ABSTRACT
Objective
5.8 GHz spectrum is gaining more attention in wireless technology. To explore the potential hazards, we investigated the effect of exposure to 5.8 GHz microwave on learning and memory ability of rats. Methods: Morris Water maze (MWM), Novel object recognition (NOR) and Fear conditioning test (FCT) were used to evaluate the ability of spatial and non-spatial memory of rats. The hippocampal morphology, the level of brain injury factors in serum and the mitochondrial membrane potential of hippocampal neurons was examined to evaluate the damage of hippocampal neurons. The density of dendritic spines, the ultrastructure of synapses and the level of PSD95, Synaptophysin, p-CREB and CREB were detected to evaluate the hippocampal synaptic plasticity.
Results
Compared with Sham group, there was no significant difference in the performance of ethology (in MWM, NOR, FCT) in Microwave 2 h group or Microwave 4 h group. The hippocampal morphology, the serum level of brain injury factors and the content of mitochondrial JC-1 monomer in Microwave 2 h group or Microwave 4 h group did not change obviously, compared with Sham group. The density of dendritic spines, the ultrastructure of synapse and the level of PSD95, Synaptophysin, p-CREB and CREB in hippocampus in Microwave 2 h group or Microwave 4 h group did not significantly change, compared with Sham group.
Conclusion
Under this experimental condition, exposure to 5.8 GHz microwave could not affect the hippocampal synaptic plasticity of rats.
Introduction
Microwave is a kind of non-ionizing radiation, which is mainly generated by wireless communication devices, radar equipment and etc. Compared with traditional 2.4 GHz spectrum, the 5.8 GHz spectrum has a faster data rate, fewer disconnections and a greater application. Recently, 5.8 GHz spectrum has been authorized to be the common frequency band of point-to-point or point-to-multi-point spread spectrum communication system, high-speed wireless local area network, broadband wireless access system, Bluetooth technology system and vehicle wireless automatic identification system in China.
The rapid introduction of 5.8 GHz spectrum has increased public concern about the potential adverse effects on human health. Scientists have been aware of this issue and a few studies have been conducted in vitro. Miyakoshi(Miyakoshi et al. Citation2019) reported that exposure to 5.8 GHz microwave had little or no influence on genotoxicity in HCE-T cultured cells derived human eye. The results of Kuzniar(Kuzniar et al. Citation2017) indicated that less than 1% of the quantitated human or mouse proteome responded to 5.8 GHz microwave by small changes in protein abundance.
Brain is sensitive to microwave exposure(Hao et al. Citation2015). Exposure to specific parameters of microwave could induce changes in neurological behavior in humans or animals. Schoeni(Schoeni et al. Citation2015) found that Wi-Fi microwave exposure could affect memory performance of adolescents. Mortazavi(Mortazavi et al. Citation2013) found that exposure to radar microwave radiation lead to decreased reaction time and the lower performance of short-term memory. Some studies suggested that the spatial memory of rats was impaired after microwave exposure(Narayanan et al. Citation2009; Varghese et al. Citation2018).
At present, none of existed literature had reported the effects of 5.8 GHz microwave exposure on neurological behavior yet. Therefore, in this study, we investigated the effects of exposure to 5.8 GHz microwave on learning and memory ability of rats.
Materials and methods
Animals
Seventy-five healthy adult male Sprague–Dawley rats with body weight (258 ± 5) g were purchased from the Laboratory Animal Center of Air Force Medical University (Xi’an, China). All rats were allowed free access to food and water under controlled conditions (12/12 h light/dark cycle with humidity of 50 ± 2%, and the temperature of 23 ± 2°C). All animal experiments reported in this study were conducted according to the experimental protocol approved by the Research and Education of Air Force Medical University (No. 20,170,607, Xi’an, China), and all efforts were made to minimize animal suffering.
Exposure and groups
The exposure system mainly consisted of a signal generator, an amplifier, and a radiating antenna (). The signal generator (MG3692A, Anritsu, Japan) was used to generate the initial carrier signal with preset amplitude. The specific signal was amplified via power amplifier (SWTA-P5kC-1, Chengdu Siwi Electronic Co.,Ltd., China). A horn antenna (HD-58HA20, Hengda Microwave, China) was used for the exposure in horizontal polarization. The gain of the antenna was 20 dB and the half-power beam width was 19.9° at 5.8 GHz.
The rats were randomly divided into Sham exposure group (Sham, n = 25), Microwave exposure for 2 h (Microwave 2 h, n = 25) group and for 4 h (Microwave 4 h, n = 25) group. Both Microwave groups were exposed for 15 days. The rats were individually placed in plexiglass experimental boxes (26 cm × 8 cm × 7 cm, with small ventilation holes on every side). In each group, the 25 experimental boxes loaded with rats were arranged in 5 × 5 array and placed on the shelf with the lateral side facing the antenna. The distance from the antenna to the plane of the target animals was 3.3 m, which ensured that the target animals were in the far field of the antenna. During exposure, the rats were awake and were kept unrestrained in the plexiglass box. The Power density (PD) was 74.25 W/m2, the whole-body specific absorption rate (SAR) was 1.15 W/kg and the brain SAR was 2.33 W/kg. The PD value and SAR value increased with the distance from the center, and the maximum attenuation value is about 3 dB. The rectal temperature of rats was measured immediately before and after 5.8 GHz microwave exposure, which caused a rise in rectal temperature of <1°C. The exposure of Microwave 4 h group was conducted from 8:00 to 12:00 while Microwave 2 h group was from 8:00 to 10:00. The animals in the Sham group were treated in the same way as those in the Microwave group, except that the power system of microwave exposure was switched off. In addition, the animals of Microwave 2 h group were remained in the plexiglass experimental boxes for 2 hours bonus after exposure to control the same confinement time of the animals, which means all the animals in different groups were placed in plexiglass experimental boxes for 4 hours per day.
Morris Water Maze (MWM)
MWM was used to detect the spatial learning and memory ability of rats after microwave exposure (n = 13 for each group). The cylindrical tank used in the experiment was 150 cm in diameter and 50 cm in height. During the experiment, the water temperature was kept at (22 ± 1) °C, and the ink was poured into the water to prevent the rats from observing the bottom of the tank. The escape plateau (12 cm in diameter) was located 2 cm underwater. The training trial was performed from 10th to 14th day, while the probe trial was performed at 15th day. The experimental procedure was performed as Vorhees (Vorhees and Williams Citation2006) described and recorded by Noldus Ethovision XT (Noldus, Holland). The experiment was conducted using 13 independent animals from each group.
Novel Object Recognition (NOR)
NOR was used to detect the scene memory ability of rats. The experiment was conducted in the open field (1 m × 1 m). For the experiment, object A and object B were rectangular and six-prism black iron blocks with the same size (5 cm × 2 cm × 8 cm), respectively. The objects were placed 10 cm away from the bottom edge of the open field. Twenty-four hours before the experiment, the rats were placed in the open field for 5 min to adaptation. Then the rats were put back into the original cage. During the training phase, two identical objects A were put into the open field, and the rats were permitted to observe the objects for 5 min. After training, the rats were put back into the cage for 1 h. In the test phase, one object A was replaced by another object B, and the rats were still placed in the open field for 5 min. The experiment was performed from 11th to 12th day. The exploration of rat was recorded and analyzed by Noldus Ethovision XT (Noldus, Holland). The experiment was conducted using 12 independent animals from each group.
Fear Conditioning Test (FCT)
FCT was used to detect the fear memory of rats (n = 12 for each group) and was performed from 12th to 13th day. For a total of 5 min, the rats were given 3 periods of sound stimulation (70 dB, 2000 Hz, 30 s) and electrical stimulation (0.2 mA, 2 s) in the Skinner box after 2 min of adaptation. The next day rats were put back into the color-changed Skinner box for 5 min, but no stimulation was exported. One hour later, rats were put into the original Skinner box again to receive 3 cycles of sound stimulation only. The behavior of rat was recorded and analyzed by SuperFcs (XinRuan Technology, China). The experiment was conducted using the same animals as the NOR.
Hematein-Eosin (HE) Stain
After 15 days of 5.8 GHz microwave exposure, rats were deeply anesthetized with 1% sodium pentobarbital (60 mg/kg) and perfused with 4% paraformaldehyde (PFA) in phosphate buffer after flushing out the blood with 0.9% sodium chloride. The brain was harvested and fixed in 4% PFA for routine histological examination. After being trimmed, dehydrated, defatted and embedded, the brain was serially sliced with the thickness of 3 μm by Leica RM2135 rotary microtome (Leica, Germany). Subsequently, the brain slices were stained with hematoxylin–eosin (HE) according to routine procedure. The morphology of the brain was observed by Leica DMI4000B microscope (Leica, Germany). The experiment was conducted using four independent animals from each group.
Immunofluorescence stain
The brains were harvested as described in the HE stain and immersed in 30% sucrose at 4°C until they sank to the bottom. Serial coronal, 25-μm brain sections were prepared using a Leica CM1800 cryotome (Leica, Germany) and stored at −20°C. For staining, the sections were washed with 5-mM sodium phosphate-buffered 0.9% sodium chloride (PBS, pH 7.2–7.4) 3 times (5 minutes each), then incubated in polyclonal rabbit PSD95 (1:500, Cat.# ab18258, Abcam, UK) at 4°C overnight and rewarmed at room temperature for 30 minutes. After being washed with PBS, the sections were incubated with Alexa Fluor 594-conjugated donkey anti-rabbit IgG (1:500, Cat.# 127,803, Jackson Immuno Research Labs, USA) for 3 hours at room temperature. After being washed with PBS, the sections were incubated with DAPI staining solution (Cat.# DA0002, Leagene, China) at room temperature for 10 minutes. Finally, all the sections were washed, mounted onto glass slides and cover-slipped. Digital images were obtained with Leica DMI4000B microscope (Leica, Germany) and analyzed by Fiji (NIH, USA). The experiment was conducted using 4 independent animals from each group.
Golgi stain
After anesthesia as previously described, the rats were quickly sacrificed and the brains were harvested. The Golgi stain was performed strictly according to the manual of FD Rapid Golgi Stain Kit (Cat.# PK401, FD Neurotech, USA). The brains were sliced coronally by Leica CM1800 freezing microtome (Leica, Germany) with the thickness of 150 μm. After being shielded from light for drying, the dendritic spine in the middle of the secondary and tertiary dendrites of the pyramidal neurons in the CA1 region of the hippocampus was observed under the microscope (Olympus, Japan). The density of the dendritic spine was detected by Fiji (NIH, USA). The experiment was conducted using 4 independent animals from each group.
Transmission Electron Microscope (TEM)
After flushing out the blood with 0.9% sodium chloride, the brains were harvested and the hippocampus was isolated. Small pieces of tissue specimens are optimally placed rapidly into glutaraldehyde and fixed for at least 1 h at room temperature and then post-fixed in osmium tetroxide. Fixed cells are embedded in agar. The specimens are dehydrated in graded concentrations of ethanol and propylene oxide, and embedded in Spurr’s plastic. Semi-thin sections are cut from blocks with a glass knife and the blocks are selected for thinning. Thin sections cut with diamond knives are placed on copper grids, impregnated with uranyl acetate and lead citrate, and scoped. The experiment was conducted using 4 independent animals from each group. The size of synapses, the number of synaptic vesicles, the width of synaptic cleft and the area of postsynaptic dense was detected and measured by Fiji (NIH, USA).
Enzyme Linked Immunosorbent Assay (ELISA)
Rats were deeply anesthetized with 1% sodium pentobarbital as described after the whole process of the experiment. Blood samples were taken from the left ventricle of the heart. After sufficient clotting, centrifuge at 3000 g for 15 min at 4°C and collect serum for the assay. The content of Neuron specific enolase (NSE) and S100 calcium-binding protein B (S100B) in serum was determined with ELISA Kit (Cat.# KE1312 and Cat.# KE1454, respectively, Immunoway, USA) according to the manual. The experiment was conducted using 5 independent animals from each group.
Mitochondrial membrane potential detection
The animals were anesthetized, the hippocampus was isolated rapidly from the brain. After weighing, the tissues were homogenate and centrifuged (4°C, 600 g, 5 min) to get supernatant, centrifuged (4°C, 11,000 g, 10 min) again to collect precipitate for the purification of mitochondria, resuspended the mitochondria Mitochondrial storage fluid (Cat.# C3606, Beyotime, China). The resuspended mitochondria and JC-1 working solution (Cat:# C2006, Beyotime, China) were added into 96-well plates at volume ratio of 1:9, mixed and scanned with excitation wavelength of 485 nm and emission wavelength of 590 nm by fluorescence microplate reader. The experiment was conducted using 4 independent animals from each group.
Western Blot (WB)
The brain of rat was harvested, and the hippocampus was isolated. After being lysed with radio immunoprecipitation assay lysis buffer, which was supplemented with phenylmethanesulfonyl fluoride and phosphatase/protease inhibitors (Cat.# KGP250, KeyGEN Biotech, China), the hippocampus tissue was homogenized (4°C, 12,000 rpm, 15 min) to extract total proteins in a homogenate device (Leica, Germany). The experiment was conducted using four independent animals from each group.
The concentration of total proteins obtained above was measured by Bicinchoninic Acid Protein Assay Kit (Cat.# P0010, Beyotime, China). About 25 μg protein from each sample was separated by 10% SDS-PAGE and then transferred onto PVDF membrane (Millipore, USA). The membranes were then blocked with 5% non-fat milk for 2 h at room temperature and incubated with primary antibody overnight at 4°C (). After being rewarmed, the membranes were then incubated with species-matched HRP-conjugated secondary antibodies (1:5000, CWBIO, China) for 2 h at room temperature. The bonded proteins were visualized with chemiluminescent HRP substrate (Millipore, USA) by Universal Hood II Electrophoresis Imaging Cabinet (Bio-Rad, Italy), and quantified by Quantity One 4.62 (Bio-Rad, Italy).
Table 1. Primary antibodies used for WB.
Statistical analysis
All data were presented as Mean ± SEM. SPSS 17.0 (SPSS Inc., USA) was used for statistical analysis with one-way ANOVA by the individual blinded to group of exposure. P value <0.05 were considered statistically significant.
Results
Effects of exposure to 5.8 GHz microwave on the learning and memory ability of rats under current conditions
The results of MWM showed that (), after exposure for 15 days, there was no significant difference in the escape latency, the frequency of crossing the plateau and the percentage of time spent in the target quadrant of three groups in the training trial, as well as the latency of the first arrival to the plateau of three groups in the probe trial (P > 0.05), which indicated that exposure to microwave under current condition had no obvious effect on the spatial learning and memory ability of rats.
Figure 2. Effects of exposure to 5.8 GHz microwave on ethology. (A) Escape Latency in MWM, (B) Frequency to crossing the plateau in MWM, (C) Percentage of time spent in plateau quadrant in MWM, (D) The latency of the first arrival to the plateau in MWM, (E) Exploring time of novel object in NOR, (F) Exploring time of familiar object in NOR, (G) Percentage of time spent in exploring the novel object in NOR, (H) Discrimination index in NOR, (I) Percentage of freezing time in context test of FCT, (J) Percentage of freezing time in cued test of FCT.
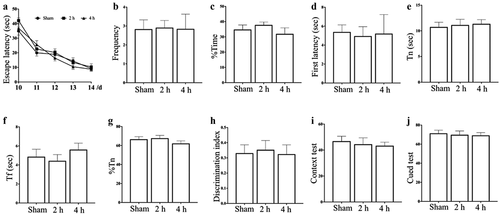
The results of NOR showed that (), after exposure for 15 days, there was no significant difference in the exploration time of novel and familiar objects, the percentage of exploring time of novel object and the discrimination index in Microwave 2 h group or Microwave 4 h group, compared with Sham group (P > 0.05), which indicated that exposure to microwave under current condition had no obvious effect on the scene memory ability of rats.
The results of FCT showed that (), compared with Sham group, Microwave 2 h group or Microwave 4 h group had no significant difference in the percentage of rigidity time after exposure for 15 days (P > 0.05), which indicated that exposure to microwave under current condition had no obvious effect on the emotional memory ability of rats.
Effects of exposure to microwave on the hippocampal neurons of rats under current condition
The results of HE stain showed that (), after exposure for 15 days, the hippocampal morphology of rats in Microwave 2 h group or Microwave 4 h group did not change obviously, compared with Sham group. NSE and S100B are usually considered as indicators for brain injury assessment and prognosis prediction. The results of ELISA showed that (), there was no significant increase of NSE and S100B in serum after exposure to 5.8 GHz Microwave 2 h/day or 4 h/day for 15 days (P > 0.05).
Figure 3. Effects of exposure to microwave on the hippocampal neurons. (A) HE stain and TEM, bar = 400 μm for Hippocampus (Hippo), bar = 100 μm for CA1, bar = 5 μm for Neuron and bar = 1 μm for Mitochondria (Mit), (B) The content of NSE in serum, (C) The content of S100B in serum, (D) The fluorescence intensity of JC-1 monomer in hippocampal neurons.
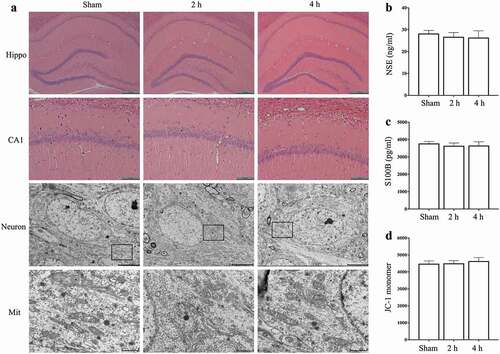
The mitochondrial membrane potential of hippocampal neurons was detected by JC-1 and the ultrastructure of mitochondria was detected by TEM. The results of JC-1 assay showed that (), after exposure for 15 days, Microwave 2 h group or Microwave 4 h group had no significant difference in the content of mitochondrial JC-1 monomer in hippocampal neurons, compared with Sham group (P > 0.05). The results of TEM showed that (), after exposure for 15 days, no obvious mitochondrial swelling was observed in each group. The mitochondrial ridges of the hippocampal neurons in each group were clear, the mitochondrial membrane was intact, which suggested that exposure to microwave under current condition had no obvious effect on hippocampal mitochondria.
Effects of exposure to microwave on the hippocampal synaptic plasticity of rats under current condition
Golgi stain showed that (), compared with Sham group, the density of apical and basal dendritic spines in CA1 region of hippocampus in Microwave 2 h group or Microwave 4 h group was not significantly changed after exposure for 15 days (P > 0.05).
Figure 4. Effects of exposure to microwave on the synapses in hippocampus. (A) Golgi stain, bar = 20 μm, (B) The density of apical dendritic spines, (C) The density of basal dendritic spines. (D) The synaptic ultrastructure, bar = 500 nm, (E) The area of postsynaptic dense, (F) The number of synaptic vesicles, (G) The size of synapses, (H) The width of synaptic cleft.
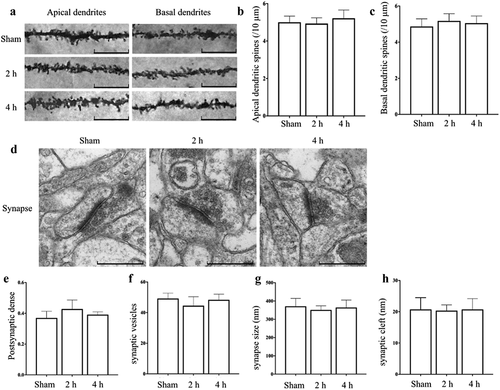
The results of TEM showed that (), after exposure for 15 days, no obvious synaptic disintegration was observed in each group. The pre- and post-synaptic membranes were clear and intact, the synaptic vesicles were uniformly sized and clustered in the presynaptic terminal. The size of synapses, the number of synaptic vesicles, the width of synaptic cleft and the area of postsynaptic dense in Microwave 2 h group or Microwave 4 h group were not significantly changed, compared with those in Sham group (P > 0.05), which suggested that exposure to microwave under current condition had no obvious effect on the synaptic ultrastructure in hippocampus of rats.
Synaptophysin and PSD95 are located in the presynaptic membrane and postsynaptic membrane, and are closely related to synaptic plasticity(Becher et al. Citation1999; El-Husseini et al. Citation2000). p-CREB and CREB are important nuclear transcription factors, not only play an important role in regulating the processes of neuronal differentiation, maturation and death, but also participate in the regulation of memory formation and synaptic plasticity(Silva et al. Citation1998; Kandel Citation2001; Dawson and Ginty Citation2002; Pittenger and Duman Citation2008; Mamiya et al. Citation2009; Luo et al. Citation2017). The results of WB showed that (), after exposure for 15 days, the protein level of PSD95, Synaptophysin, p-CREB and CREB in hippocampus did not significantly change in Microwave 2 h group or Microwave 4 h group, compared with Sham group (P > 0.05).
Figure 5. Effects of exposure to microwave on synapse related protein in hippocampus. (A) The results of WB, (B-E) The semi-quantitative of p-CREB, CREB, PSD95 and Synaptophysin, respectively.
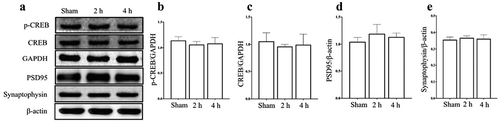
The results of immunofluorescence stain showed that (), after exposure for 15 days, compared with Sham group, there was no significant difference in the number of PSD95+ cells in CA1 region in Microwave 2 h group or Microwave 4 h group (P > 0.05), and so did the fluorescence intensity of PSD95 in CA1 region (P > 0.05).
Figure 6. Effects of exposure to microwave on the localization of PSD95 in CA1 region. (A) Immunofluorescence stain, bar = 200 μm and bar = 100 μm, (B) The number of PSD95+ cells, (C) The fluorescence intensity of PSD95.
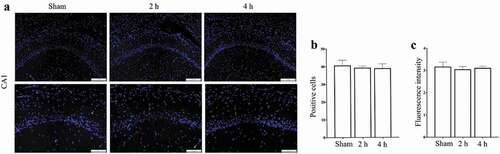
The above results indicated that exposure to 5.8 GHz microwave (2 h/day or 4 h/day, 15 days) had no obvious effect on the hippocampal synaptic plasticity of rats.
Discussion
According to the standard released by International Committee on Electromagnetic Safety (IEEE) in 2005, the power density limit of occupational exposure at 5.8 GHz spectrum was 100 W/m2(CitationIEEE). While, in the latest C95.1TM-2019 edition of ‘IEEE Standard for Safety Levels with Respect to Human Exposure to Radio Frequency Electromagnetic Fields’, the limit was adjusted to 50 W/m2(CitationIEEE). Considered of the evolution of the two above IEEE standard, the power density of microwave in this study was selected as 75 W/m2, the actual measured value was 74.25 W/m2. Under this condition, the whole-body SAR of rats was 1.15 W/kg and was lower than the threshold of thermal effect (4 W/kg), which was consistent with the results of the rectal temperature detecting in our study. It was reported that cognitive decline which is closely connected to the hippocampal synaptic plasticity could be induced immediately even after a single 6-min exposure to microwave (SAR < 4 W/kg) and could last up to 28 days(Tan et al. Citation2017). In this study, considered with the reported literature and the reality, the exposure time was taken as 2 h/day and 4 h/day for 15 days, the total exposure time was 30 h and 60 h, respectively.
It was reported that impairments of pyramidal and granular cell were observed after 25 h exposure with 900 MHz microwave. Kim(Kim et al. Citation2018) confirmed that pan-calcium channel expression in hippocampal neurons was significantly decreased after exposure to 835 MHz microwave, moreover, the autophagy pathway was activated while apoptosis pathway was inhibited. It was reported that after exposure to microwave 1 h/day for 4 weeks, nucleus shrinkage, apoptosis and necrosis were observed in the hippocampal neurons of rats(Bas et al. Citation2009; Narayanan et al. Citation2010). Mitochondrion not only provides energy for cell, but also plays a crucial role in the regulation of long-term memory at the level of synapses(Naziroglu et al. Citation2013; Carrasco et al. Citation2015; Fu et al. Citation2017; Khacho et al. Citation2019). Aitken(Aitken et al. Citation2005) reported that microwave had significant damage to the mitochondrial genome of mice. The study of Ertilav(Ertilav et al. Citation2018) found that microwave exposure of 900 MHz and 1.8 GHz could induce the depolarization of mitochondrial membrane, the production of reactive oxygen species and the activation of hippocampal neurons. At the same time, some studies also reported that microwave exposure could not trigger significant biological damage effects (Son et al. Citation2016; Shirai et al. Citation2017; Jeong et al. Citation2018). In our study, there were no hippocampal neuron damage after exposure to 5.8 GHz microwave 2 h/day for 15 days, and it is consistent with those negative finding studies. The contradictory results could be due to the strain, the age and the gender of animals, as well as the parameters of microwave used in the study.
It is generally accepted that synaptic plasticity encodes memories. Some studies reported that microwave exposure could significantly inhibit hippocampal synaptic plasticity. In vitro, exposure to 1.8 GHz microwave could decrease the number and the activity of excitatory synapses in hippocampal neurons(Xu et al. Citation2006). In vivo, exposure to microwave could not only cause structural and functional damage to the hippocampus, but also reduce the density of dendritic spines, inhibit the NMDAR-PSD95-CaMKII signal pathway, and alter the expression of Synapsin I, Vamp-2, Syntaxin and Synaptophysin(Wang et al. Citation2009, Citation2015; Zhi et al. Citation2018; Sharaf et al. Citation2019). However, scientists found that even high power microwave did not affect the ability of neurons to develop or retain the long-term potentiation(Pakhomov et al. Citation2003). At the molecular level, changes in synaptic structure are often accompanied by changes in the expression of transcription factor. It is verified that CREB plays a vital role in the regulation of transportation of synaptic receptors and the expression of PSD95(Middei et al. Citation2013). Studies have shown that exposure to 2.45 GHz microwave for 15 days could significantly reduce the level of CREB and inhibit synaptic plasticity in hippocampus(Shahin et al. Citation2018). Researchers studied the effect of 2.856 GHz microwave exposure on synaptic plasticity and found that, p-CREB notably increased in the PC12 cells after exposure to 2.856 GHz microwave, however, p-CREB significantly decreased in the exposed hippocampal tissues(Xiong et al. Citation2015). This different p-CREB expression pattern might result from the activation of diverse signal pathways, which included protein kinase A, mitogen activated protein kinases, and other Ca2+/calmodulin kinases, associated with microwave exposure in the hippocampus. The study of Gokceksarac(Gokcek-Sarac et al. Citation2017) showed that, the expression of CREB in hippocampus of rats was continuously up-regulated after exposure to 2.1 GHz microwave, while the expression of CERB in hippocampus was fluctuated after exposure to 900 MHz microwave. In our study, exposure to microwave had no influence on the density of dendritic spines or on the level of p-CREB, CREB, PSD95 and Synaptophysin in hippocampus, which indicated that exposure to 5.8 GHz microwave had no obvious effect on the hippocampal synaptic plasticity of rats.
According to the World Health Organization, the influence of electromagnetic radiation on human health is mainly caused by the thermal effect, but the intensity of daily microwave exposure is relatively low, which usually cannot produce obvious thermal effect (Van Deventer et al. Citation2011). Although some studies have found some positive results, these findings are difficult to replicate because of the nonthermal effects and uncertainty associated with low-intensity exposure. Moreover, different laboratories have come up with radically different results. If these differences are not due to systematic or measurement errors, then the results are to some extent related to differences in experimental equipment or inconsistencies in exposure parameters, and differences in genetic background and health status may also result in inconsistencies in experimental results. Therefore, joint multi-center studies are recommended in the future.
Conclusions
From the results described above, we conclude that under this experimental condition, exposure to 5.8 GHz microwave (2 h/day or 4 h/day, 15 days) could not affect the learning and memory ability of rats. Whether positive findings with prolonged exposure time or enhanced exposure intensity could be induced remains to be further investigated.
Acknowledgments
The authors thank Jia-Jin Lin for technical support.
Disclosure statement
No potential conflict of interest was reported by the authors.
References
- Aitken RJ, Bennetts LE, Sawyer D, Wiklendt AM, King BV. 2005. Impact of radio frequency electromagnetic radiation on DNA integrity in the male germline. Int J Androl. 28(3):171–179. doi:10.1111/j.1365-2605.2005.00531.x.
- Bas O, Odaci E, Kaplan S, Acer N, Ucok K, Colakoglu S. 2009. 900 MHz electromagnetic field exposure affects qualitative and quantitative features of hippocampal pyramidal cells in the adult female rat. Brain Res. 1265:178–185. doi:10.1016/j.brainres.2009.02.011.
- Becher A, Drenckhahn A, Pahner I, Margittai M, Jahn R, Ahnert-Hilger G. 1999. The synaptophysin-synaptobrevin complex: a hallmark of synaptic vesicle maturation. J Neurosci. 19(6):1922–1931. doi:10.1523/JNEUROSCI.19-06-01922.1999.
- Carrasco C, RodrÍGuez AB, Pariente JA. 2015. Melatonin as a stabilizer of mitochondrial function: role in diseases and aging. Turk J Biol. 39(6):822–831. doi:10.3906/biy-1504-26.
- Dawson TM, Ginty DD. 2002. CREB family transcription factors inhibit neuronal suicide. Nat Med. 8(5):450–451. doi:10.1038/nm0502-450.
- El-Husseini AE, Schnell E, Chetkovich DM, Nicoll RA, Bredt DS. 2000. PSD-95 involvement in maturation of excitatory synapses. Science. 290(5495):1364–1368.
- Ertilav K, Uslusoy F, Ataizi S, Naziroglu M. 2018. Long term exposure to cell phone frequencies (900 and 1800 MHz) induces apoptosis, mitochondrial oxidative stress and TRPV1 channel activation in the hippocampus and dorsal root ganglion of rats. Metab Brain Dis. 33(3):753–763. doi:10.1007/s11011-017-0180-4.
- Fu ZX, Tan X, Fang H, Lau PM, Wang X, Cheng H, Bi GQ. 2017. Dendritic mitoflash as a putative signal for stabilizing long-term synaptic plasticity. Nat Commun. 8(1):31. doi:10.1038/s41467-017-00043-3.
- Gokcek-Sarac C, Er H, Kencebay Manas C, Kantar Gok D, Ozen S, Derin N. 2017. Effects of acute and chronic exposure to both 900 MHz and 2100 MHz electromagnetic radiation on glutamate receptor signaling pathway. Int J Radiat Biol. 93(9):980–989.
- Hao YH, Zhao L, Peng RY. 2015. Effects of microwave radiation on brain energy metabolism and related mechanisms. Mil Med Res. 2:4.
- IEEE. Std C95.1TM-. 2005. IEEE Standard for Safety Levels with Respect to Human Exposure to Radio Frequency Electromagnetic Fields, 3 kHz to 300 GHz.
- IEEE. Std C95.1TM-. 2019. IEEE Standard for Safety Levels with Respect to Human Exposure to Electric, Magnetic, and Electromagnetic Fields, 0 Hz to 300 GHz.
- Jeong YJ, Son Y, Han NK, Choi HD, Pack JK, Kim N, Lee YS, Lee HJ. 2018. Impact of long-term RF-EMF on oxidative stress and neuroinflammation in aging brains of C57BL/6 mice. Int J Mol Sci. 19(7):2103. doi:10.3390/ijms19072103.
- Kandel ER. 2001. The molecular biology of memory storage: a dialogue between genes and synapses. Science. 294(5544):1030–1038. doi:10.1126/science.1067020.
- Khacho M, Harris R, Slack RS. 2019. Mitochondria as central regulators of neural stem cell fate and cognitive function. Nat Rev Neurosci. 20(1):34–48.
- Kim JH, Sohn UD, Kim HG, Kim HR. 2018. Exposure to 835 MHz RF-EMF decreases the expression of calcium channels, inhibits apoptosis, but induces autophagy in the mouse hippocampus. Korean J Physiol Pharmacol. 22(3):277–289. doi:10.4196/kjpp.2018.22.3.277.
- Kuzniar A, Laffeber C, Eppink B, Bezstarosti K, Dekkers D, Woelders H, Zwamborn AP, Demmers J, Lebbink JH, Kanaar R. 2017. Semi-quantitative proteomics of mammalian cells upon short-term exposure to non-ionizing electromagnetic fields. PLoS One. 12(2):e0170762. doi:10.1371/journal.pone.0170762.
- Luo Y, Kuang S, Li H, Ran D, Yang J. 2017. cAMP/PKA-CREB-BDNF signaling pathway in hippocampus mediates cyclooxygenase 2-induced learning/memory deficits of rats subjected to chronic unpredictable mild stress. Oncotarget. 8(22):35558–35572. doi:10.18632/oncotarget.16009.
- Mamiya N, Fukushima H, Suzuki A, Matsuyama Z, Homma S, Frankland PW, Kida S. 2009. Brain region-specific gene expression activation required for reconsolidation and extinction of contextual fear memory. J Neurosci. 29(2):402–413. doi:10.1523/JNEUROSCI.4639-08.2009.
- Middei S, Houeland G, Cavallucci V, Ammassari-Teule M, D’Amelio M, Marie H. 2013. CREB is necessary for synaptic maintenance and learning-induced changes of the ampa receptor GluA1 subunit. Hippocampus. 23(6):488–499. doi:10.1002/hipo.22108.
- Miyakoshi J, Tonomura H, Koyama S, Narita E, Shinohara N. 2019. Effects of exposure to 5.8 GHz electromagnetic field on micronucleus formation, DNA strand breaks, and heat shock protein expressions in cells derived from human eye. IEEE Trans Nanobiosci. 18(2):257–260. doi:10.1109/TNB.2019.2905491.
- Mortazavi SM, Taeb S, Dehghan N. 2013. Alterations of visual reaction time and short term memory in military radar personnel. Iran J Public Health. 42(4):428–435.
- Narayanan SN, Kumar RS, Potu BK, Nayak S, Bhat GP, Mailankot M. 2010. Effect of radio-frequency electromagnetic radiations (RF-EMR) on passive avoidance behaviour and hippocampal morphology in Wistar rats. Ups J Med Sci. 115(2):91–96. doi:10.3109/03009730903552661.
- Narayanan SN, Kumar RS, Potu BK, Nayak S, Mailankot M. 2009. Spatial memory performance of Wistar rats exposed to mobile phone. Clinics (Sao Paulo). 64(3):231–234. doi:10.1590/S1807-59322009000300014.
- Naziroglu M, Yuksel M, Kose SA, Ozkaya MO. 2013. Recent reports of Wi-Fi and mobile phone-induced radiation on oxidative stress and reproductive signaling pathways in females and males. J Membr Biol. 246(12):869–875. doi:10.1007/s00232-013-9597-9.
- Pakhomov AG, Doyle J, Stuck BE, Murphy MR. 2003. Effects of high power microwave pulses on synaptic transmission and long term potentiation in hippocampus. Bioelectromagnetics. 24(3):174–181. doi:10.1002/bem.10079.
- Pittenger C, Duman RS. 2008. Stress, depression, and neuroplasticity: a convergence of mechanisms. Neuropsychopharmacol. 33(1):88–109.
- Schoeni A, Roser K, Roosli M. 2015. Memory performance, wireless communication and exposure to radiofrequency electromagnetic fields: a prospective cohort study in adolescents. Environ Int. 85:343–351. doi:10.1016/j.envint.2015.09.025.
- Shahin S, Banerjee S, Swarup V, Singh SP, Chaturvedi CM. 2018. From the cover: 2.45-GHz Microwave radiation impairs hippocampal learning and spatial memory: involvement of local stress mechanism-induced suppression of iGluR/ERK/CREB signaling. Toxicol Sci. 161(2):349–374. doi:10.1093/toxsci/kfx221.
- Sharaf NE, Galal AF, El-Sawy MS, Shalby AB, Sayed AH, Ahmed HH. 2019. Role of designed Bio-Geometrical forms in antagonizing neurobehavioral burden of Wi-Fi radiation: evidence-based experimental study. Biomed Pharmacol J. 12(3):1217–1234. doi:10.13005/bpj/1751.
- Shirai T, Wang J, Kawabe M, Wake K, Watanabe SI, Takahashi S, Fujiwara O. 2017. No adverse effects detected for simultaneous whole-body exposure to multiple-frequency radiofrequency electromagnetic fields for rats in the intrauterine and pre- and post-weaning periods. J Radiat Res. 58(1):48–58. doi:10.1093/jrr/rrw085.
- Silva AJ, Kogan JH, Frankland PW, Kida S. 1998. CREB and Memory. Annu Rev Neurosci. 21(1):127–148. doi:10.1146/annurev.neuro.21.1.127.
- Son Y, Jeong YJ, Kwon JH, Choi HD, Pack JK, Kim N, Lee YS, Lee HJ. 2016. 1950 MHz radiofrequency electromagnetic fields do not aggravate memory deficits in 5xFAD mice. Bioelectromagnetics. 37(6):391–399. doi:10.1002/bem.21992.
- Tan S, Wang H, Xu X, Zhao L, Zhang J, Dong J, Yao B, Wang H, Zhou H, Gao Y, et al. 2017. Study on dose-dependent, frequency-dependent, and accumulative effects of 1.5 GHz and 2.856 GHz microwave on cognitive functions in Wistar rats. Sci Rep. 7(1):10781. doi:10.1038/s41598-017-11420-9
- Van Deventer E, van Rongen E, Saunders R. 2011. WHO research agenda for radiofrequency fields. Bioelectromagnetics. 32(5):417–421. doi:10.1002/bem.20660.
- Varghese R, Majumdar A, Kumar G, Shukla A. 2018. Rats exposed to 2.45GHz of non-ionizing radiation exhibit behavioral changes with increased brain expression of apoptotic caspase 3. Pathophysiology. 25(1):19–30. doi:10.1016/j.pathophys.2017.11.001.
- Vorhees CV, Williams MTJNP. 2006. Morris water maze: procedures for assessing spatial and related forms of learning and memory. Nat Protoc. 1(2):848–858. doi:10.1038/nprot.2006.116.
- Wang L, Peng R, Hu X, Gao Y, Wang S, Zhao L, Dong J, Su Z, Xu X, Gao R, et al. 2009. Abnormality of synaptic vesicular associated proteins in cerebral cortex and hippocampus after microwave exposure. Synapse. 63(11):1010–1016. doi:10.1002/syn.20684
- Wang L-F, Wei L, Qiao S-M, Gao X-N, Gao Y-B, Wang S-M, Zhao L, Dong J, Xu X-P, Zhou H-M, et al. 2015. Microwave-induced structural and functional injury of hippocampal and PC12 cells is accompanied by abnormal changes in the NMDAR-PSD95-CaMKII pathway. Pathobiology. 82(5):181–194. doi:10.1159/000398803
- Xiong L, Sun CF, Zhang J, Gao YB, Wang LF, Zuo HY, Wang SM, Zhou HM, Xu XP, Dong J, et al. 2015. Microwave exposure impairs synaptic plasticity in the rat hippocampus and PC12 cells through over-activation of the NMDA receptor signaling pathway. Biomed Environ Sci. 28(1):13–24.
- Xu S, Ning W, Xu Z, Zhou S, Chiang H, Luo J. 2006. Chronic exposure to GSM 1800-MHz microwaves reduces excitatory synaptic activity in cultured hippocampal neurons. Neurosci Lett. 398(3):253–257. doi:10.1016/j.neulet.2006.01.004.
- Zhi W-J, Peng R-Y, Li H-J, Zou Y, Yao B-W, Wang C-Z, Liu Z-H, Gao X-H, Xu X-P, Dong J, et al. 2018. Microwave radiation leading to shrinkage of dendritic spines in hippocampal neurons mediated by SNK-SPAR pathway. Brain Res. 1679:134–143. doi:10.1016/j.brainres.2017.11.020.