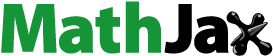
ABSTRACT
A rapid, reliable, sensitive, and multiplex colloidal gold immunochromatographic assay (CGIA) was developed for the simultaneous detection of three polyether ionophore antibiotics (PEs) in chicken muscle. The IC50 values of the optimized CGIA were 1.63, 1.94, and 1.18 ng/mL with a linear range of 0.87–3.04 ng/mL, 0.85–4.41 ng/mL, and 0.57–2.44 ng/mL for maduramicin (MAD), monensin (MON), and salinomycin (SAL), respectively. The cut-off values were 10, 5, and 5 ng/mL, respectively. The whole testing process was completed within 15 min. A parallel analysis in blind chicken muscle samples was conducted by liquid chromatography–tandem mass spectrometry (LC-MS/MS), the developed CGIA did not appear false-positive and false-negative. The results show that the established method could provide a rapid and effective approach for on-site screening and determination of PEs residues in chicken muscle samples.
1. Introduction
Polyether ionophore antibiotics (PEs) are a group of compounds widely used as feed additives to control systemic coccidiosis and change the microbial population of the rumen in food-producing animals, with primary prophylactic use for commercial broiler production (Corah, Citation1991; Dutton et al., Citation1995; Noack et al., Citation2019). Although PEs can effectively treat coccidiosis and facilitate animal growth, toxicity and adverse effects could be potential risks for humans and animals if exposed to low levels of coccidiosis drugs over a long period of time (Dorne et al., Citation2013; Shimshoni et al., Citation2014). Maduramicin is the most toxic of all the PEs for non-target animals and humans (Gao et al., Citation2018). It might cause severe cardiovascular defects, as it inhibits proliferation and induces apoptosis in myoblasts (Chen et al., Citation2014). PEs are also potential emerging groundwater contaminants of concern through excretion in poultry and subsequent land-spreading (Mooney et al., Citation2020). PEs can be absorbed into the human body through edible animal tissues and contaminated water sources, resulting in potential chronic toxicity (Rokka et al., Citation2013). Therefore, monitoring PEs residues in animal-derived food is an important task for human and animal health. It must be assured that the levels of these substances will not exceed the limits introduced in the regulatory document (Sun et al., Citation2023). Hence, maximum residue limits (MRLs) of PEs have been set by many regions (). The European Commission has established MRLs for lasalocid in chicken tissues, for the other PEs MRLs have not been set (The National Archives, Citationn.d.). Compared with other food animals, chickens have superior feed conversion rates, health characteristics (low fat and high protein content), relatively low cost, and quick production time (Kadykalo et al., Citation2018). According to the Food and Agriculture Organization, poultry production is projected to more than double by the year 2050 to fulfill the increasing need for protein by the growing world population (Alexandratos & Bruinsma, Citationn.d.).
Table 1. MRLs of 5 PEs from chicken tissues in different regions.
To monitor PE residue levels in livestock products and guarantee food safety, simple and reliable analytical methods are required. Many analytical methods have been applied to investigate the occurrence of residues of these compounds in different food matrices, including milk, eggs, feed, and tissues (Abafe et al., Citation2020; Silva et al., Citation2020; Silva et al., Citation2021; Wang et al., Citation2010; Wang, Liu, et al., Citation2020). Immunological methods based on specific binding reactions of antibodies and antigens have been rapidly developed for food safety screening (Gu et al., Citation2016). Immunochromatographic assay has been successfully used to monitor many drug residues because of their rapidity, user-friendliness, and sensitivity (Foubert et al., Citation2017; Lee et al., Citation2009; Song et al., Citation2016; Song et al., Citation2019).
This paper developed a multiplex colloidal gold immunochromatographic assay (CGIA) for the simultaneous detection of three PEs in chicken muscle. Three antigens were sprayed onto the nitrocellulose membrane as three test lines to achieve multi-residue detection. To our knowledge, this is the first report of using a single test strip to simultaneously determine Maduramicin (MAD), Monensin (MON), and Salinomycin (SAL) in chicken muscle. This study provided a simple, economical, and rapid method to monitor PEs residue levels to ensure animal-derived food safety.
2. Materials and methods
2.1. Reagents and apparatus
Bovine serum albumin (BSA), goat anti-mouse immunoglobulin G (IgG), Dextran 4000, polyethylene glycol (PEG), chloroauric acid (HAuCl4·4H2O), the analytical standards of 98% lasalocid A sodium salt (LAS) and 98% Narasin (NAR) were purchased from Sigma-Aldrich (St. Louis, MO, USA). The analytical standards of 99.5% Maduramicin ammonium (MAD), 99.5% Monensin sodium (MON), and 99% Salinomycin sodium (SAL) were purchased from Dr. Ehrenstorfer GmbH (Augsburg, German). The 97% Nigericin sodium (NIG) was purchased from Shanghai Yuanye Bio-Technology Co., Ltd (Shanghai, China). The anti-MAD monoclonal antibody (mAb), coating antigens of BSA-conjugated MAD (MAD-BSA) and ovalbumin (OVA)-conjugated salinomycin (SAL-OVA) were preserved in our laboratory (Chen et al., Citation2021; Huang et al., Citation2021). The anti-MON-mAb, MON-BSA conjugate, the anti-SAL-mAb were obtained from Beijing WDWK Biotechnology Co., Ltd. (Beijing, China). The nitrocellulose (NC) membranes were purchased from Sartorius Stedim Biotech (GmbH, Germany). The sample pad, the absorbance pad, and adhesive backing card were supplied by Shanghai Jieyi Biotechnology Co., Ltd. Other chemical substances were purchased from Beijing Chemical Reagent Company (Beijing, China).
The ZX1000 Dispensing Platform and the CM4000 Guillotine Cutting Module were purchased from BioDot Inc. (Irvine, CA, USA). Purified water was obtained using a Milli-Q water purification system (Millipore, Bedford, MA).
2.2. Preparation of colloidal gold nanoparticles
Colloidal gold (CG) was prepared according to the classical sodium citrate reduction method (Li et al., Citation2015; Wang, Wu, et al., Citation2020). All glassware was immersed into aqua regia for 24 h, rinsed several times with ultrapure water, and dried before use. First, 100 mL of ultrapure water was heated to boiling under constant stirring, then 1 mL of 1% chloroauric acid solution (w/v) was added. When the solution was boiling again and then immediately added 2 mL of freshly prepared 1% trisodium citrate solution (w/v) drop-by-drop. The reaction was boiled for another 10 min, and the solution was then cooled to room temperature and reconstituted to the initial volume by adding ultrapure water, and then stored at 4 °C for further use.
The colloidal gold particles were characterized by transmission electron microscopy (Figure S1).
2.3. Preparation of CG-labeled mAb probes
The coupling between the CG and mAbs was based on electrostatic absorption. The proper pH is the key factor in synthesizing CG-labeled mAbs. When the pH of CG solutions is greater than or equal to the isoelectric point of the mAb, the CG-labeled mAb can form a stable colloidal solution (Guo et al., Citation2018; Xu et al., Citation2019).
The three kinds of CG-labeled mAb probes studied in this paper were produced using the same method (Kong et al., Citation2016; Liu et al., Citation2018), as follows: 1 mL of the CG solution was adjusted with 15 μL of 0.1 M K2CO3 (w/v), then 2 μg of anti-MAD mAb was added to pH-adjusted CG solution. The mixture was incubated for 10 min at room temperature, and then 20 μL of 20% BSA (w/v) was added to block for another 10 min, the solution was centrifuged at 12,000 rpm for 15 min at 4°C. Then the CG-labeled mAb precipitate was resuspended with 200 μL of 0.05 M phosphate buffer (PBS, pH 7.4) containing 1% Dextran 4000, 1% BSA, 0.05% PEG, and 0.02% Proclin300. The preparation of another two CG-labeled mAb probes was the same to the above description, just the optimal pH and mAb amount were different. The three prepared CG-labeled mAb probes were mixed at a ratio of 1:2:1 and stored at 4°C for further use.
2.4. Preparation of immunochromatographic test strip
The components of the test strip including NC membrane coated with capture reagents, absorbent pad, sample pad, and adhesive backing card. The capture reagents MAD-BSA (0.79 mg/mL), MON-BSA (0.87 mg/mL), SAL-OVA (1.21 mg/mL) and goat anti-mouse immunoglobulin (0.32 mg/mL) dissolved in 0.01 M phosphate buffer were sprayed onto the NC membrane as the test lines and control line. The sprayed volume of each of the above solutions was 0.8 μL/cm. The prepared NC membrane was then dried overnight at 37°C. Finally, the NC membrane, sample pad, and absorbent pad were laminated and pasted to the adhesive backing card as the test strip, the whole pad was cut into 4.0 mm wide strips and kept dry at room temperature.
2.5. Sample pretreatment
The homogenized chicken muscle sample of approximately 2.00 g was put in a 50-mL centrifuge tube. Then, 15 mL methanol was added, vortexed for 2 min, and followed by ultrasonic treatment in a water bath for 10 min and centrifuged at 5000 rpm for 10 min. Supernatant was dried under nitrogen at 50°C, and reconstituted in 2 mL 0.01 M PBS containing 5% methanol. The recombinant solution was filtered through a 0.22-µm filter membrane for strip detection.
2.6. Test procedure
The principle of the immunoassay procedure is based on the competitive binding of analytes in a sample and coating antigen conjugate to the probes. 200 μL of sample extract solution were added into one well of 96-microtiter plate followed by 8 μL of mixed detection probes with complete mixing. After incubating for 3 min, an immunochromatographic strip was immersed vertically into the corresponding microwell for 15 min. Due to capillary forces and the absorbent pad, the liquid migrates along the NC membrane. The result could be qualitatively judged by the naked eye. If the drug is not present in the sample, the test line area will show a red band because the probe has been captured by the antigen. Otherwise, if any one, two or three of the analytes are present in the sample, the probes will conjugate with the analytes. The intensity of the test line color decreases or disappears as the concentration of drug increases in the sample ().
2.7. Establishment of quantitative calibration curves
To establish the calibration curves, chicken muscle free of MAD, MON and SAL confirmed by LC-MS/MS, was obtained from the National Reference Laboratory for Veterinary Drug Residues (China Agricultural University, Beijing, China). The chicken muscle samples were fortified with three analytes at various concentrations. The final concentrations were as follows: 0, 0.25, 0.5, 1.0, 2.5, 10.0, 25.0, and 50.0 ng/mL for MAD, MON, and SAL, respectively. The ratio of the color intensity of the negative sample test line to the control line was named B0, and the positive sample was named B. The calibration curve was constructed by plotting the B/B0 ratio against the logarithm of target concentrations. Calculations were performed on OrginPro8.5 software (Northampton, MA, USA). A four-parameter logistic equation was used to calculate the obtained data.
A = response value at high asymptote, B = response value at low asymptote, C = concentration corresponding to IC50, D = slope, and X = calibration concentration.
The limit of detection (LOD) and dynamic range represent the calibration concentrations obtained at inhibition rates of 10%, between 20% and 80%, respectively.
2.8. Evaluation of the CGIA
The specificity, stability, accuracy, and precision of the CGIA were evaluated in this work. To evaluate the specificity of the strip, six PEs standard solutions were tested for cross-reactivity by the strip test. The stability was evaluated by an aging test in which the strip was stored under 45°C dry condition, then the color intensity and sensitivity of the strip were analyzed on 7, 14, and 30 days, respectively. For the recovery test, blank chicken muscle samples were spiked with standard solutions to final concentrations of MAD (2.5, 5.0, and 10.0 ng/mL), MON (1.0, 2.5, and 5.0 ng/mL) and SAL (1.0, 2.5, and 5.0 ng/mL). All the spiked samples were pretreated as described in Section 2.5. The detection of drug in the spiked samples was carried out using the CGIA. The accuracy was assessed using the mean recovery results obtained from samples spiked, and precision was expressed as the coefficient of variation (CV, %) of the recovery of samples. Calculate accuracy and precision with five replicates for each spiking level at different time points. Besides, LC-MS/MS analysis was also performed to validate the GICA results. Each test was repeated three times.
3. Results and discussion
3.1. Optimization of the CGIA
The analytical performance of the CGIA could be affected by a variety of conditions, such as the pH value of the CG solution, coating position, the amount of mAb and the usage of the probes (Wang et al., Citation2017). Correct pH value of solution is very important to prepare satisfactory CG-Labeled mAb probe. In this study, the pH of the solution was adjusted by the addition of 0.1 M K2CO3. The results indicated that the pH value of anti-MAD mAb, anti-MON mAb, anti-SAL mAb are suitable when 15, 20, 20 μL 0.1 M K2CO3 are added (Figure S2). The absorbance is greatest when the CG solution reaches its most stable state. For the desired visibility and optimal sensitivity, the amount of mAbs was optimized. A gradient amount of each mAb was added into 1 mL pH-adjusted CG solution, the optimum conditions were obtained based on the T line intensity and inhibition rate. We found that insufficient antibodies result in lower intensity when detecting negative samples, whereas excess antibody reduces the sensitivity (Figure S3a–c). Therefore the optimum amount anti-MAD mAb, anti-MON mAb, anti-SAL mAb are 2 μg, 6 μg, 7.5 μg, respectively. Additionally, three coating antigens were sprayed onto the NC membrane, forming six different position combinations to evaluate the effect of coated position of antigens on sensitivity and color intensity of the test strip. The results showed that there were no sensitivity and color intensity differences between the six position combinations (Figure S4). This may be because each drug has a small molecular weight, no steric hindrance, and good antibody specificity. So, based on personal preferences, MAD-BSA conjugate was sprayed as test line 1, MON-BSA conjugate was sprayed as test line 2, and SAL-OVA conjugate was sprayed as test line 3, respectively. The principle of using probes is to achieve the appropriate color intensity, too many probes will affect sensitivity. Figure S5 showed the optimal usage of three probes, MAD, MON, SAL, which were 2, 4, 2 μL. The optimization of the CGIA conditions was summarized in . Under these optimized conditions, the developed CGIA had clear bands, with high sensitivity.
Table 2. The optimization of the CGIA conditions.
3.2. Establishment of the calibration curves
As shown in , with the increased concentrations of the three analytes, the color intensity of respective test lines decreased. The cut-off value is defined as the lowest concentration in the T line without visible color band. According to the calibration curves (), the limits of detection (LOD), IC50 values and dynamic ranges were calculated. The detail results are shown in . The qualitative and quantitative analysis of this strip met the requirement of the detection levels for MAD, MON, and SAL residues in chicken muscle samples.
Figure 2. Image of the CGIA acquired for 0, 0.25, 0.5, 1.0, 2.5, 10.0, 25.0, and 50.0 ng/mL analytes (including MAD, MON, and SAL); from left to right.

Table 3. Analytical characteristics of standard curves of the CGIA.
3.3. Specificity and stability
The results of cross-reactivity studies demonstrated that both MAD and MON have no cross-reactivity with others (Table S1). But the SAL had cross-reactivity with NAR, this is because the structures of the two drugs are similar (Figure S6). When the concentration of NAR was 10 ng/mL, the test line 3 was weakly positive. This means that the CGIA developed can also analyze NAR in chicken muscle. However, there is not certain whether is SAL or NAR. The results of aging test showed the color intensity and sensitivity remained basically unchanged after 30 days (Table S2). This indicates that the test strips have good stability and can be stored for more than 6 months at room temperature according to the Arrhenius equation (Pei et al., Citation2016).
3.4. Accuracy and precision
The recoveries for the CGIA ranged from 87.9% to 117.8%, with CV less than 14.5% (). For validation of the CGIA, 30 blind chicken muscle samples were used to compare with the reference LC-MS/MS method. CGIA did not give any false-negative or false-positive results (Table S3). These results indicate that the CGIA was well established. Thus the CGIA proposed in this work could be a useful tool for the multiple screening of MAD, MON, and SAL residues in chicken muscle.
Table 4. Recovery and coefficient of variation for the analysis of chicken muscle.
4. Conclusion
In this study, the CGIA was successfully developed for simultaneous detection of three PEs in chicken muscle within 15 min. The developed strip test is multiplex, rapid, reliable, sensitivity, meeting the requirements of on-site and visual inspection. Under optimized conditions, the cut-off values and LODs are lower than the MRLs of many regions. This method provides an analytical detection strategy for the initial screening of three PEs residues in chicken muscle to ensure the development of poultry industry and animal-derived food safety.
Supplemental Material
Download MS Word (9.6 MB)Disclosure statement
No potential conflict of interest was reported by the author(s).
Additional information
Funding
References
- Abafe, O. A., Gatyeni, P., & Matika, L. (2020). A multi-class multi-residue method for the analysis of polyether ionophores, tetracyclines and sulfonamides in multi-matrices of animal and aquaculture fish tissues by ultra-high performance liquid chromatography tandem mass spectrometry. Food Additives & Contaminants: Part A, 37(3), 438–450. https://doi.org/10.1080/19440049.2019.1705399
- Alexandratos, N., & Bruinsma, J. (n.d.). World agriculture towards 2030/2050: The 2012 revision.
- Chen, X., Gu, Y., Singh, K., Shang, C., Barzegar, M., Jiang, S., & Huang, S. (2014). Maduramicin inhibits proliferation and induces apoptosis in myoblast cells. PLoS ONE, 9(12), e115652. https://doi.org/10.1371/journal.pone.0115652
- Chen, Y., Zhao, K., Huang, J., Li, M., Sun, X., & Li, J. (2021). Detection of salinomycin and lasalocid in chicken liver by icELISA based on functional bispecific single-chain antibody (scDb) and interpretation of molecular recognition mechanism. Analytical and Bioanalytical Chemistry, 413(28), 7031–7041. https://doi.org/10.1007/s00216-021-03666-0
- Corah, L. R. (1991). Polyether lonophores — Effect on rumen function in feedlot cattle. Veterinary Clinics of North America: Food Animal Practice, 7(1), 127–132. https://doi.org/10.1016/S0749-0720(15)30813-6
- Dorne, J. L. C. M., Fernández-Cruz, M. L., Bertelsen, U., Renshaw, D. W., Peltonen, K., Anadon, A., Feil, A., Sanders, P., Wester, P., & Fink-Gremmels, J. (2013). Risk assessment of coccidostatics during feed cross-contamination: Animal and human health aspects. Toxicology and Applied Pharmacology, 270(3), 196–208. https://doi.org/10.1016/j.taap.2010.12.014
- Dutton, C. J., Banks, B. J., & Cooper, C. B. (1995). Polyether ionophores. Natural Product Reports, 12(2), 165–181. https://doi.org/10.1039/np9951200165
- Foubert, A., Beloglazova, N. V., Gordienko, A., Tessier, M. D., Drijvers, E., Hens, Z., & Saeger, S. D. (2017). Development of a rainbow lateral flow immunoassay for the simultaneous detection of four mycotoxins. Journal of Agricultural and Food Chemistry, 65(33), 7121–7130. https://doi.org/10.1021/acs.jafc.6b04157
- Gao, X., Peng, L., Ruan, X., Chen, X., Ji, H., Ma, J., Ni, H., Jiang, S., & Guo, D. (2018). Transcriptome profile analysis reveals cardiotoxicity of maduramicin in primary chicken myocardial cells. Archives of Toxicology, 92(3), 1267–1281. https://doi.org/10.1007/s00204-017-2113-8
- Gu, H., Liu, L., Song, S., Kuang, H., & Xu, C. (2016). Development of an immunochromatographic strip assay for ractopamine detection using an ultrasensitive monoclonal antibody. Food and Agricultural Immunology, 27(4), 471–483. https://doi.org/10.1080/09540105.2015.1126808
- Guo, L., Xu, L., Song, S., Liu, L., & Kuang, H. (2018). Development of an immunochromatographic strip for the rapid detection of maduramicin in chicken and egg samples. Food and Agricultural Immunology, 29(1), 458–469. https://doi.org/10.1080/09540105.2017.1401045
- Huang, J., Zhao, K., Li, M., Chen, Y., Liang, X., & Li, J. (2021). Development of an immunomagnetic bead clean-up ELISA method for detection of Maduramicin using single-chain antibody in chicken muscle. Food and Agricultural Immunology, 32(1), 820–836. https://doi.org/10.1080/09540105.2021.1998388
- Kadykalo, S., Roberts, T., Thompson, M., Wilson, J., Lang, M., & Espeisse, O. (2018). The value of anticoccidials for sustainable global poultry production. International Journal of Antimicrobial Agents, 51(3), 304–310. https://doi.org/10.1016/j.ijantimicag.2017.09.004
- Kong, D., Liu, L., Song, S., Suryoprabowo, S., Li, A., Kuang, H., Wang, L., & Xu, C. (2016). A gold nanoparticle-based semi-quantitative and quantitative ultrasensitive paper sensor for the detection of twenty mycotoxins. Nanoscale, 8(9), 5245–5253. https://doi.org/10.1039/C5NR09171C
- Lee, H. J., Cho, S. S., Simkhada, J. R., & Yoo, J. C. (2009). Monoclonal antibody production and immunochemical detection of polyether antibiotics. Archives of Pharmacal Research, 32(3), 437–441. https://doi.org/10.1007/s12272-009-1318-7
- Li, X., Wen, K., Chen, Y., Wu, X., Pei, X., Wang, Q., Liu, A., & Shen, J. (2015). Multiplex immunogold chromatographic assay for simultaneous determination of macrolide antibiotics in raw milk. Food Analytical Methods, 8, 2368–2375. https://doi.org/10.1007/s12161-015-0130-x
- Liu, L., Xu, L., Suryoprabowo, S., Song, S., & Kuang, H. (2018). Development of an immunochromatographic test strip for the detection of ochratoxin A in red wine. Food and Agricultural Immunology, 29(1), 434–444. https://doi.org/10.1080/09540105.2017.1401043
- Mooney, D., Richards, K. G., Danaher, M., Grant, J., Gill, L., Mellander, P.-E., & Coxon, C. E. (2020). An investigation of anticoccidial veterinary drugs as emerging organic contaminants in groundwater. Science of the Total Environment, 746, 141116. https://doi.org/10.1016/j.scitotenv.2020.141116
- Noack, S., Chapman, H. D., & Selzer, P. M. (2019). Anticoccidial drugs of the livestock industry. Parasitology Research, 118(7), 2009–2026. https://doi.org/10.1007/s00436-019-06343-5
- Pei, X., Wang, Q., Li, X., Xie, J., Xie, S., Peng, T., Wang, C., Sun, Y., & Jiang, H. (2016). Provision of ultrasensitive quantitative gold immunochromatography for rapid monitoring of olaquindox in animal feed and water samples. Food Analytical Methods, 9, 1919–1927. https://doi.org/10.1007/s12161-015-0360-y
- Rokka, M., Jestoi, M., & Peltonen, K. (2013). Trace level determination of polyether ionophores in feed. BioMed Research International, 2013, 1–12. https://doi.org/10.1155/2013/151363
- Shimshoni, J. A., Britzi, M., Pozzi, P. S., Edery, N., Berkowitz, A., Bouznach, A., Cuneah, O., Soback, S., Bellaiche, M., Younis, A., Blech, E., Oren, P., Galon, N., Shlosberg, A., & Perl, S. (2014). Acute maduramicin toxicosis in pregnant gilts. Food and Chemical Toxicology, 68, 283–289. https://doi.org/10.1016/j.fct.2014.03.034
- Silva, F. R. N., Bortolotte, A. R., Braga, P. A. D. C., Reyes, F. G. R., & Arisseto-Bragotto, A. P. (2020). Polyether ionophores residues in Minas Frescal cheese by UHPLC-MS/MS. Food Additives & Contaminants: Part B, 13(2), 130–138. https://doi.org/10.1080/19393210.2020.1739149
- Silva, F. R. N., Pereira, M. U., Spisso, B. F., & Arisseto-Bragotto, A. P. (2021). Polyether ionophores residues in pasteurized milk marketed in the state of São Paulo, Brazil: Occurrence and exposure assessment. Food Research International, 141, 110015. https://doi.org/10.1016/j.foodres.2020.110015
- Song, S., Suryoprabowo, S., Liu, L., Kuang, H., Xu, L., Ma, W., & Wu, X. (2019). Development of monoclonal antibody-based colloidal gold immunochromatographic assay for analysis of halofuginone in milk. Food and Agricultural Immunology, 30(1), 112–122. https://doi.org/10.1080/09540105.2018.1550058
- Song, Y., Song, S., Liu, L., Kuang, H., Guo, L., & Xu, C. (2016). Simultaneous detection of tylosin and tilmicosin in honey using a novel immunoassay and immunochromatographic strip based on an innovative hapten. Food and Agricultural Immunology, 27(3), 314–328. https://doi.org/10.1080/09540105.2015.1089843
- Sun, Z., Li, M., Qian, S., Gu, Y., Huang, J., & Li, J. (2023). Development of a detection method for 10 non-steroidal anti-inflammatory drugs residues in four swine tissues by ultra-performance liquid chromatography with tandem mass spectrometry. Journal of Chromatography B, 1223, 123722. https://doi.org/10.1016/j.jchromb.2023.123722
- The National Archives. (n.d.). Commission Directive 2009/8/EC of 10 February 2009 amending Annex I to Directive 2002/32/EC of the European Parliament and of the Council as regards maximum levels of unavoidable carry-over of coccidiostats or histomonostats in non-target feed (Text with EEA relevance). https://Webarchive.Nationalarchives.Gov.Uk/Eu-Exit/Https://Eur-Lex.Europa.Eu/Legal-Content/EN/TXT/?Uri=CELEX:32009L0008; Retrieved May 26, 2023, from https://www.legislation.gov.uk/eudr/2009/8/introduction
- Wang, B., Liu, J., Zhao, X., Xie, K., Diao, Z., Zhang, G., Zhang, T., & Dai, G. (2020). Determination of eight coccidiostats in eggs by liquid–liquid extraction–solid-phase extraction and liquid chromatography–tandem mass spectrometry. Molecules, 25(4), 987. https://doi.org/10.3390/molecules25040987
- Wang, C., Li, X., Peng, T., Wang, Z., Wen, K., & Jiang, H. (2017). Latex bead and colloidal gold applied in a multiplex immunochromatographic assay for high-throughput detection of three classes of antibiotic residues in milk. Food Control, 77, 1–7. https://doi.org/10.1016/j.foodcont.2017.01.016
- Wang, Z., Cheng, L., Shi, W., Zhang, S., & Shen, J. (2010). Fluorescence polarization immunoassay for salinomycin based on monoclonal antibodies. Science China Chemistry, 53(3), 553–555. https://doi.org/10.1007/s11426-010-0085-0
- Wang, Z., Wu, X., Liu, L., Xu, L., Kuang, H., & Xu, C. (2020). Rapid and sensitive detection of diclazuril in chicken samples using a gold nanoparticle-based lateral-flow strip. Food Chemistry, 312, 126116. https://doi.org/10.1016/j.foodchem.2019.126116
- Xu, X., Liu, L., Cui, G., Wu, X., & Kuang, H. (2019). Development of an immunochromatography assay for salinomycin and methyl salinomycin in honey. Food and Agricultural Immunology, 30(1), 995–1006. https://doi.org/10.1080/09540105.2019.1649370