ABSTRACT
Plant chemodiversity is a helpful tool for disease prevention and a basis for adjuvant treatments to conventional therapies. In this regard, the extract of Polypodium leucotomos rhizomes, PLE, has shown benefits fighting inflammation and recurrent infections but its molecular mechanism is poorly undersood. This work shows that Plesinox 3A, containing PLE, Vitamins A, C, and selenium, helps modulate the initial inflammatory response triggered by bacterial LPS through the upregulation of IL8 and IL10, together with downregulation of COX2, IL1B and TNF, in a more efficient manner than PLE alone. Additionally, this formulation enhances the antiviral response through the upregulation of MX1, IFNA1 and IFNG in different cell types. Finally, the addition of vitamins and selenium to PLE in Plesinox 3A greatly boosts the anti-bacterial properties of PLE alone. Overall, these findings support the combined use of PLE, vitamins, and selenium, in the form of Plesinox 3A as an immune booster to prevent recurrent infections, highlighting a gene set potentially involved in its beneficial effect, as well as showing its direct anti-bacterial properties, which are greater than those of PLE alone.
GRAPHICAL ABSTRACT
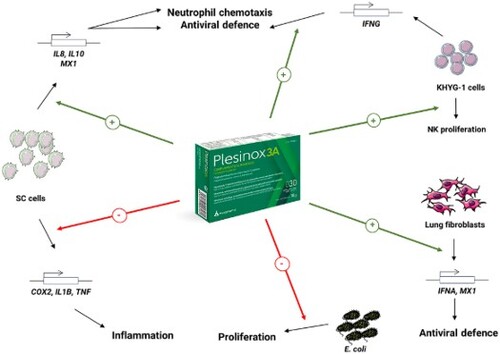
Introduction
Medicinal plants, despite being underscored for a long time, are increasingly acquiring an important place in modern medicine due to their ability to produce myriads of active principles. These phytochemicals possess biologically active beneficial effects and provide protection to the plants from insects, bacteria, viruses and other commensals (Vellingiri et al., Citation2020). As the search of new antimicrobial agents becomes increasingly important in medicine, phytochemicals progressively gain attention as potential alternatives or chemical backbones for the synthesis of new molecules. In this regard, there is compelling experimental evidence of the potential of plant sources to overcome antimicrobial resistances through the production of small proteins commonly referred to as antimicrobial peptides (AMPs) (Sharma et al., Citation2022).
One of the relevant consequences of some blood-borne and respiratory viruses is the generation of oxidative stress (Ivanov et al., Citation2017). It is well known that plants display a wide range of substances with antioxidant properties that help mitigate this situation. For example, flavonoids isolated from different plant sources have shown both antioxidant and antimicrobial activity in different experimental setups, both in vitro and in vivo (Al Aboody & Mickymaray, Citation2020; Baharfar et al., Citation2015; Jiang et al., Citation2011; Wang et al., Citation2020; Yin et al., Citation2019). On the other hand, plant extracts mainly composed by terpenes, have also demonstrated an antioxidant activity concomitant with antimicrobial, antifungal or antiviral effects (El-Sayed et al., Citation2022; Nadjib, Citation2020; Sun et al., Citation2020). Apart of mitigating oxidative stress due to their intrinsic antioxidant properties, some of the proposed mechanisms of action of these compounds are the disruption of cell wall integrity in the case of bacteria and fungi (El-Sayed et al., Citation2022) and the inhibition of key enzyme activities and protein interactions for viruses (Nadjib, Citation2020).
Apart of the direct action against the biology of microorganisms, which is undertaken once the infection has occurred, an important property of plant-based formulations is their immunomodulatory effects.
The first response of the immune system against an infection is inflammation, which involves the secretion of different second messengers and cytokines with pleiotropic effects. Regarding this physiologic response, a great body of evidence supports the ability of plant extracts to modulate the response to LPS, which emulates bacterial infections in vitro. Specifically, these works demonstrate that exposure of LPS-challenged immune cells to plant extracts reduces both the activation of inflammation-related signalling pathways and the secretion of inflammation mediators (Schink et al., Citation2018; Zhu et al., Citation2018), which upon long-term activation would become deleterious for the organism.
Contrarily, phytochemicals can also help stimulate the immune system at the first steps of infection, thus promoting the rapid elimination of pathogens. In this regard, plant extracts have shown to promote the proliferation of immune effector cells, the release of immune activator molecules, the phagocytosis of pathogens and the natural killer cell-mediated cytotoxicity in vitro (del Carmen Juárez-Vázquez et al., Citation2013; Park et al., Citation2013), as well as the proliferation and phagocytic ability of splenocytes in animal models (Nudo & Catap, Citation2011).
A plant extract that has received wide attention due to its immunomodulatory properties is that obtained from rhizomes of the fern Polypodium leucotomos. Although it has long been used to prevent and treat dermatological diseases and as a photoprotective agent (Middelkamp-Hup et al., Citation2004; Segars et al., Citation2021), a piece of evidence supports the immunomodulatory capabilities of this extract through the promotion of PBMC, T and NK lymphocytes proliferation, the inhibition of pro-inflammatory cytokines, the stimulation of anti-inflammatory and antiviral cytokines and the reduction of excessive expression of adhesion molecules involved in immune cell extravasation (Sempere et al., Citation1997; Sempere-Ortells et al., Citation2002). Moreover, in a model of infant tonsilitis, the application of this plant extract has shown immune system-boosting actions that may prevent from recurrent infection episodes (Sánchez-Rodríguez, Citation2018). Interestingly, in an in vivo setup, intake of PLE has been correlated with a decrease in the number of infections in a cohort of athletes (Solivellas & Martín, Citation2012).
All these things considered, this work aimed at contributing to shed light on the potential molecular mediators of the in vitro immunomodulatory effects against recurrent infections of the food supplement formulation Plesinox 3A, containing PLE combined with vitamin A, vitamin C and selenium, as well as showing its improved properties over PLE alone.
Materials and methods
Cell culture
SC cells (ATCC CRL-9855) were cultured in Iscove’s Modified Dulbecco’s Medium (IMDM, Gibco) supplemented with 4 mM L-glutamine, 1.5 g/L sodium bicarbonate 0.05 mM 2-mercaptoethanol, 0.1 mM hypoxanthine and 0.016 mM thymidine and 10% FBS. Human Lung Fibroblasts (HLF) were purchased from Cell Applications and cultured in HLF Growth medium (Cell Applications). KHYG-1 (JCRB0156) were purchased from Japanese Collection of Research Bioresources Cell Bank and cultured in Roswell Park Memorial Institute (RPMI, Gibco) medium supplemented with IL-2 100 U/mL and 10% fetal bovine serum.
Cell viability
SC cells were seeded in 96-well plates (Costar) at a density of 5·104 cells/well and subsequently exposed to PLE alone or Plesinox 3A for 48 h at 37°C. Next, cells were washed with PBS and incubated with MTT (Sigma-Aldrich) for 3 h at 37°C. The Abs 570 nm of the DMSO-solubilized crystals was recorded with a multi-well reader.
HLF were seeded in 96-well plates (Falcon) at 104 cells/well and exposed to Plesinox 3A at different concentrations in HLF growth medium for 24 h. After the exposure, cells were washed with PBS and incubated with MTT (Thermo Scientific) for 3 h at 37°C. The Abs 570 nm of the DMSO-solubilized crystals was recorded with a multi-well reader.
KHYG-1 cells were seeded in 96-well plates (Falcon) at 2·104 cells/well and exposed to Plesinox 3A at different concentrations in growth medium with reduced IL-2 (10 U/mL) for 72 h. After the exposure, resazurin sodium salt (Thermo Scientific) was added to a final concentration of 44 µM and incubated for 3 h at 37°C. Next, fluorescence (Ex = 554 nm/Em = 593 nm) was recorded with a multi-well plate reader.
Inflammation
SC cells were grown to 100% confluence in 96-well plates and treated for 3 h with lipopolysaccharide (LPS) from Escherichia coli (Sigma-Aldrich) at 200 ng/mL to induce the expression of inflammation-related genes. After this period, PLE alone or Plesinox 3A was added to the culture medium at different concentrations, in the presence of LPS, for additional 24 h to assess their anti-inflammatory potential.
Antiviral activity
SC cells were grown to 100% confluence in 96-well plates and treated for 24 h with PLE alone or Plesinox 3A to assess their anti-viral potential. On the other hand, HLF were seeded in 12-well plates at 3·105 cells/well and treated for 24 h with Plesinox 3A to assess its anti-viral potential, whereas KHYG-1 cells were seeded in 12-well plates at a density of 5·105 cells/mL and treated for 24 h with Plesinox 3A to assess its anti-viral potential.
Gene expression
RNA from LPS-challenged or treated SC cells was isolated with EZNA Total RNA extraction Kit (Omega Biotek), whereas RNA from treated HLF and KHYG-1 was isolated with RNeasy Mini Kit (Qiagen). The effect of the treatments in the expression of inflammation-related genes in SC cells was evaluated through qPCR using specific primers for IL6, IL8, COX2, TNF, IL1B, and MX1 using GAPDH as housekeeping gene. The evaluation of antiviral defense-related genes was performed in SC cells through qPCR using specific primers for MX1, using 18S as a housekeeping gene, and in HLF using specific fluorescent probe-based assays for MX1, using ACTB as a housekeeping gene. Finally, the evaluation of antiviral potential in KHYG-1 cells was assessed through qPCR with a StepOnePlus™ Real-Time PCR System (Applied Biosystems) using specific fluorescent probe-based assays for IFNG, using ACTB as a housekeeping gene. Relative gene expression was calculated with the 2-ΔΔCt expression (Supplementary Figure 1).
Anti-bacterial activity
Pre-inocula from single colonies of Escherichia coli BL-21 (DE3) were grown in liquid LB medium in the presence of PLE alone or Plesinox 3A for 24 h at 37°C. After the incubation period, aliquots of these cultures were diluted 1/1000 in fresh LB medium and exposed to PLE or Plesinox 3A for 24 h at 37°C. Then, the bacterial viability was assessed through the absorbance measurements at 570 nm of the reduced form of iodonitrotetrazolium (INT) reagent.
Results
Characterisation of the cytotoxicity profile of Plesinox 3A
Prior to the functional evaluation of Plesinox 3A, the cytotoxicity profile of the mixture was evaluated in two in vitro models. With the idea of evaluating the functional effects of Plesinox 3A in the innate immune system, the monocyte/macrophage cell line SC was chosen as a model. In this regard, the viability of SC cells treated with PLE alone was not compromised at any of the tested doses. Similarly, treatment with Plesinox 3A for 48 h at 0.005 and 0.01 mg/mL did not trigger relevant changes in cell viability, whereas doses ranging from 0.05 mg/mL to 1 mg/mL exerted a dose-dependent cytotoxicity, by reducing cell viability between 29.6% and 60.6% versus the same dose of PLE alone (A).
Figure 1. Cytotoxicity profile of PLE alone and Plesinox 3A. (A) Relative cell viability values (calculated versus untreated control) of SC cells treated for 48 h with PLE alone or Plesinox 3A, obtained by the MTT assay. (B) Cell viability values of HLF treated for 24 h with Plesinox 3A, obtained by the MTT assay.

As one of the main routes of recurrent infections is the respiratory system, primary human lung fibroblasts (HLF) were used as a representative model of body invasion route of environmental pathogens. As shown in B, exposure of HLF to Plesinox 3A diluted at 0.01 mg/mL in cell culture medium for 24 h did not impact cell viability. Conversely, from 0.03 mg/mL onwards, a subtle cytotoxic effect was observed that reached a maximum effect at 1 mg/mL, with a reduction of cell viability of 26.2% versus untreated cells.
Overall, these data indicated that Plesinox 3A had a more cytotoxic profile than PLE alone at the same doses, presumably due to synergistic actions between the extract components and the added vitamins in SC cells. On the other hand, the cytotoxicity profile of this formulation in HLF was very subtle, with mild reductions of cell viability even at the highest dose of 1 mg/mL.
Plesinox 3A displays anti-inflammatory properties in SC cells
The first response upon pathogen invasion is inflammation (Serhan, Citation2017), which has a widely recognised physiological function, provided that its duration is strictly regulated (Panigrahy et al., Citation2021). Nonetheless, an excessively prolonged inflammatory response is associated with a range of diseases. Thus, the adjuvant properties of Plesinox 3A were tested in a model of acute inflammation involving SC cells challenged with bacterial LPS.
As shown in A, the expression levels of the gene encoding the neutrophil-recruiting chemokine IL-8 were greatly enhanced upon exposure to PLE at 0.01, 0.05 and 0.1 mg/mL in SC cells by more than 2-fold versus untreated cells challenged with LPS. Importantly, the addition of vitamins and selenium to the plant extract clearly enhanced this effect, with an upregulation in the expression of this gene of 7-fold and 40-fold when Plesinox 3A was applied at 0.05 and 0.1 mg/mL, respectively.
Figure 2. The exposure of SC cells to Plesinox 3A ameliorates the gene expression profile upon inflammatory stimuli. Relative expression values IL8 (A), IL10 (B), COX2 (C), IL1B (D) and TNF (E) in SC cells exposed to bacterial LPS 200 ng/mL for 3 h and subsequently treated with PLE alone or Plesinox 3A at different concentrations for additional 24 h.

In addition, the treatment of LPS-challenged SC cells with PLE 24 h also enhanced the expression levels of IL10, whose product displays anti-inflammatory properties and boosts the maturation of B cells and the production of antibodies (Iyer & Cheng, Citation2012; Saxena et al., Citation2015), between 2-fold and more than 5-fold at the three doses tested. Of note, this effect became more pronounced upon treatment with Plesinox 3A at the same doses, with a dose-dependent upregulation in the expression of this gene between 7-fold and 162-fold at 0.01 mg/mL and 0.1 mg/mL, respectively (B).
Regarding the expression of pro-inflammatory molecules, the application of PLE at 0.01–0.1 mg/mL to SC cells challenged with LPS greatly diminished the expression of COX2 to ∼41-45% of the values registered in untreated cells in a statistically significant manner. This effect was very similar for Plesinox 3A at 0.01 mg/mL and 0.05 mg/mL. Strikingly, this combination at 0.1 mg/mL turned the expression of this gene into almost undetectable, with expression values even lower than those of LPS-unchallenged SC cells (C). Consistently, very similar results were obtained for IL1B, whose expression was reduced in a dose-dependent manner by Plesinox 3A to such an extent that it was barely detectable after application at 0.1 mg/mL and displayed lower levels than in cells treated with PLE alone at the three doses tested (D). In addition, TNF expression was also downregulated in LPS-challenged SC cells simultaneously treated with PLE or Plesinox 3A, which displayed relative expression values between ∼35% and ∼65% lower than untreated cells at the three doses tested. In this case, the boosting effect of the vitamins and selenium was more evident at 0.1 mg/mL, with a reduction of 65.4% by Plesinox 3A versus 54.3% with PLE alone (E).
All in all, these results support the beneficial properties of Plesinox 3A by helping resolve the acute inflammation triggered by the invasion of pathogens at two different levels: on one hand, by accelerating the neutrophil-recruiting expression profile, via upregulation of IL10. On the other hand, by preventing the chronic hyperactivation of pro-inflammatory molecule secretion, both directly through downregulation of positive modulators of inflammation (COX2, TNF, IL1B) and indirectly through upregulation of the anti-inflammatory cytokine IL10.
Plesinox 3A enhances antiviral response
Among the most prevalent recurrent infections, those derived from respiratory viruses must be noted, especially in cold seasons. This fact is of paramount relevance in the current context of COVID19 pandemics. For this reason, the expression of several molecules involved in the antiviral response was evaluated in different cell types involved in the protective response versus viral infections.
MX1 encodes a GTPase that inhibits the replication of several DNA and RNA viruses and is broadly expressed across different cell types (Lee & Vidal, Citation2002). Importantly, the expression of this gene, which underwent a mild upregulation in SC cells after treatment with PLE at the three tested doses, exhibited a clear dose-dependent upregulation in SC cells treated with Plesinox 3A at the dose range 0.01-0.1 mg/mL for 24 h, with relative expression values more than 2-fold higher than in untreated cells at the doses 0.05 and 0.1 mg/mL (A). Again, these data reinforce the beneficial effects of combining the plant extract with vitamins and selenium. Additionally, the expression of this gene also showed an increasing trend in HLF exposed to the same doses of the combined formulation, although data did not reach statistical significance (B).
Figure 3. The application of Plesinox 3A enhances antiviral response in different cell types. (A) Relative expression values of MX1 in SC cells treated for 16 h with PLE alone or Plesinox 3A. (B) Relative expression values of MX1 in HLF treated for 24 h with Plesinox 3A. (C) Relative expression values of IFNA in HLF treated for 24 h with Plesinox 3A. (D) Cell viability values of KHYG-1 cells treated for 72 h with Plesinox 3A at different doses, obtained by incubation with Resazurin. (E) Relative expression values of IFNG in KHYG-1 cells treated for 24 h with Plesinox 3A.

Interferon A1 (IFNA1) belongs to the type I family of interferons, which are ubiquitously expressed and play an important role in fighting viral infections (Murira & Lamarre, Citation2016). The expression of this molecule was evaluated in HLF as an example of peripheral cell type infected by external pathogens. As shown in C, HLF treated with Plesinox 3A underwent a dose-dependent upregulation of IFNA1, which reached statistical significance at 1 mg/mL, with an increase in relative expression of 0.9-fold versus untreated control. Interestingly, this formulation at 0.1 mg/mL also triggered an increase in the average expression levels of this gene by 0.4-fold versus untreated cells that did not result as statistically significant.
Finally, a different line of antiviral defense is the action of Natural Killer (NK) cells, which play a crucial role by both secreting cytokines and directly lysing infected cells (Piersma & Brizić, Citation2022). For this purpose, the NK-derived cell line KHYG-1 was subjected to treatment with Plesinox 3A and different parameters were evaluated. On one hand, the proliferative ability of this cell line was slightly boosted upon exposure to the formulation at 100 ng/mL and 1 µg/mL for 72 h, although data did not reach statistical significance (D). Most importantly, the expression of IFNG, which encodes the Type II interferon IFNγ, was positively modulated in a dose-dependent manner by Plesinox 3A, which significantly increased its relative expression by 0.8-fold at 0.01 mg/mL and 1.6-fold at 1 mg/mL (E).
Taken together, the data shown in this section greatly support the beneficial properties of Plesinox 3A in the prevention and resolution of viral infections from the earliest stage of tissue invasion to the elimination of infected cells.
Plesinox 3A inhibits bacterial growth
The data presented so far strongly substantiate the ability of Plesinox 3A to boost the expression of molecules involved in the innate immune response, as well as in fighting viral infections. Moreover, it must be noted that recurrent infections in airways can be of both viral and bacterial origin (Anderson & Paterek, Citation2023), and that the increasing emergence of resistance to classic antibiotics brings the need to find new antibacterial substances and/or prebiotics.
To address the potential of Plesinox 3A to help fighting bacterial infections, a culture of E. coli was incubated in the presence of the formulation at different concentrations, and its colony-forming ability was evaluated. As shown in , the incubation of E. coli in the presence of Plesinox 3A resulted highly and dose-dependently cytotoxic at doses ranging from 0.1 mg/mL to 5 mg/mL, which reduced the cell viability between ∼20% and ∼70% versus untreated cells. Most importantly, the addition of vitamins and selenium clearly boosted the anti-bacterial effect of PLE at all the doses tested, with Plesinox 3A triggering statistically significant reductions in bacterial viability over PLE alone by 22.8% and 26.0% at 0.5 and 5 mg/mL.
Figure 4. Plesinox 3A displays better anti-bacterial properties than PLE alone. Relative cell viability values (calculated versus untreated control) of Escherichia coli liquid cultures subjected to treatment with PLE alone or Plesinox 3A for 24 h.

In summary, these results strongly suggest that the intake of Plesinox 3A could help reduce the bacterial growth in recurrent infections, a hypothesis that is supported by its immune-boosting properties in in vitro models of some respiratory airway infections (Solivellas & Martín, Citation2012).
Discussion
The extraordinary number of plant secondary metabolites constitutes an important pool for the development of food supplements potentially useful as adjuvants in the treatment of microbial infections affecting for instance urinary (Chiancone et al., Citation2019; Koradia et al., Citation2019) and gastrointestinal tracts (Ayala et al., Citation2014). On the other hand, vitamins and selenium have also demonstrated benefits fighting respiratory infections (De Luca et al., Citation2012; Ferrara et al., Citation2021; Wimalawansa, Citation2022; Xiao & He, Citation2021). Most importantly, the combination of plant extracts with vitamins is a powerful tool to prevent pathogen colonisation of different organs (Jeitler et al., Citation2022; Kutwin et al., Citation2022; Rösler et al., Citation2012) and to boost the immune system (Aguzzi et al., Citation2020).
Among plant extracts, PLE, obtained from Polypodium leucotomos rhizomes, has been proposed as a relevant booster of innate immune system (Sempere et al., Citation1997; Sempere-Ortells et al., Citation2002) and has proven to be a useful tool to prevent respiratory recurrent infections (Sánchez-Rodríguez, Citation2018; Solivellas & Martín, Citation2012). Even though the molecular mechanism of action underlying the protective effects of PLE against UVR-induced skin damage has been thoroughly characterised (Jańczyk et al., Citation2007; Portillo-Esnaola et al., Citation2021; Zattra et al., Citation2009), scarce evidence is available regarding the targets of this extract in the context of bacterial and viral infections. Based on this evidence, this work aimed at highlighting some of the gene targets potentially involved in this beneficial effect and describing the direct anti-microbial effects triggered by Plesinox 3A, which results from the combination of PLE with Vitamin A, Vitamin C and selenium, contributing to fighting recurrent infections.
The results presented here support that, at subcytotoxic doses, the formulation Plesinox 3A partially reverses the pro-inflammatory gene expression profile triggered by bacterial LPS in the human cell line SC. Specifically, it upregulates the anti-inflammatory cytokine IL10 and the neutrophil-recruiting chemokine IL8. Concomitantly, it downregulates the pro-inflammatory molecules COX2, TNF and IL1B. These data are consistent with previous reports showing an increase in the secretion of IL10 and a decrease in the release of IL-1β in peripheral blood mononuclear cells (Sempere et al., Citation1997). Nonetheless it is worth noting that, under the experimental conditions tested in this work, the addition of vitamins and selenium clearly boosted the anti-inflammatory properties of PLE, especially in the case of IL8 and IL10. Moreover, the expression of the antiviral molecule MX1 was also enhanced in SC cells subjected to PLE treatment, a phenomenon that became more pronounced upon addition of vitamins and selenium to the plant extract. The antiviral gene expression signature triggered by Plesinox 3A could also be applicable to HLF, which underwent an upregulation of both MX1 and IFNA1 upon exposure to the formulation. Besides, this effect was also confirmed in the NK cell line KHYG-1, where the expression of IFNG was clearly upregulated by the formulation, and a subtle proliferation-promoting effect could also be observed. These results are also in line with those published by Sempere et al., which demonstrated the antiviral properties of PLE alone through the stimulation of interferon gamma secretion (Sempere et al., Citation1997) and the proliferation of NK cells (Sempere-Ortells et al., Citation2002). In addition to the modulation of the expression pattern of immune response-related molecules in different cell types, Plesinox 3A clearly surpasses the bacterial growth-inhibitory potential of PLE alone, thus supporting its use in not only viral, but also bacterial infections.
Conclusion
In conclusion, the results presented strongly support that the addition of vitamins and selenium to PLE found in Plesinox 3A enhance the immunomodulatory, antiviral and antibacterial properties of the plant extract alone and substantiate its efficacy as a food supplement against recurrent infections by providing evidence of transcriptional changes that could enhance the immunomodulatory and anti-inflammatory response.
Acknowledgements:
The authors wish to acknowledge the involvement of Amparo Estepa, who passed away, in the generation of data for this article.
Disclosure statement
No potential conflict of interest was reported by the author(s).
Correction Statement
This article has been corrected with minor changes. These changes do not impact the academic content of the article.
Additional information
Funding
References
- Aguzzi, C., Marinelli, O., Zeppa, L., Santoni, G., Maggi, F., & Nabissi, M. (2020). Evaluation of anti-inflammatory and immunoregulatory activities of Stimunex® and Stimunex D3® in human monocytes/macrophages stimulated with LPS or IL-4/IL-13. Biomedicine & Pharmacotherapy, 132, 110845. https://doi.org/10.1016/j.biopha.2020.110845
- Al Aboody, M. S., & Mickymaray, S. (2020). Anti-fungal efficacy and mechanisms of flavonoids. Antibiotics, 9, 45. https://doi.org/10.3390/antibiotics9020045
- Anderson, J., & Paterek, E. (2023, Aug 8). Tonsillitis. In StatPearls [Internet]. StatPearls Publishing. https://www.ncbi.nlm.nih.gov/books/NBK430685/
- Ayala, G., Escobedo-Hinojosa, W. I., de la Cruz-Herrera, C. F., & Romero, I. (2014). Exploring alternative treatments for Helicobacter pylori infection. World Journal of Gastroenterology, 20(6), 1450–1469. https://doi.org/10.3748/wjg.v20.i6.1450
- Baharfar, R., Azimi, R., & Mohseni, M. (2015). Antioxidant and antibacterial activity of flavonoid-, polyphenol- and anthocyanin-rich extracts from Thymus kotschyanus boiss & hohen aerial parts. Journal of Food Science and Technology, 52(10), 6777–6783. https://doi.org/10.1007/s13197-015-1752-0
- Chiancone, F., Carrino, M., Meccariello, C., Pucci, L., Fedelini, M., & Fedelini, P. (2019). The use of a combination of Vaccinium macracarpon, Lycium barbarum L. and probiotics (Bifiprost®) for the prevention of chronic bacterial prostatitis: A double-blind randomized study. Urologia Internationalis, 103(4), 423–426. https://doi.org/10.1159/000502765
- del Carmen Juárez-Vázquez, M., Josabad Alonso-Castro, A., & García-Carrancá, A. (2013). Kaempferitrin induces immunostimulatory effects in vitro. Journal of Ethnopharmacology, 148(1), 337–340. https://doi.org/10.1016/j.jep.2013.03.072
- De Luca, C., Kharaeva, Z., Raskovic, D., Pastore, P., Luci, A., & Korkina, L. (2012). Coenzyme Q10, vitamin E, selenium, and methionine in the treatment of chronic recurrent viral mucocutaneous infections. Nutrition, 28, 509–514. https://doi.org/10.1016/j.nut.2011.08.003
- El-Sayed, S. M., Hassan, K. M., Abdelhamid, A. N., Yousef, E. E., Abdellatif, Y. M., Abu-Hussien, S. H., Nasser, M. A., Elshalakany, W. A., Darwish, D. B. E., Abdulmajeed, A. M., & Alabdallah, N. M. (2022). Exogenous Paclobutrazol reinforces the antioxidant and antimicrobial properties of lavender (Lavandula officinalis L.) oil through modulating its composition of oxygenated terpenes. Plants, 11, 1607. https://doi.org/10.3390/plants11121607
- Ferrara, P., Ianniello, F., Bianchi, V., Quintarelli, F., Cammerata, M., Quattrocchi, E., Terranova, G. M., Miggiano, G. A., & Casale, M. (2021). Beneficial therapeutic effects of vitamin C on recurrent respiratory tract infections in children: Preliminary data. Minerva Pediatrics, 73, 22–27. https://doi.org/10.23736/S2724-5276.16.04621-1
- Ivanov, A. V., Bartosch, B., & Isaguliants, M. G. (2017). Oxidative stress in infection and consequent disease. Oxidative Medicine and Cellular Longevity, 2017, https://doi.org/10.1155/2017/3496043
- Iyer, S. S., & Cheng, G. (2012). Role of interleukin 10 transcriptional regulation in inflammation and autoimmune disease. Critical Reviews™ in Immunology, 32(1), 23–63. https://doi.org/10.1615/CritRevImmunol.v32.i1.30
- Jańczyk, A., Garcia-Lopez, M. A., Fernandez-Peñas, P., Alonso-Lebrero, J. L., Benedicto, I., López-Cabrera, M., & Gonzalez, S. (2007). A Polypodium leucotomos extract inhibits solar-simulated radiation-induced TNF-? and iNOS expression, transcriptional activation and apoptosis. Experimental Dermatology, 16(10), 823–829. https://doi.org/10.1111/j.1600-0625.2007.00603.x
- Jeitler, M., Michalsen, A., Schwiertz, A., Kessler, C. S., Koppold-Liebscher, D., Grasme, J., Kandil, F. I., & Steckhan, N. (2022). Effects of a supplement containing a cranberry extract on recurrent urinary tract infections and intestinal microbiota: A prospective, uncontrolled exploratory study. Journal of Integrative and Complementary Medicine, 28, 399–406. https://doi.org/10.1089/jicm.2021.0300
- Jiang, M. Z., Yan, H., Wen, Y., & Li, X. M. (2011). In vitro and in vivo studies of antioxidant activities of flavonoids from Adiantum capillus-veneris L. African Journal of Pharmacy and Pharmacology, 5, https://doi.org/10.5897/AJPP11.500
- Koradia, P., Kapadia, S., Trivedi, Y., Chanchu, G., & Harper, A. (2019). Probiotic and cranberry supplementation for preventing recurrent uncomplicated urinary tract infections in premenopausal women: A controlled pilot study. Expert Review of Anti-Infective Therapy, 17(9), 733–740. https://doi.org/10.1080/14787210.2019.1664287
- Kutwin, P., Falkowski, P., Łowicki, R., Borowiecka-Kutwin, M., & Konecki, T. (2022). Are we sentenced to pharmacotherapy? Promising role of lycopene and vitamin A in benign urologic conditions. Nutrients, 14, 859. https://doi.org/10.3390/nu14040859
- Lee, S.-H., & Vidal, S. M. (2002). Functional diversity of Mx proteins: Variations on a theme of host resistance to infection. Genome Research, 12(4), 527–530. https://doi.org/10.1101/gr.20102
- Middelkamp-Hup, M. A., Pathak, M. A., Parrado, C., Goukassian, D., Rius-Díaz, F., Mihm, M. C., Fitzpatrick, T. B., & González, S. (2004). Oral Polypodium leucotomos extract decreases ultraviolet-induced damage of human skin. Journal of the American Academy of Dermatology, 51(6), 910–918. https://doi.org/10.1016/j.jaad.2004.06.027
- Murira, A., & Lamarre, A. (2016). Type-I interferon responses: From friend to foe in the battle against chronic viral infection. Frontiers in Immunology, 7.
- Nadjib, B. M. (2020). Effective antiviral activity of essential oils and their characteristic terpenes against coronaviruses: An update. Journal of Pharmacology Clinical Toxicology, 9.
- Nudo, L. P., & Catap, E. S. (2011). Immunostimulatory effects of Uncaria perrottetii (A. Rich.) Merr.(Rubiaceae) vinebark aqueous extract in Balb/C mice. Journal of Ethnopharmacology, 133(2), 613–620. https://doi.org/10.1016/j.jep.2010.10.044
- Panigrahy, D., Gilligan, M. M., Serhan, C. N., & Kashfi, K. (2021). Resolution of inflammation: An organizing principle in biology and medicine. Pharmacology & Therapeutics, 227, 107879. https://doi.org/10.1016/j.pharmthera.2021.107879
- Park, H.-Y., Yu, A.-R., Choi, I.-W., Hong, H.-D., Lee, K.-W., & Choi, H.-D. (2013). Immunostimulatory effects and characterization of a glycoprotein fraction from rice bran. International Immunopharmacology, 17(2), 191–197. https://doi.org/10.1016/j.intimp.2013.06.013
- Piersma, S. J., & Brizić, I. (2022). Natural killer cell effector functions in antiviral defense. The FEBS Journal, 289(14), 3982–3999. https://doi.org/10.1111/febs.16073
- Portillo-Esnaola, M., Rodríguez-Luna, A., Nicolás-Morala, J., Gallego-Rentero, M., Villalba, M., Juarranz, Á, & González, S. (2021). Formation of cyclobutane pyrimidine dimers after UVA exposure (Dark-CPDs) is inhibited by an hydrophilic extract of Polypodium leucotomos. Antioxidants, 10(12), 1961. https://doi.org/10.3390/antiox10121961
- Rösler, D., Fuchs, N., Markolin, G., Edler, B., Kuklinski, B., & Kästenbauer, T. (2012). Reduction of influenza-like infections by a phytoextract-vitamin-combination: A randomized, double-blind, placebo-controlled study. MMW Fortschritte der Medizin, 154(Suppl 4), 115–122.
- Sánchez-Rodríguez, C. (2018). Immunomodulatory effect of Polypodium leucotomos (Anapsos) in child palatine tonsil model. International Journal of Pediatric Otorhinolaryngology, 6.
- Saxena, A., Khosraviani, S., Noel, S., Mohan, D., Donner, T., & Hamad, A. R. A. (2015). Interleukin-10 paradox: A potent immunoregulatory cytokine that has been difficult to harness for immunotherapy. Cytokine, 74(1), 27–34. https://doi.org/10.1016/j.cyto.2014.10.031
- Schink, A., Neumann, J., Leifke, A. L., Ziegler, K., Fröhlich-Nowoisky, J., Cremer, C., Thines, E., Weber, B., Pöschl, U., Schuppan, D., & Lucas, K. (2018). Screening of herbal extracts for TLR2-and TLR4-dependent anti-inflammatory effects. PloS one, 13, e0203907. https://doi.org/10.1371/journal.pone.0203907
- Segars, K., McCarver, V., & Miller, R. A. (2021). Dermatologic applications of Polypodium leucotomos: A literature review. The Journal of Clinical and Aesthetic Dermatology, 14, 50–60.
- Sempere, J. M., Rodrigo, C., Campos, A., Villalba, J. F., & Diaz, J. (1997). Effect of anapsos (Polypodium leucotomos extract) on in vitro production of cytokines. British Journal of Clinical Pharmacology, 43(1), 85–89. https://doi.org/10.1111/j.1365-2125.1997.tb00142.x
- Sempere-Ortells, J. M., Campos, A., Velasco, I., Marco, F., Ramirez-bosca, A., Diaz, J., & Pardo, J. (2002). Anapsos (Polypodium leucotomos) modulates lymphoid cells and the expression of adhesion molecules. Pharmacological Research, 46(2), 185–190. https://doi.org/10.1016/S1043-6618(02)00091-9
- Serhan, C. N. (2017). Treating inflammation and infection in the 21st century: New hints from decoding resolution mediators and mechanisms. The FASEB Journal, 31(4), 1273–1288. https://doi.org/10.1096/fj.201601222R
- Sharma, P., Kaur, J., Sharma, G., & Kashyap, P. (2022). Plant derived antimicrobial peptides: Mechanism of target, isolation techniques, sources and pharmaceutical applications. Journal of Food Biochemistry, 46, https://doi.org/10.1111/jfbc.14348
- Solivellas, B. M., & Martín, T. C. (2012). Polypodium leucotomos extract use to prevent and reduce the risk of infectious diseases in high performance athletes. Infection and Drug Resistance, 5, 149–153. https://doi.org/10.2147/IDR.S29113
- Sun, X., Cameron, R. G., & Bai, J. (2020). Effect of spray-drying temperature on physicochemical, antioxidant and antimicrobial properties of pectin/sodium alginate microencapsulated carvacrol. Food Hydrocolloids, 100, 105420. https://doi.org/10.1016/j.foodhyd.2019.105420
- Vellingiri, B., Jayaramayya, K., Iyer, M., Narayanasamy, A., Govindasamy, V., Giridharan, B., Ganesan, S., Venugopal, A., Venkatesan, D., Ganesan, H., & Rajagopalan, K. (2020). COVID-19: A promising cure for the global panic. Science of The Total Environment, 725, 138277. https://doi.org/10.1016/j.scitotenv.2020.138277
- Wang, L., Song, J., Liu, A., Xiao, B., Li, S., Wen, Z., Lu, Y., & Du, G. (2020). Research progress of the antiviral bioactivities of natural flavonoids. Natural Products and Bioprospecting, 10, 271–283. https://doi.org/10.1007/s13659-020-00257-x
- Wimalawansa, S. J. (2022). Rapidly increasing serum 25(OH)D boosts the immune system, against infections – sepsis and COVID-19. Nutrients, 14(14), 2997. https://doi.org/10.3390/nu14142997
- Xiao, J., & He, W. (2021). The immunomodulatory effects of vitamin D drops in children with recurrent respiratory tract infections. American Journal of Translational Research, 13, 1750–1756.
- Yin, L., Han, H., Zheng, X., Wang, G., Li, Y., & Wang, W. (2019). Flavonoids analysis and antioxidant, antimicrobial, and anti-inflammatory activities of crude and purified extracts from Veronicastrum latifolium. Industrial Crops and Products, 137, 652–661. https://doi.org/10.1016/j.indcrop.2019.04.007
- Zattra, E., Coleman, C., Arad, S., Helms, E., Levine, D., Bord, E., Guillaume, A., El-Hajahmad, M., Zwart, E., van Steeg, H., Gonzalez, S., Kishore, R., & Goukassian, D. A. (2009). Polypodium leucotomos extract decreases UV-induced Cox-2 expression and inflammation, enhances DNA repair, and decreases mutagenesis in hairless mice. The American Journal of Pathology, 175(5), 1952–1961. https://doi.org/10.2353/ajpath.2009.090351
- Zhu, F., Du, B., & Xu, B. (2018). Anti-Inflammatory effects of phytochemicals from fruits, vegetables, and food legumes: A review. Critical Reviews in Food Science and Nutrition, 58(8), 1260–1270. https://doi.org/10.1080/10408398.2016.1251390