ABSTRACT
Agarwood (Aquilaria crassna) leaves are commonly used as an herbal tea for health supplements. The present study aims to develop the post-harvest process of agarwood leaves using yeast extract (YE) as an elicitor and evaluate its anti-inflammatory and anti-diabetic effects. The results showed that 1% of YE elicitation for 120 min significantly increased genkwanin 5-O-β-primevoside (1.8-fold), mangiferin (1.3-fold), and total benzophenones (1.2-fold) contents over the control group. The phenylalanine ammonia-lyase (PAL) activity increased in maximal at 30 min after YE treatment. The agarwood leaf elicitation with 1% YE for 120 min showed a higher anti-inflammation effect by down-regulation of iNOS, IL-6, and COX-2 in LPS-stimulated RAW264.7 cells and an anti-diabetic effect by enhanced AMPK-α1, AMPK-α2, and GLUT4 in L6 cells over the non-treatment. Our results suggest that YE could be a biotic elicitor to enhance the phytochemical contents and increase the benefit of agarwood leaves as a health supplement.
GRAPHICAL ABSTRACT
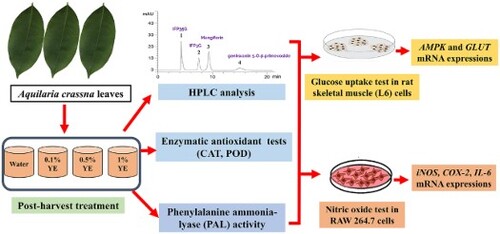
1. Introduction
Nowadays, functional foods such as vegetables and herbs are used in the food supplement industry, the pharmaceutical industry, and the cosmetic product industry. The method of post-harvesting uses a short exposure time to immerse the fresh plant in the elicitor solution. The vegetables and herbs processed during the post-harvest method can increase the production of secondary metabolites (Crupi et al., Citation2014; Temple, Citation2022; Télessy, Citation2019). Improvement of raw material quality after harvest is one strategy for increasing plant value and benefit. For example, extension of time for transfer, maintaining freshness and quality, and enhancement of bioactive compounds (Al-Dairi et al., Citation2021; Buraphaka et al., Citation2022; Liu et al., Citation2023). The dry plants or extracts from the enhancing quality process will have higher prices in the industrial market (Angelova et al., Citation2006; Baenas et al., Citation2014).
Yeast extract (YE) is an elicitor in post-harvested treatment that can act like a pathogen invades the plant cell and stimulates the enzymatic process of plant with biotic components (Sharma et al., Citation2012). Many studies have confirmed that YE has a potential effect on increasing phytochemical components and enzymatic activities in plant cells, such as those of Dregea volubilis, Glycyrrhiza glabra, and Agastache rugosa (Nagarajan et al., Citation2019; Park et al., Citation2016; Vijayalakshmi & Shourie, Citation2019).
Aquilaria crassna Pierre ex Lecomte (AC), or agarwood, is a medicinal plant in the Thymelaeaceae family, and its leaves are commonly used as an herbal tea for a health supplement (Adam et al., Citation2017). The fragrant wood from AC has often been used in Ayurvedic and traditional medicine to treat various illnesses such as joint pain, inflammatory-related disorders, diarrhea, and several others (Hashim et al., Citation2016). In Thailand, agarwood is the main ingredient in the herbal remedy called Yahom, used to treat fainting and abdominal discomfort. The leaves of AC were used as herbal tea, which is marketed in Thailand (Chootip et al., Citation2017).
The pharmaceutical properties of the isolated compounds from AC leaves, such as mangiferin and iriflophenone derivatives (iriflophenone 3-C-β-D-glucoside; IFP3G, iriflophenone 3,5-C-β-D-diglucopyranoside; IFP35G) were shown superior potential on the inhibition of interleukin-1α (IL-1α), interleukin-8 (IL-8), and prostaglandin E2 (PGE2) mediators related to the inflammatory process (Wongwad et al., Citation2019). The ethanolic extract of agarwood leaves showed anti-arthritic activity by dose-dependently reducing the paw edema volume test (Rahman et al., Citation2016). IFP3G and mangiferin have been reported to enhance effects on glucose uptake activity (Pranakhon et al., Citation2015; Wang et al., Citation2014), especially mangiferin that is related to AMP-activated protein kinase (AMPK) and insulin-regulated glucose transporter 4 (GLUT4) mechanisms (Girón et al., Citation2009). Besides, the prevention of cardiovascular diseases by mangferin from AC leaves has been reported (Wisutthathum et al., Citation2019).
However, there is no research study on enhancing the bioactive compounds from agarwood leaves to improve herb quality and functional food after harvest using elicitors. Therefore, this study aims to evaluate the effect of YE elicitor on bioactive components in agarwood leaves after the post-harvested process and then assessed the anti-inflammatory and anti-diabetic activities. The optimal condition can be applied in agriculture fields to produce high-quality raw materials and increase the health value of Thai herbal plants as nutraceutical foods.
2. Materials and methods
2.1. Plant material and post-harvest process
AC leaves were collected on the experiment day (voucher code: NI-PSKKU 128, herbal garden, Faculty of Pharmaceutical Sciences, Khon Kaen University). The mature and undamaged fresh leaves were selected for the post-harvest experiment. The yeast extract (YE; Himedia, India) was prepared to various concentrations (0.1%, 0.5%, and 1%, w/v), and deionized water was used as a control. All reagents were sterilized before use. Elicitation treatment was started by immersing and shaking the AC leaves in the chamber containing the YE elicitor. Then, the sample was collected at 15, 30, 60, and 120 min. After completing the process, the AC leaves were divided into two groups; the first group was dried at 50°C overnight for HPLC analysis and bioactivity assays. The other group was kept at −80°C until used for the enzymatic activity test.
2.2. Sample preparation
The dried YE-treated AC leaves were powdered and accurately weighed to 30 mg per micro-centrifuge tube. Then, 80% methanol (500 µL) was added, and the ultrasonic-assisted process did extraction for 20 min at room temperature. The supernatant was removed from the sample by centrifugation at 5000 rpm for 10 min and placed in the new tube. Ultrasonic-assisted extraction has been replicated four times to combine the extraction yield that proceeds by room temperature dryness and the adjusted concentration into mg/mL by absolute methanol.
2.3. Chromatographic conditions and methods
Quantitative HPLC analysis was performed by Agilent 1200, and the reversed-phase column for analyte separation was Merck LiChrospher 100, C18 (250 × 4 mm, 5 μm). The mobile phase system was followed by the previous method with minor modifications using isocratic conditions (15% acetonitrile in 0.01% acetic acid) with a flow rate of 0.5 mL/min (Putalun et al., Citation2013). The detector wavelength was 330 nm. For reference standards, iriflophenone 3-C-β-glucoside (IFP3G), iriflophenone 3,5-C-β-d-diglucopyranoside (IFP35G), genkwanin 5-O-β-primevoside, and mangiferin were obtained from Associate Professor Boonchoo Sritularuk, Faculty of Pharmaceutical Sciences, Chulalongkorn University, Bangkok, Thailand. The validation process was prepared by mixing four reference compounds to obtain solutions with final concentrations of 200.00, 100.00, 50.00, 25.00, 12.50, 6.25, and 3.13 µg/mL for each compound. The validation results, including linearity, the limit of detection (LOD), the limit of quantification (LOQ), and correlation coefficients, are exhibited in Table S1.
2.4. Enzymatic assays
2.4.1. Phenylalanine ammonia-lyase (PAL) activity
The protocol was followed as described in the previous study (Buraphaka & Putalun, Citation2020). The frozen samples (0.5 g) were ground to powder by liquid nitrogen on the chilled mortar, then Tris-HCl buffer (25 mM, pH 8.5) was added and homogenized. The protein extract solution was separated from the plant fibres by centrifugation at 13,500 rpm at 4°C for 20 min. The protein yield was determined by Bradford assay and calculated as mg protein. After that, the extract was stored at 4°C until it was used to determine the PAL and CAT assays.
For the PAL (EC 4.3.1.5) assay, the plant protein was mixed with L-phenylalanine (150 mM) and incubated at 37°C for 1 h. Then, the enzymatic reaction was finished by hydrochloric acid (6 M) and trans-cinnamic acid, and the final product from the PAL assay was separated by toluene partition. The toluene phase was collected, and a UV spectrophotometer measured the production of trans-cinnamic acid at 290 nm. The data were calculated by comparing them with a standard curve of trans-cinnamic acid. The PAL activity was expressed as μg trans-cinnamic acid/mg protein/hour.
2.4.2. Catalase (CAT) activity
The CAT (EC 1.11.1.6) protocol was followed as described in the previous study (Buraphaka & Putalun, Citation2020). The extraction process was the same as the PAL assay, as previously described. The plant protein was combined with sodium phosphate buffer (50 mM, pH 7.0) and H2O2 (130 mM). Then, it was incubated at 37°C for 1 min, and the reaction was finished by adding ammonium molybdate (32.4 mM). The final product from the enzymatic reaction was determined by a UV spectrophotometer at 405 nm. The CAT activity results were reported as U/mg protein.
2.4.3. Peroxidase (POD) activity
The POD (EC1.11.1.7) protocol was followed as described in the previous study (Buraphaka & Putalun, Citation2020). The frozen samples (0.5 g) were ground to powder by liquid nitrogen on the chilled mortar, then potassium phosphate buffer (40 mM, pH 5) was added and homogenized. The protein extract solution was separated from the plant fibres by centrifugation at 13,500 rpm at 4°C for 20 min. The protein yield was determined by Bradford assay and calculated as mg protein. After that, the extract was stored at 4°C until it was used.
The plant extract was mixed with H2O2 (1%) and 2, 2′-azino-bis-3-ethylbenzthiazoline-6-sulphonic acid (10 mM). Then, it was incubated at 37°C for 1 min and measured by a UV spectrophotometer at 405 nm. The data were calculated by comparing them with a standard curve of peroxidase (U/min). The POD activity results were reported as U/mg protein/min.
2.5. Anti-inflammatory assay in macrophage RAW267.4 cell culture
According to the previous study (Buraphaka et al., Citation2022), the anti-inflammatory activity was performed on macrophage RAW267.4 cells by measuring nitric oxide. The extracts were dissolved in DMSO and diluted in Delbecco's modified eagle's medium (DMEM; Gibco, USA) by fixing the final concentration at not over 0.1% in a medium. Lipopolysaccharide (LPS) from Escherichia coli 0111:B4 (Sigma-Aldrich, USA) was used as an inflammatory stimulant at a concentration of 0.1 μg/mL, and Nω-Nitro-L-arginine methyl ester hydrochloride (L-NAME; Sigma-Aldrich, USA) was used as a positive control in all experiments.
2.6. Anti-diabetic assay in rat skeletal muscle (L6) cell culture
According to the previous study (Choonong et al., Citation2021), the glucose uptake stimulation activity was performed on rat myotube (L6) cells by measuring the concentration of glucose in the cell medium. The extracts were dissolved in DMSO and diluted in the minimum essential medium eagle alpha modification (α-MEM; Sigma-Aldrich, USA) by fixing the final concentration at not over 0.1% in a medium. Insulin (500 nM, Biocon, India) and metformin (2 nM, Sigma-Aldrich, USA) were used as the positive control experiments. The result has been calculated as the percentage of glucose uptake (% of glucose uptake).
2.7. Cell viability
After completing the anti-inflammatory test in RAW267.4 cells and the anti-diabetic assay in rat myotube (L6) cells, the cell medium was removed and the cells were cleaned with sterile PBS buffer (pH 7.0). The cell viability test was performed using 3-(4.5-dimethylthiazole-2-yl)−2,5-diphenyl tetrazolium bromide (MTT; Sigma-Aldrich, USA) solution (5 mg/mL) and incubating the treated cells for 2 h at 37°C. After that, the MTT solution was discarded. The formazan form, which resulted from the MTT reaction, was dissolved by isopropanol AR and determined at 595 nm. The absorbance of formazan was calculated by comparing it with the non-treatment group and reported as the percentage of cell viability.
2.8. RNA extraction and cDNA synthesis
The treated cells were extracted with TRIzol reagent (Invitrogen, USA) by precipitating total RNA. After the homogenizing process, the protein partition was performed by adding chloroform. Then, the aqueous layer, which contained RNA was separated into a new clean tube. The RNA was purified by isopropanol and 75% ethanol, respectively. The RNA concentration was determined by BioDrop (Biochrom, UK), and all RNA samples were diluted with nuclease-free water at the same final concentration. Then, the cDNA synthesis was performed by ReverTra Ace qPCR RT Master Mix (Toyobo, Japan). The diluted RNA sample was mixed with the 5X RT master mix and processed according to the manufacturer protocol. The finished cDNA was kept at −80°C until used for real-time PCR analysis.
2.9. Gene expression analysis by real-time-PCR
The gene expression was performed by real-time PCR with specific primers (Table S2), The β-actin was chosen as the reference gene for RAW 264.7 cells, and GADPH was selected as the reference gene for L6 cells. The cDNA sample was mixed with the specific primer and qPCR Mix (THUNDERBIRD™ SYBR®, Toyobo, Japan) following the manufacturer protocol. The qPCR was performed by Biorad-iQ5 (Biorad, USA) with the following condition: 95°C for 3 min, 40 cycles of 20 s at 95°C, 20 s at 56°C, and 30 s at 72°C. Relative gene expression was calculated by the 2−ΔΔCt method to compare with the reference gene. The data was expressed as fold expression change relative to the control group.
2.10. Statistical analysis
The chemical contents from HPLC, enzymatic activities, and real-time PCR results were performed in triplicate. Besides, the nitric oxide suppressive activity of RAW 264.7 cells, the glucose uptake activity of rat myotube (L6) cells, and the cell viability of RAW 264.7 and L6 cells were performed in triplicate. The data were analysed with SPSS version 26 software (IBM Corp., USA), and the data were expressed as mean ± SD. The statistical significance analysis was calculated by using an analysis of variance (ANOVA) followed by Dunnett’s test (p < 0.05).
3. Results and discussion
3.1. Effect of YE on chemical contents in agarwood leaves
The developed HPLC method for detection of major chemical constituents in AC leaves, including IFP35G, IFP3G, mangiferin, and genkwanin 5-O-β-primevoside was simultaneously determined in AC leaves (). The YE-treated AC leaves with various concentrations (0.1%, 0.5%, and 1%) of YE for 15, 30, 60, and 120 min were analysed and the content interpreted into mg/g dry weight. As a result, the chemical contents at different times and concentration treatments were different, as shown by the dynamic change of phytochemical compounds in Figure S1. The genkwanin 5-O-β-primevoside content was increased following elicitor concentration and exposure time. The superior genkwanin 5-O-β-primevoside content was shown at 1% of YE elicitation for 120 min (135.01 ± 1.12 mg/g dry weight), and 0.5% of YE elicitation for 120 min (119.01 ± 2.83 mg/g dry weight), increasing up to 1.8-fold and 1.4-fold from control at the same condition, respectively (C). For total benzophenones, including IFP35G and IFP3G, the elicitation with 1% of YE for 120 min showed the highest total benzophenones (57.08 ± 2.82 mg/g dry weight) increased up to 1.2-fold from control at the same condition (A). For mangiferin, the elicitation with 1% of YE for 120 min increased up to 1.3-fold compared with the control group at the same condition (20.97 ± 0.75 mg/g dry weight) (B). Many studies showed that YE could enhance the secondary metabolites in plants, including natural phenols, such as kaempferol (Nagarajan et al., Citation2019), licochalcone A (Vijayalakshmi & Shourie, Citation2019), and rosmarinic acid (Park et al., Citation2016). Our results indicate that YE can elicit the secondary metabolites in agarwood leaves over the control. As a result, the suitable condition was 0.5% - 1% of YE for 120 min.
Figure 1. The HPLC chromatograms of (A) reference standards chemical in AC leaves (1–3; 25 µg/mL, 4; 100 µg/mL), (B) the methanol extract from the AC leaves control group, (C) the methanol extract from the AC leaves elicitation with 1% yeast extract for 120 min. (1) iriflophenone 3,5-C-β-d-diglucopyranoside (IFP35G), (2) iriflophenone 3-C-β-glucoside (IFP3G), (3) mangiferin, and (4) genkwanin 5-O-β-primevoside.

Figure 2. The chemical contents of A. crassna leaves after treated with yeast extract (YE). (A) total benzophenones, (B) mangiferin, and (C) genkwanin 5-O-β-primevoside. Values were showed means ± SD (mg/g dry weight). * p < 0.05 compared with control group in the same condition. iriflophenone 3-C-β-glucoside (IFP3G), iriflophenone 3,5-C-β-d-diglucopyranoside (IFP35G).

3.2. Effect of YE on phenylalanine ammonia-lyase (PAL) activity
The PAL activity was determined by trans-cinnamic acid production. Overall, in PAL activity (), trans-cinnamic acid production increased and reached maximum at 30 min. After that, it decreased at a later time. The elicitation with 1% YE for 30 min showed the highest trans-cinnamic acid production, about 2-fold over control at the same condition (6.95 ± 0.03 µg trans-cinnamic acid/mg protein/hr). This data indicates that the YE elicitor triggered plant biosynthesis pathway. YE is a biotic elicitor that contains many substances to accelerate secondary metabolites. PAL is the first enzyme in the plant biosynthesis pathway and continued to other enzymes and secondary metabolites in the process of biosynthesis pathway (Joubert et al., Citation2014). In this experiment, the maximum PAL activity was observed at 30 min after YE treatment on AC leaves. Then, the secondary metabolites in AC leaves increased at 120 min treatment. This result can be explained by plant biosynthesis of AC, which is related to flavonoid biosynthesis, and it might pass through many intermediate substances in the pathway and result in genkwanin 5-O-β-primevoside production (Liu et al., Citation2021). Our result correlated with the other study, in which YE triggered PAL activity. For example, in the post-elicitation effect of YE in apple cell suspension cultures, PAL activity was enhanced in a short time and directed toward enhanced polyphenolic acid biosynthesis at a later time (Sarkate et al., Citation2017). These supported the finding that PAL activity showed a correlation with a high chemical constituent in AC leaves.
Figure 3. The phenylalanine ammonia-lyase (PAL) activity of YE-treated leaves. Values were showed means ± SD of three replicates (µg trans-cinnamic acid/mg protein/hr). * p < 0.05; ** p < 0.01 compared with control group in the same condition; significant level were determined by One-way ANOVA, Dunnett’s test.

3.3. Effect of YE on antioxidant system activity
The POD and CAT activities showed a similar response to YE following concentration and time. At first, after 15 min, the POD activity increased in every treatment; then it dropped after 30, 60, and 120 min (A). Similarly, with CAT, the enzymatic activity exhibited the highest activity at 15–30 min of treatment time. After that, it shuts down at a later time (B). This result correlated with the other study. The YE can trigger the plant enzymatic system in the antioxidant process because some biotic components in the YE can act like pathogens that invade the plant cell (Sharma et al., Citation2012). For example, CAT activity has increased in Catharanthus roseus cultivating tissue under the YE stress condition (Maqsood & Abdul, Citation2017). POD activity was exhibited in Levisticum officinale pre-harvest with 0.1% YE (Złotek et al., Citation2020). In AC leaves, the YE elicitor at a concentration in the range of 0.1–1% is suitable for inducing oxidative stress in plants.
Figure 4. The enzymatic activities of YE-treated leaves. (A) catalase (CAT) activity, and (B) peroxidase (POD) activity. Values were showed means ± SD (mg/g dry weight) of three replicates (Unit/g protein/min). * p < 0.05; ** p < 0.01 compared with control group in the same condition; significant level were determined by One-way ANOVA, Dunnett’s test.

3.4. Effect of agarwood leaves treatment on cell viability in RAW264.7 cells
The cytotoxicity of AC leaves in RAW264.7 cells was determined by MTT assay, and the percentage of cell viability is shown in Figure S2A. The percentage of cell viability treated with 0.1 µg/mL LPS was 100% viability and had a non-toxic effect on the cell at the same condition. The reference compounds in AC leaves, IFP35G, IFP3G, mangiferin, and genkwanin 5-O-β-primevoside at doses of 20, 10, and 5 µg/mL showed a percentage of cell viability of 90–100%. The selected plant from the post-harvest process in this toxicity experiment was 1% and 0.5% of YE for 120 min AC leaf extracts. The optimal plant extract at the concentrations of 80, 40, and 20 µg/mg dry weight showed more than 80% cell viability. Therefore, the treated AC leaves with YE had no cytotoxic effect on RAW264.7 cells.
3.5. Effect of agarwood leaves treatment on nitric oxide (NO) production in RAW264.7 cells
The YE-treated AC leaves candidate for evaluation of anti-inflammatory effects in RAW264.7 cells because of the superior total benzophenones, mangiferin, and genkwanin 5-O-β-primevoside content. According to the HPLC results (), AC leaves treated with 0.5% and 1% of YE for 120 min were selected to evaluate NO production due to the high level of genkwanin 5-O-β-primevoside was obtained. The reference compounds in AC leaves, IFP35G, IFP3G, mangiferin, and genkwanin 5-O-β-primevoside at the doses 20, 10, and 5 µg/mL were evaluated. The results showed that at the dose of 20 µg/mL of all reference compounds, NO production was significantly reduced compared with 0.1 µg/mL LPS. Moreover, IFP35G and genkwanin 5-O-β-primevoside showed a superior NO inhibition effect over IFP3G and mangiferin at the same concentration (A). The optimal concentration of plant extracts with no cytotoxicity at doses of 80, 40, and 20 µg/mg dry weight showed NO inhibition. The elicitation leaves with 1% YE for 120 min showed a significant reduction in NO compared with non-treated leaf extract at 80 and 40 µg/mg dry weight (B). The previous study supported this result; the isolated compound from AC leaves exhibited an anti-inflammatory effect via NO reduction in RAW264.7 cell culture (Wongwad et al., Citation2019).
Figure 5. Effect of reference standards chemical in AC leaves and YE-treated leaves extracts on NO production in LPS-stimulated RAW 264.7 cells. (A) Cells were treated with difference concentrations of reference standards, control group were obtained in the absence of LPS, and positive group was treated with L-NAME. (B) Cells were treated with difference concentration of YE-treated leaves extracts. * p < 0.05 compared with LPS (+) group, # p < 0.05 compared with AC leaves extract in each control at same condition; significant level were determined by One-way ANOVA, Dunnett’s test.

3.6. Effect of agarwood leaves treatment on mRNAs related inflammation gene expression
In the RAW macrophage cell model, it mainly processes inflammatory mediation with NO. Inducible nitric oxide synthase (iNOS) is directly related to evaluating anti-inflammatory activity; a high level of iNOS expression indicates a high inflammatory process (Sonar & Lal, Citation2019; Xue et al., Citation2018). Interleukin 6 (IL-6) and cyclooxygenase-2 (COX-2) are alternative mechanisms in the inflammatory process. IL-6 is a proinflammatory cytokine that is produced predominantly by activated macrophages and is involved in the up-regulation of inflammatory reactions. Besides, COX-2 is an important enzyme in prostaglandin biosynthesis, which is related to long-term inflammation (Ayoub et al., Citation2020).
The AC leaves with elicitation with 1% of YE for 120 min, which contain 1.8-fold more genkwanin 5-O-β-primevoside content than the control group, were selected to evaluate iNOS, IL-6, and COX-2 gene expression in RAW264.7 cell culture. The results showed that 0.1 µg/mL of LPS could up-regulate iNOS, IL-6, and COX-2 expression levels (318-fold, 123-fold, and 43-fold, respectively) significantly when compared with the non-treated group (). The AC leaves with 1% of YE for 120 min showed dose-dependent inhibition of iNOS, IL-6, and COX-2 expression levels. Besides, the high dose treatment at 80 µg/mg dry weight significantly inhibited iNOS, IL-6, and COX-2 expression as well as that of a positive control (L-NAME, 50 µg/mL). However, the housekeeping gene as β-actin did not change in level of expression when the cell was treated with the AC leaf extract. Furthermore, an aglycone form of genkwanin has been reported to have a down-regulation effect on the mRNA expression of iNOS, COX-2, TNF-α, IL-1β, and IL-6 (Gao et al., Citation2014; Sun et al., Citation2020). Mangiferin also exhibited pro-inflammatory mediator inhibition effects (COX-2, iNOS, and TNF-α) in LPS-stimulated RAW 264.7 cells (Bulugonda et al., Citation2017). Our finding could imply that a high content of genkwanin 5-O-β-primevoside in the elicitation of AC leaves has correlated with a down-regulation level of iNOS, IL-6, and COX-2, which exhibited a superior anti-inflammatory effect.
Figure 6. Real time-PCR analysis of the AC leaves extracts on anti-inflammation specific gene expressed as fold expression in RAW264.7 cells. (A) iNOS, (B) COX-2, and (C) IL-6. Data were expressed as fold expression, * p < 0.05 compared with LPS (+) group, # p < 0.05 compared with AC leaves extract in each control at same condition; significant level were determined by One-way ANOVA, Dunnett’s test.

3.7. Effect of agarwood leaves treatment on cell viability in rat skeletal muscle (L6) cells
The cytotoxicity of AC leaves in rat skeletal muscle (L6) cells was determined by MTT assay, and the percentage of cell viability is shown in Figure S2B. The percentage of cell viability treated with 0.1% DMSO has been calculated to be 100% cell viability, which implies a non-toxic effect on a cell. The reference compounds in AC leaves, IFP35G, IFP3G, and mangiferin at the dose 200, 100, and 50 µg/mL and genkwanin 5-O-β-primevoside at the doses 100, 50, and 25 µg/mL showed a percentage of cell viability of 85–100%. The AC leaves from the post-harvest process in this toxicity experiment were 1% and 0.5% YE for 120 min AC leaf extracts. The optimal plant extract at the concentrations of 400, 200, and 100 µg/mg dry weight showed a percentage of cell viability over 90%. Thus, the treated AC leaves with YE had no cytotoxic effect on rat skeletal muscle (L6) cells.
3.8. Effect of agarwood leaves treatment on glucose uptake activity in rat skeletal muscle (L6) cells
The non-toxicity concentrations of reference compounds and YE-treated AC leaf extracts were evaluated on rat skeletal muscle (L6) cell cultures overnight. The results of all experiments are shown in . The reference compounds groups IFP35G, IFP3G, and mangiferin at the doses 200, 100, and 50 µg/mL and genkwanin 5-O-β-primevoside at the doses 100, 50, and 25 µg/mL were shown to be concentration-related to glucose uptake activity. The highest dose (200 µg/mL) treatment of four compounds could enhance glucose uptake by 119.99 ± 2.66, 127.90 ± 2.12, 133.37 ± 5.02, and 131.03 ± 3.91%, respectively. For AC leaves, the post-harvest treatment with 0.5% and 1% YE for 120 min was selected and compared with the non-YE treatment as a control. The non-toxic doses at 400, 200, and 100 µg/mL of selected AC leaves showed glucose uptake activity in . As a result, the highest dose of the AC control group exhibited glucose uptake of 119.34 ± 4.90%. In comparison, the AC leaves treated with 0.5% and 1% YE for 120 min at the same concentration could enhance glucose uptake by 133.12 ± 4.60 and 134.56 ± 3.18%, respectively, which showed superior glucose uptake activity.
Table 1. Glucose uptake stimulatory activity of reference standards chemical in AC leaves and YE-treated AC leaves.
Our result correlated with the other study, such as the isolated compounds from AC leaves, IFP3G at 2.5 µM could enhance glucose uptake by 154.6 ± 11.2% on rat adipocyte cells (Pranakhon et al., Citation2015). Besides, mangiferin at 40 µM increased glucose consumption by 113% compared with basal cells (Wang et al., Citation2014).
3.9. Effect of agarwood leaves treatment on mRNAs related glucose uptake gene expression
The selected concentrations of 200 µg/mL of IFP35G, IFP3G, mangiferin, and 100 µg/mL of genkwanin 5-O-β-primevoside were evaluated on glucose uptake-related gene expression in L6 cells. The specific gene expression of AMPK-α1, AMPK-α2, GLUT1, and GLUT4 has been observed in this experiment. The result is exhibited in . Overall, IFP35G and mangiferin could significantly enhance all gene expressions over the control. Genkwanin 5-O-β-primevoside significantly increased only AMPK-α1, AMPK-α2, and GLUT4, while IFP35G significantly enhanced only AMPK-α1. The result correlated with previous studies showing that mangiferin could enhance AMPK (Wang et al., Citation2014) and GLUT4 (Girón et al., Citation2009).
Figure 7. Relative expression of (A) AMPK-α1, (B) AMPK-α2, (C) GLUT1 and (D) GLUT4 in rat L6 myotube cells after 24 h treatment. Data were expressed as fold expression, and * p < 0.05 compared with control group; # p < 0.05 compared with AC leaves extract control group; significant level were determined by One-way ANOVA, Dunnett’s test.

The AC leaves treated with 1% YE for 120 min was selected for glucose uptake-related gene expression. The relative expression of AMPK-α1, AMPK-α2, GLUT1, and GLUT4 is shown in . When compared with non-treatment AC leaves as the control group, the AC leaves with 1% YE for 120 min could significantly enhance AMPK-α1, AMPK-α2, and GLUT4, but there was no significant difference on GLUT1. According to the HPLC results, the 1% YE-treated AC leaves for 120 min contained 1.8-fold of genkwanin 5-O-β-primevoside and 1.3-fold of mangiferin, both reference compounds potentially enhance AMPK-α1, AMPK-α2, and GLUT4 mRNA expression on L6 cells. Thus, the higher glucose uptake effect of the YE-treated AC leaves could be correlated with the high content of genkwanin 5-O-β-primevoside and mangiferin.
4. Conclusion
Our study revealed the YE effect on the enhancement of phytochemical compounds, including IFP35G, IFP3G, mangiferin, and genkwanin 5-O-β-primevoside after post-harvested treatment. The AC leaves treated with 1% YE for 120 min could increase genkwanin 5-O-β-primevoside (1.8-fold), mangiferin (1.3-fold), and total benzophenones (1.2-fold) contents over the control group. The effect of YE treatment to enhance the secondary metabolites was correlated with PAL activity in the enzymatic biosynthesis pathway and antioxidant enzymatic activity. Moreover, AC leaf after treatment with 1% YE for 120 min showed a higher anti-inflammation effect by down-regulating iNOS, IL-6, and COX-2 in LPS-stimulated RAW264.7 cells and an anti-diabetic effect by enhancing AMPK-α1, AMPK-α2, and GLUT4 in rat skeletal muscle (L6) cells over the non-treatment AC leaves. Therefore, YE is an interesting elicitor that triggers plant antioxidant and PAL enzymatic activities in a short time, not over 2 h. It can increase genkwanin 5-O-β-primevoside content and improve both anti-inflammatory and anti-diabetic effects. Our finding indicates that YE at optimal concentration can be applied for post-harvest treatment of agarwood leaves to enhance the health-promoting effect of natural products or plant raw materials.
Acknowledgements
Author contributions: R.C., J.J., V.W., and K.B. performed the experiments. R.C. participated in data analysis and wrote the manuscript. B.S. provided reference compounds. W.P. conceived and designed the experiment and approved the final draft of the manuscript. All authors reviewed and approved the manuscript.
Disclosure statement
No potential conflict of interest was reported by the author(s).
Additional information
Funding
References
- Adam, A. Z., Lee, S. Y., & Mohamed, R. (2017). Pharmacological properties of agarwood tea derived from Aquilaria (Thymelaeaceae) leaves: An emerging contemporary herbal drink. Journal of Herbal Medicine, 10, 37–44. https://doi.org/10.1016/j.hermed.2017.06.002
- Al-Dairi, M., Pathare, P. B., & Al-Yahyai, R. (2021). Effect of postharvest transport and storage on color and firmness quality of tomato. Horticulturae, 7(7), 163. https://doi.org/10.3390/horticulturae7070163
- Angelova, Z., Georgiev, S., & Roos, W. (2006). Elicitation of plants. Biotechnology & Biotechnological Equipment, 20(2), 72–83. https://doi.org/10.1080/13102818.2006.10817345
- Ayoub, S., Berbéri, A., & Fayyad-Kazan, M. (2020). Cytokines, masticatory muscle inflammation, and pain: An update. Journal of Molecular Neuroscience, 70(5), 790–795. https://doi.org/10.1007/s12031-020-01491-1
- Baenas, N., García-Viguera, C., & Moreno, A. D. (2014). Elicitation: A tool for enriching the bioactive composition of foods. Molecules, 19(9), 13541–13563. https://doi.org/10.3390/molecules190913541
- Bulugonda, R. K., Kumar, K. A., Gangappa, D., Beeda, H., Philip, G. H., Muralidhara Rao, D., & Faisal, S. M. (2017). Mangiferin from Pueraria tuberosa reduces inflammation via inactivation of NLRP3 inflammasome. Scientific Reports, 7(1), 42683–42683. https://doi.org/10.1038/srep42683
- Buraphaka, H., & Putalun, W. (2020). Stimulation of health-promoting triterpenoids accumulation in Centella asiatica (L.) urban leaves triggered by postharvest application of methyl jasmonate and salicylic acid elicitors. Industrial Crops and Products, 146, 112171. https://doi.org/10.1016/j.indcrop.2020.112171
- Buraphaka, H., Puttha, W., & Putalun, W. (2022). Comparative evaluation of antioxidant and anti-inflammatory activity of active compounds identified in Ardisia elliptica extracts from different plant parts. Chemistry & Biodiversity, 19(2), e202100796. https://doi.org/10.1002/cbdv.202100796
- Choonong, R., Jabsanthia, J., Waewaram, V., Panjanghan, K., & Putalun, W. (2021). Comparative study of callus culture and leaves of Thunbergia laurifolia for their bioactive constituents and the activation of AMPK and GLUT-dependent glucose uptake on rat skeletal muscle (L6) cells. Journal of Food Processing and Preservation, 45(5), e15434. https://doi.org/10.1111/jfpp.15434
- Chootip, K., Chaiyakunapruk, N., Soonthornchareonnon, N., Scholfield, C. N., & Fuangchan, A. (2017). Efficacy and safety of “Yahom” as a traditional Thai herbal therapy: A systematic review. Journal of Ethnopharmacology, 196, 110–123. https://doi.org/10.1016/j.jep.2016.12.002
- Crupi, P., Pichierri, A., Milella, R., Perniola, R., & Antonacci, D. (2014). Role of the physical elicitors in enhancing postharvest antioxidant capacity of table grape cv redglobe (Vitis vinifera L.). Journal of Food Research, 3(2), 61–70. https://doi.org/10.5539/jfr.v3n2p61
- Gao, Y., Liu, F., Fang, L., Cai, R., Zong, C., & Qi, Y. (2014). Genkwanin inhibits proinflammatory mediators mainly through the regulation of miR-101/MKP-1/MAPK pathway in LPS-activated macrophages. PloS One, 9(5), e96741. https://doi.org/10.1371/journal.pone.0096741
- Girón, M. D., Sevillano, N., Salto, R., Haidour, A., Manzano, M., Jiménez, M. L., Rueda, R., & López-Pedrosa, J. M. (2009). Salacia oblonga extract increases glucose transporter 4-mediated glucose uptake in L6 rat myotubes: Role of mangiferin. Clinical Nutrition, 28(5), 565–574. https://doi.org/10.1016/j.clnu.2009.04.018
- Hashim, Y. Z. H.-Y., Kerr, P. G., Abbas, P., & Mohd Salleh, H. (2016). Aquilaria spp. (Agarwood) as source of health beneficial compounds: A review of traditional use, phytochemistry and pharmacology. Journal of Ethnopharmacology, 189, 331–360. https://doi.org/10.1016/j.jep.2016.06.055
- Joubert, E., de Beer, D., Hernández, I., & Munné-Bosch, S. (2014). Accummulation of mangiferin, isomangiferin, iriflophenone-3-C-β-glucoside and hesperidin in honeybush leaves (Cyclopia genistoides Vent.) in response to harvest time, harvest interval and seed source. Industrial Crops and Products, 56, 74–82. https://doi.org/10.1016/j.indcrop.2014.02.030
- Liu, W., Feng, Y., Yu, S., Fan, Z., Li, X., Li, J., & Yin, H. (2021). The flavonoid biosynthesis network in plants. International Journal of Molecular Sciences, 22(23), 12824. https://doi.org/10.3390/ijms222312824
- Liu, Z., Hu, T., Yu, C., Tan, W., Shen, Y., & Duan, L. (2023). Design of aminoethoxyvinylglycine functional analogues to delay postharvest ripening of tomato fruit. Postharvest Biology and Technology, 195, 112096. https://doi.org/10.1016/j.postharvbio.2022.112096
- Maqsood, M., & Abdul, M. (2017). Yeast extract elicitation increases vinblastine and vincristine yield in protoplast derived tissues and plantlets in Catharanthus roseus. Revista Brasileira de Farmacognosia, 27(5), 549–556. https://doi.org/10.1016/j.bjp.2017.05.008
- Nagarajan, Y., Bhakyaraj, R., Syed Ali, M., & Muthezhila, R. (2019). Effect of yeast elicitor on the enhancement of Kaempferol from in vivo and in vitro callus cultures of Dregea volubilis benth. Asian Journal of Biological Sciences, 12(2), 278–283. https://doi.org/10.3923/ajbs.2019.278.283
- Park, W. T., Arasu, M. V., Al-Dhabi, N. A., Yeo, S. K., Jeon, J., Park, J. S., Lee, S. Y., & Park, S. U. (2016). Yeast extract and silver nitrate induce the expression of phenylpropanoid biosynthetic genes and induce the accumulation of Rosmarinic acid in Agastache rugosa cell culture. Molecules, 21(4), 426. https://doi.org/10.3390/molecules21040426
- Pranakhon, R., Aromdee, C., & Pannangpetch, P. (2015). Effects of iriflophenone 3-C-β-glucoside on fasting blood glucose level and glucose uptake. Pharmacognosy Magazine, 11(41), 82–89. https://doi.org/10.4103/0973-1296.149711
- Putalun, W., Yusakul, G., Saensom, P., Sritularak, B., & Tanaka, H. (2013). Determination of Iriflophenone 3-C-β-d-Glucoside from Aquilaria spp. By an indirect competitive enzyme-linked immunosorbent assay using a specific polyclonal antibody. Journal of Food Science, 78(9), 1363–1367. https://doi.org/10.1111/1750-3841.12225
- Rahman, H., Eswaraiah, M. C., & Dutta, A. M. (2016). Anti-arthritic activity of leaves and oil of Aquilaria agallocha. Haya: The Saudi Journal of Life Sciences, 1, 34–43. http://doi.org/10.36348/sjls
- Sarkate, A., Banerjee, S., Mir, J., Roy, P., & Sircar, D. (2017). Antioxidant and cytotoxic activity of bioactive phenolic metabolites isolated from the yeast-extract treated cell culture of apple. Plant Cell, Tissue and Organ Culture, 130(3), 641–649. https://doi.org/10.1007/s11240-017-1253-0
- Sharma, P., Jha, A. B., Dubey, R. S., & Pessarakli, M. (2012). Reactive oxygen species, oxidative damage, and antioxidative defense mechanism in plants under stressful conditions. Journal of Botany, 2012, 1–26. https://doi.org/10.1155/2012/217037
- Sonar, S. A., & Lal, G. (2019). The iNOS activity during an immune response controls the CNS pathology in experimental autoimmune encephalomyelitis. Frontiers in Immunology, 10, 710. https://doi.org/10.3389/fimmu.2019.00710
- Sun, Y., Bao, Y., Yu, H., Chen, Q., Lu, F., Zhai, S., Zhang, C., Li, F., Wang, C., & Yuan, C. (2020). Anti-rheumatoid arthritis effects of flavonoids from Daphne genkwa. International Immunopharmacology, 83, 106384. https://doi.org/10.1016/j.intimp.2020.106384
- Télessy, I. G. (2019). Nutraceuticals. In R. B. Singh, R. R. Watson, & T. Takahashi (Eds.), The role of functional food security in global health (pp. 409–421). Academic Press. https://doi.org/10.1016/B978-0-12-813148-0.00024-4
- Temple, N. J. (2022). A rational definition for functional foods: A perspective. Frontiers in Nutrition, 9, 957516. https://doi.org/10.3389/fnut.2022.957516
- Vijayalakshmi, U., & Shourie, A. (2019). Yeast extract-mediated elicitation of anti-cancerous compounds licoisoflavone B, licochalcone A, and liquirtigenin in callus cultures of Glycyrrhiza glabra. BioTechnologia, 100(4), 441–451. https://doi.org/10.5114/bta.2019.90245
- Wang, F., Yan, J., Niu, Y., Li, Y., Lin, H., Liu, X., Liu, J., & Li, L. (2014). Mangiferin and its aglycone, norathyriol, improve glucose metabolism by activation of AMP-activated protein kinase. Pharmaceutical Biology, 52(1), 68–73. https://doi.org/10.3109/13880209.2013.814691
- Wisutthathum, S., Kamkaew, N., Inchan, A., Chatturong, U., Paracha, T. U., Ingkaninan, K., Wongwad, E., & Chootip, K. (2019). Extract of Aquilaria crassna leaves and mangiferin are vasodilators while showing no cytotoxicity. Journal of Traditional and Complementary Medicine, 9(4), 237–242. https://doi.org/10.1016/j.jtcme.2018.09.002
- Wongwad, E., Pingyod, C., Saesong, T., Waranuch, N., Wisuitiprot, W., Sritularak, B., Temkitthawon, P., & Ingkaninan, K. (2019). Assessment of the bioactive components, antioxidant, antiglycation and anti-inflammatory properties of Aquilaria crassna Pierre ex Lecomte leaves. Industrial Crops and Products, 138, 111448. https://doi.org/10.1016/j.indcrop.2019.06.011
- Xue, Q., Yan, Y., Zhang, R., & Xiong, H. (2018). Regulation of iNOS on immune cells and its role in diseases. International Journal of Molecular Sciences, 19(12), 3805. https://doi.org/10.3390/ijms19123805
- Złotek, U., Szymanowska, U., Rybczyńska-Tkaczyk, K., & Jakubczyk, A. (2020). Effect of Jasmonic acid, yeast extract elicitation, and drying methods on the main bioactive compounds and consumer quality of lovage (Levisticum officinale Koch). Foods (basel, Switzerland), 9(3), 323. https://doi.org/10.3390/foods9030323