013). A two-stage process for the production of a novel cheese flavor powder: The process of novel cheese flavor powder. Journal of Food Process Engineering, 36(5), 591–597. https://doi.org/10.1111/jfpe.12022
- Mäkinen, O. E., Wanhalinna, V., Zannini, E., & Arendt, E. K. (2016). Foods for special dietary needs: Non-dairy plant-based milk substitutes and fermented dairy-type products. Critical Reviews in Food Science and Nutrition, 56(3), 339–349. https://doi.org/10.1080/10408398.2012.761950
- Mao, B., Guo, W., Huang, Z., Tang, X., Zhang, Q., Yang, B., Zhao, J., Cui, S., & Zhang, H. (2022). Production of conjugated fatty acids in probiotic-fermented walnut milk with the addition of lipase. LWT-Food Science and Technology, 172, 114204. https://doi.org/10.1016/j.lwt.2022.114204
- Meinlschmidt, P. (2016). Soy protein hydrolysates fermentation: Effect of debittering and degradation of major soy allergens. LWT-Food Science and Technology, 71, 202–212. https://doi.org/10.1016/j.lwt.2016.03.026
- Meng, F.-B., Zhou, L., Li, J.-J., Li, Y.-C., Wang, M., Zou, L.-H., Liu, D.-Y., & Chen, W.-J. (2022). The combined effect of protein hydrolysis and Lactobacillus plantarum fermentation on antioxidant activity and metabolomic profiles of quinoa beverage. Food Research International, 157, 111416. https://doi.org/10.1016/j.foodres.2022.111416
- Mink, R., Sommer, S., Kölling, R., Schmarr, H.-G., Baumbach, L., & Scharfenberger-Schmeer, M. (2014). Diacetyl reduction by commercial Saccharomyces cerevisiae strains during vinification: Diacetyl reduction by commercial Saccharomyces cerevisiae strains. Journal of the Institute of Brewing, 120(1), 23–26. https://doi.org/10.1002/jib.106
- Mozzi, F., Ortiz, M. E., Bleckwedel, J., De Vuyst, L., & Pescuma, M. (2013). Metabolomics as a tool for the comprehensive understanding of fermented and functional foods with lactic acid bacteria. Food Research International, 54(1), 1152–1161. https://doi.org/10.1016/j.foodres.2012.11.010
- Mu, W., Yu, S., Zhu, L., Jiang, B., & Zhang, T. (2012). Production of 3-phenyllactic acid and 4-hydroxyphenyllactic acid by Pediococcus acidilactici DSM 20284 fermentation. European Food Research and Technology, 235(3), 581–585. https://doi.org/10.1007/s00217-012-1768-x
- Ni, Z.-J., Zhang, Y.-G., Chen, S.-X., Thakur, K., Wang, S., Zhang, J.-G., Shang, Y.-F., & Wei, Z.-J. (2022). Exploration of walnut components and their association with health effects. Critical Reviews in Food Science and Nutrition, 62(19), 5113–5129. https://doi.org/10.1080/10408398.2021.1881439
- Nieminen, M. T., Hernandez, M., Novak-Frazer, L., Kuula, H., Ramage, G., Bowyer, P., Warn, P., Sorsa, T., & Rautemaa, R. (2014). dl-2-Hydroxyisocaproic acid attenuates inflammatory responses in a Murine Candida albicans biofilm model. Clinical and Vaccine Immunology, 21(9), 1240–1245. https://doi.org/10.1128/CVI.00339-14
- Oliveira, I., Sousa, A., Ferreira, I. C. F. R., Bento, A., Estevinho, L., & Pereira, J. A. (2008). Total phenols, antioxidant potential and antimicrobial activity of walnut (Juglans regia L.) green husks. Food and Chemical Toxicology, 46(7), 2326–2331. https://doi.org/10.1016/j.fct.2008.03.017
- Ozcan, T., Sahin, S., Akpinar-Bayizit, A., & Yilmaz-Ersan, L. (2019). Assessment of antioxidant capacity by method comparison and amino acid characterisation in Buffalo milk kefir. International Journal of Dairy Technology, 72(1), 65–73. https://doi.org/10.1111/1471-0307.12560
- Park, B., Jo, K., Lee, T. G., Hyun, S.-W., Kim, J. S., & Kim, C.-S. (2019a). Polydatin inhibits NLRP3 inflammasome in dry eye disease by attenuating oxidative stress and inhibiting the NF-κB pathway. Nutrients, 11(11), 2792. https://doi.org/10.3390/nu11112792
- Park, M. K., Choi, H.-S., Kim, Y.-S., & Cho, I. H. (2019b). Comparison of volatile profiles in Fagopyrum esculentum (buckwheat) Soksungjang prepared with different starter cultures during fermentation. Food Science and Biotechnology, 28(4), 1037–1045. https://doi.org/10.1007/s10068-018-00549-6
- Pua, A., Tang, V. C. Y., Goh, R. M. V., Sun, J., Lassabliere, B., & Liu, S. Q. (2022). Ingredients, processing, and fermentation: Addressing the organoleptic boundaries of plant-based dairy analogues. Foods (Basel, Switzerland), 11(6), 875. https://doi.org/10.3390/foods11060875
- Qiao, Z., Wang, X., Wang, C., Han, J., Qi, W., Zhang, H., Liu, Z., & You, C. (2022). Lactobacillus paracasei BD5115-derived 2-hydroxy-3-methylbutyric acid promotes intestinal epithelial cells proliferation by upregulating the MYC signaling pathway. Frontiers in Nutrition, 9, 799053. https://doi.org/10.3389/fnut.2022.799053
- Radha, A., Jaswal, N., Thakur, K. K., & Gujral, I. K. (2021). A review on interaction of different preservatives with plasma proteins. Materials Today: Proceedings, 37, 2446–2452. https://doi.org/10.1016/j.matpr.2020.08.283
- Ros, E. (2010). Health benefits of nut consumption. Nutrients, 2(7), 652–682. https://doi.org/10.3390/nu2070652
- Ru, Y., Wang, Z., Li, Y., Kan, H., Kong, K., & Zhang, X. (2022). The influence of probiotic fermentation on the active compounds and bioactivities of walnut flowers. Journal of Food Biochemistry, 46(4), SI. https://doi.org/10.1111/jfbc.13887
- Sandhu, K. S., Punia, S., & Kaur, M. (2016). Effect of duration of solid state fermentation by Aspergillus awamorinakazawa on antioxidant properties of wheat cultivars. LWT - Food Science and Technology, 71, 323–328. https://doi.org/10.1016/j.lwt.2016.04.008
- Sathe, S. K., Venkatachalam, M., Sharma, G. M., Kshirsagar, H. H., Teuber, S. S., & Roux, K. H. (2009). Solubilization and electrophoretic characterization of select edible nut seed proteins. Journal of Agricultural and Food Chemistry, 57(17), 7846–7856. https://doi.org/10.1021/jf9016338
- Schlegel, K., Lidzba, N., Ueberham, E., Eisner, P., & Schweiggert-Weisz, U. (2021). Fermentation of lupin protein hydrolysates—Effects on their functional properties, sensory profile and the allergenic potential of the major lupin allergen lup an 1. Foods (basel, Switzerland), 10(2), 281. https://doi.org/10.3390/foods10020281
- Sharma, A., Noda, M., Sugiyama, M., Ahmad, A., & Kaur, B. (2021). Production of functional buttermilk and soymilk using Pediococcus acidilactici BD16 (alaD+). Molecules, 26(15), 4671. https://doi.org/10.3390/molecules26154671
- Shudong, P., Guo, C., Wu, S., Cui, H., Suo, H., & Duan, Z. (2022). Bioactivity and metabolomics changes of plant-based drink fermented by Bacillus coagulans VHProbi C08. LWT - Food Science and Technology, 156, 113030. https://doi.org/10.1016/j.lwt.2021.113030
- Shumoy, H., Gabaza, M., Vandevelde, J., & Raes, K. (2019). Impact of fermentation on in vitro bioaccessibility of phenolic compounds of tef injera. LWT - Food Science and Technology, 99, 313–318. https://doi.org/10.1016/j.lwt.2018.09.085
- Silvestre, G. F. G., de Lucena, R. P., & da Silva Alves, H. (2022). Cucurbitacins and the immune system: Update in research on anti-inflammatory, antioxidant, and immunomodulatory mechanisms. Current Medicinal Chemistry, 29(21), 3774–3789. https://doi.org/10.2174/0929867329666220107153253
- Sim, D.-H., Shin, K.-C., & Oh, D.-K. (2015). 13- Hydroxy- 9Z, 11E- octadecadienoic acid production by recombinant cells expressing Burkholderia thailandensis 13- lipoxygenase. Journal of the American Oil Chemists’ Society, 92(9), 1259–1266. https://doi.org/10.1007/s11746-015-2694-4
- Spence, L. A., Henschel, B., Li, R., Tekwe, C. D., & Thiagarajah, K. (2023). Adding walnuts to the usual diet can improve diet quality in the United States: Diet modeling study based on NHANES 2015–2018. Nutrients, 15(2), 258. https://doi.org/10.3390/nu15020258
- Sun, L., Li, M., Zhang, S., Bao, Z., & Lin, S. (2022). Explore the mechanism of pulsed electric field technology on improving the antioxidant activity of Leu-Tyr-Gly-Ala-Leu-Gly-Leu. Food Bioscience, 47, 101629. https://doi.org/10.1016/j.fbio.2022.101629
- Tangyu, M., Muller, J., Bolten, C. J., & Wittmann, C. (2019). Fermentation of plant-based milk alternatives for improved flavour and nutritional value. Applied Microbiology and Biotechnology, 103(23–24), 9263–9275. https://doi.org/10.1007/s00253-019-10175-9
- Verbeke, K. A., Boobis, A. R., Chiodini, A., Edwards, C. A., Franck, A., Kleerebezem, M., Nauta, A., Raes, J., van Tol, E. A. F., & Tuohy, K. M. (2015). Towards microbial fermentation metabolites as markers for health benefits of prebiotics. Nutrition Research Reviews, 28(1), 42–66. https://doi.org/10.1017/S0954422415000037
- Wang, C.-Y., Wu, S.-J., & Shyu, Y.-T. (2014). Antioxidant properties of certain cereals as affected by food-grade bacteria fermentation. Journal of Bioscience and Bioengineering, 117(4), 449–456. https://doi.org/10.1016/j.jbiosc.2013.10.002
- Wang, J., Wang, G., Zhang, Y., Zhang, R., & Zhang, Y. (2021a). Novel angiotensin-converting enzyme inhibitory peptides identified from walnut glutelin-1 hydrolysates: Molecular interaction, stability, and antihypertensive effects. Nutrients, 14(1), 151. https://doi.org/10.3390/nu14010151
- Wang, Q., Zhi, T., Han, P., Li, S., Xia, J., Chen, Z., Wang, C., Wu, Y., Jia, Y., & Ma, A. (2021b). Potential anti-inflammatory activity of walnut protein derived peptide leucine-proline-phenylalanine in lipopolysaccharides-irritated RAW264.7 cells. Food and Agricultural Immunology, 32(1), 663–678. https://doi.org/10.1080/09540105.2021.1982870
- Wang, X., Li, X., & Chen, D. (2011). Evaluation of antioxidant activity of isoferulic acid in vitro. Natural Product Communications, 6(9), 1934578X1100600. https://doi.org/10.1177/1934578X1100600919
- Wang, X., Zhang, P., & Zhang, X. (2021). Probiotics regulate gut microbiota: An effective method to improve immunity. Molecules, 26(19), 6076. https://doi.org/10.3390/molecules26196076
- Wang, X., Zhang, Z., Xu, H., Li, X., & Hao, X. (2020). Preparation of sheep bone collagen peptide–calcium chelate using enzymolysis-fermentation methodology and its structural characterization and stability analysis. RSC Advances, 10(20), 11624–11633. https://doi.org/10.1039/D0RA00425A
- Widelski, J., Luca, S., Skiba, A., Chinou, I., Marcourt, L., Wolfender, J.-L., & Skalicka-Wozniak, K. (2018). Isolation and antimicrobial activity of coumarin derivatives from fruits of Peucedanum luxurians Tamamsch. Molecules, 23(5), 1222. https://doi.org/10.3390/molecules23051222
- Xiang, H., Sun-Waterhouse, D., Waterhouse, G. I. N., Cui, C., & Ruan, Z. (2019). Fermentation-enabled wellness foods: A fresh perspective. Food Science and Human Wellness, 8(3), 203–243. https://doi.org/10.1016/j.fshw.2019.08.003
- Xie, P., Huang, L., Zhang, C., & Zhang, Y. (2015). Phenolic compositions, and antioxidant performance of olive leaf and fruit (Olea europaea L.) extracts and their structure–activity relationships. Journal of Functional Foods, 16, 460–471. https://doi.org/10.1016/j.jff.2015.05.005
- Xu, J., Jin, F., Hao, J., Regenstein, J. M., & Wang, F. (2020). Preparation of soy sauce by walnut meal fermentation: Composition, antioxidant properties, and angiotensin-converting enzyme inhibitory activities. Food Science & Nutrition, 8(3), 1665–1676. https://doi.org/10.1002/fsn3.1453
- Yang, H., Qu, Y., Li, J., Liu, X., Wu, R., & Wu, J. (2020). Improvement of the protein quality and degradation of allergens in soybean meal by combination fermentation and enzymatic hydrolysis. LWT - Food Science and Technology, 128, 109442. https://doi.org/10.1016/j.lwt.2020.109442
- Yang, M.-H., & Choong, Y.-M. (2001). A rapid gas chromatographic method for direct determination of short-chain (C2–C12) volatile organic acids in foods. Food Chemistry, 75(1), 101–108. https://doi.org/10.1016/S0308-8146(01)00211-4
- Yang, Z., Zhu, X., Wen, A., & Qin, L. (2022). Development of probiotics beverage using cereal enzymatic hydrolysate fermented with Limosilactobacillus reuteri. Food Science & Nutrition, 10(9), 3143–3153. https://doi.org/10.1002/fsn3.2913
- Yarlina, V. P., Djali, M., & Andoyo, R. (2020). A review of protein hydrolysis fermented foods and their potential for health benefits. IOP Conference Series: Earth and Environmental Science, 443(1), 012085. https://doi.org/10.1088/1755-1315/443/1/012085
- Yi, C., Li, Y., Zhu, H., Liu, Y., & Quan, K. (2021). Effect of Lactobacillus plantarum fermentation on the volatile flavors of mung beans. LWT - Food Science and Technology, 146, 111434. https://doi.org/10.1016/j.lwt.2021.111434
- Yun-Fei, L., Moriwaki, A., Hayashi, Y., Tomizawa, K., Itano, T., & Matsui, H. (1996). Effects of neurotensin on neurons in the rat central amygdaloid nucleus in vitro. Brain Research Bulletin, 40(2), 135–141. https://doi.org/10.1016/0361-9230(96)00044-5
- Zha, M., Li, K., Zhang, W., Sun, Z., Kwok, L.-Y., Menghe, B., & Chen, Y. (2021). Untargeted mass spectrometry-based metabolomics approach unveils molecular changes in milk fermented by Lactobacillus plantarum P9. LWT - Food Science and Technology, 140, 110759. https://doi.org/10.1016/j.lwt.2020.110759
- Zhang, X., Zhang, S., Xie, B., & Sun, Z. (2021). Influence of lactic acid bacteria fermentation on physicochemical properties and antioxidant activity of chickpea yam milk. Journal of Food Quality, 2021, 1–9. Article ID: 5523356. https://doi.org/10.1155/2021/5523356
- Zhao, Q., Bi, Y., Guo, J., Liu, Y., Zhong, J., Pan, L., Tan, Y., & Yu, X. (2021). Pristimerin protects against inflammation and metabolic disorder in mice through inhibition of NLRP3 inflammasome activation. Acta Pharmacologica Sinica, 42(6), 975–986. https://doi.org/10.1038/s41401-020-00527-x
- Zhong, L., Bornman, J. F., Wu, G., Hornoff, A., Dovi, K. A. P., AL-Ali, H., Aslam, N., & Johnson, S. K. (2018). The nutritional and phytochemical composition of the indigenous Australian pindan walnut (Terminalia cunninghamii) Kernels. Plant Foods for Human Nutrition, 73(1), 40–46. https://doi.org/10.1007/s11130-017-0647-9
- Zhou, X., Liu, Z., Xie, L., Li, L., Zhou, W., & Zhao, L. (2022). The correlation mechanism between dominant bacteria and primary metabolites during fermentation of red sour soup. Foods (Basel, Switzerland), 11(3), 341. https://doi.org/10.3390/foods11030341