Abstract
Purpose
To evaluate the immediate and 4-week effects of compression garments (CG) on balance using a force platform during 8 different visual, static, and dynamic conditions in hypermobile Ehlers-Danlos Syndrome (hEDS) patients.
Methods
Thirty-six participants were randomly assigned to a group: physiotherapy alone (PT, n = 19) or physiotherapy and daily CG wearing for 4 weeks (PT + CG, n = 17). Both attended 12 physiotherapy sessions (strengthening, proprioception, and balance exercises) for 4 weeks. Primary outcome: sway velocity of the centre of pressure (COP) measured before, immediately with the CG, and at 4 weeks. Secondary outcomes: ellipse area, Romberg quotient, and pain.
Results
Sway velocity in dynamic conditions decreased immediately with the CG. After 4 weeks of intervention, sway velocity (95% CI 4.36–39.23, effect size 0.93) and area (95% CI 146–3274, effect size 0.45) on the laterally oscillating platform with eyes-closed improved more in the PT + CG group than the PT group. Romberg quotient on foam cushion improved more in the PT + CG than the PT group. Pain decreased in both groups after 4 weeks with no between-group difference.
Conclusion
CG combined with physiotherapy improved dynamic balance measured with COP variables significantly more than physiotherapy alone in people with hEDS.
Trial Registration
NCT03359135
IMPLICATIONS FOR REHABILITATION
Compression garments immediately improve balance in people with hypermobile Ehlers-Danlos Syndrome (hEDS)
Compression garments combined with regular physiotherapy improve balance in people with hEDS after 4 weeks of treatment
Compression garments could compensate for proprioceptive impairment in hEDS
Introduction
The Ehlers-Danlos Syndromes (EDS) are a heterogeneous group of inherited connective tissue disorders caused by mutations in genes encoding for proteins involved in the biosynthesis and/or fibrillogenesis of collagens [Citation1] Hypermobile EDS (hEDS), the most common type, is characterised by generalised joint hypermobility, systemic connective tissue disorders, a positive family history, and musculoskeletal problems including recurrent joint dislocations and chronic pain. The diagnosis of hEDS requires the exclusion of the other EDS types and other conditions with generalised joint hypermobility [Citation2–4]. The genetic basis of hEDS still unknown, therefore the diagnosis remains clinical, based on a checklist of clinical diagnostic criteria. The disorder mainly affects women [Citation2]. People with symptomatic joint hypermobility who do not meet the criteria for hEDS are diagnosed with Hypermobility Spectrum disorders (HSD). These conditions were historically known as Joint hypermobility Syndrome (JHS). Hypermobile EDS and HSD/JHS share several symptoms (such as hyperlaxity) and were defined together in the 2017 nosology [Citation1,Citation3]. Pain associated with hEDS may be nociceptive or neuropathic, or more frequently both [Citation4]. Nociceptive pain is directly caused by joint instability, which leads to repetitive joint dislocations and sprains [Citation4]. Balance and gait disorders [Citation5,Citation6], reduced proprioception [Citation7–12], muscle weakness [Citation11,Citation13], and fatigue [Citation14] are commonly associated symptoms of hEDS.
Balance is impaired in quiet standing [Citation6,Citation15,Citation16], walking, and stair climbing [Citation17] in people with hEDS, which increases the risk of falls, and causes clumsiness and kinesiophobia [Citation6]. Furthermore, affected individuals often have low levels of physical activity and participation in daily life activities [Citation18].
Medial-lateral (ML) and anterior-posterior (AP) excursions of the centre of pressure (COP) on firm surfaces are increased in people with hEDS, both with the eyes open (EO) and closed (EC) [Citation15,Citation16]. A study of balance in 22 people with hEDS in 4 sensory conditions (EO on a firm surface, EC on a firm surface, EO on a foam cushion, and EC on a foam cushion) found a significant increase in ML and AP sway with EC on a firm surface and EO on a foam cushion compared to healthy subjects [Citation6]. These results suggest that vision and proprioception contribute to maintaining a stable standing posture in people with hEDS. Although the conventional neurological examination of proprioception often finds little impairment of proprioception in people with hEDS, several studies have found altered proprioception, including in the lower limb joints [Citation7,Citation8].
The conventional management of hEDS involves regular physiotherapy with proprioception and balance exercises and muscle strengthening. The aim is to improve motor activities, decrease pain and prevent the deconditioning that results from a lack of physical activity and kinesophobia [Citation12,Citation19–31]. This rehabilitation is recommended by experts [Citation19]. However, physiotherapy is limited by factors such as access to a physiotherapist, lack of dedicated physiotherapist time with the individual, fatigue and pain [Citation32]. To our knowledge, the effects of physiotherapy programs on balance have not yet been evaluated in people with hEDS. An uncontrolled study of 18 individuals with hyperlaxity showed that proprioception and balance measured on a balance board improved after 8 weeks of closed kinetic chain exercises [Citation9].
One method to compensate for the proprioceptive impairments of hEDS is compression garments (CG). Full-body or partial CG are considered to have a mechanical effect, stimulating exteroceptive receptors and increasing the sensory feedback to the central nervous system; this could compensate for proprioceptive impairments and improve postural control [Citation8,Citation16,Citation33–35]. A meta-analysis of healthy subjects showed that in healthy participants, CG increased sensory afferent information and improved postural stability [Citation34]. In hEDS, CG could increase balance and joint stability, and decrease pain. Self-reports of the use of CG by people with hEDS indicate a positive effect on performance of daily-life activities [Citation26]. However, the objective effect has been little studied, especially on balance [Citation16,Citation36]. Shoulder stability, and external and internal shoulder rotator power increased after 4 weeks of daily wearing of a compression jacket in people with hEDS [Citation36]. A pilot study of 6 people with hEDS found an immediate improvement in balance when wearing CG (leggings, vest and mittens) on a static force platform, particularly with EC [Citation16]. The results of that small, uncontrolled, interventional study support conducting a randomized controlled study to assess the effects of CG on balance in hEDS. Moreover, dynamic conditions, involving a higher risk of falls, should be evaluated [Citation6,Citation37].
The aim of this randomized controlled trial was to evaluate the immediate and 4-week effects of wearing CG combined with conventional physiotherapy on balance and pain in people with hEDS. We hypothesised that wearing CG combined with physiotherapy would be more effective than physiotherapy alone. We also hypothesised that wearing CG would reduce pain at 4 weeks.
Materials and methods
Design
We conducted a randomized controlled trial with 2 parallel groups between 2019 and 2021. The study was approved by the research ethics committee (No. ID RCB: 2018-A000419-46 and CPP No.: 2018-22–CPP IDF 3) and registered at Clinicaltrials.gov (No. NCT03359135).
Participants
The participants were recruited from the centre of reference for non-vascular Ehlers-Danlos Syndromes at Raymond Poincaré Hospital, AP-HP Garches, where they were diagnosed with hEDS. Those with hypermobile EDS were informed about the study when they attended the centre for their usual follow-up consultations. The inclusion criteria were people with hEDS fulfilling the 2017 diagnostic criteria [Citation1,Citation2] and aged ≥ 16 years. The exclusion criteria were previous surgery or musculoskeletal disorders of the lower limbs, wearing of a CG in the previous 2 months, having followed a rehabilitation program ≥ 3 times/weeks during the last 2 months, uncontrolled hyperalgesia, visual, vestibular, or nervous system disorders, and pregnancy or breastfeeding. Individuals meeting the inclusion criteria were enrolled until the sample size was reached and written informed consent was obtained from all participants or their guardians. Data were anonymised.
Randomization and allocation
A randomization list was produced by a statistician using R software version 4.2.0. Randomization was performed with random permuted blocks of variable size with a 1:1 ratio. The list was used to prepare randomization cards, which were placed in opaque sealed envelopes. Before the opening of the envelopes, neither the researchers nor the medical staff enrolling participants were aware of the potential allocation of the future participant. Randomization took place at the inclusion visit, during which the envelope was opened in the presence of the participant.
Timeline
Enrolment and randomization were performed at the inclusion visit, during which demographic and clinical data were collected. Participants were randomly assigned to either undergo physiotherapy only for 4 weeks (PT) or to undergo physiotherapy and wear a CG for 4 weeks (PT + CG). Postural balance was assessed before starting the interventions (W0), and at 4 weeks (at the end of the interventions, W4). For the PT group, W0 was during the inclusion visit. For the PT + CG group, W0 was 2 weeks after the inclusion visit, when they received their CG. At W0, this group performed the balance assessments without then with the CG. At W4, this group only performed the assessments with the CG. Between W0 and W4, both groups attended physiotherapy sessions and the PT + CG group also wore the CG all day, every day ().
Figure 1. Timeline of the study. PT: Physiotherapy; PT + CG: Physiotherapy and Compression Garments; W0: week 0; W4: week 4.
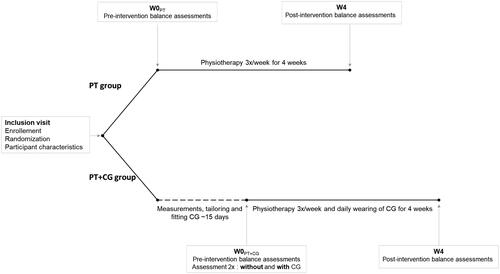
Interventions
Compression garments (for PT + CG group)
The CG were custom-made for each participant. Measurements were taken by the same orthotic practitioner at the reference centre and all garments were fabricated by the same manufacturer. The average pressures ranged from 10 to 15 mmHg. Two sets of 3 pieces (leggings, socks, and vest) were supplied. Maintenance advice and instructions were provided with the garments. After fabrication, the CG were fitted in person. If the garment did not fit, adjustments were made as required. CG wearing began at W0. Participants in the PT + CG group were instructed to wear the garments ≥ 8 h a day for 4 weeks, during daily life activities and physiotherapy. Participants were informed of possible risks associated with CG such as allergy or intolerance to materials [Citation38]. Adherence was monitored using self-report: participants recorded the actual duration of daily CG wearing in a logbook.
Physiotherapy sessions (all participants)
All participants underwent a 4-week rehabilitation program that aimed to improve proprioception and balance. The program included 1 h of outpatient physiotherapy, 3 times per week, for 4 weeks, structured around 3 domains of rehabilitation: (1) strengthening, (2) postural control, and (3) balance training. The goal was to strengthen the lower limbs to improve posture and balance. Exercises involved isometric strengthening of the ankle, knee, and hip muscles; aerobic exercises; proprioceptive training; and balance exercises on stable and unstable surfaces with EO and EC. The intervention was provided by a physiotherapist in the community near the participant’s home. These physiotherapists were supported by telephone by the reference centre physiotherapist. The performance characteristics of each exercise and the time spent per exercise were determined by the physiotherapist according to their professional clinical reasoning. Exercises were performed according to the participant’s progress, fatigue, and motivation. Adherence was monitored by self-report: participants recorded the actual duration of each session in a logbook.
Procedures
Participant characteristics
We collected demographic data (sex, age, weight, and height), joint hypermobility assessed using the Beighton and Bulbena scores [Citation39], history of repetitive ankle sprains, joint dislocations of the lower extremities, falls or balance recovery reactions, perception of instability, difficulty walking (limited distance because of joint instability or pain), joint pain, global pain and fatigue. All these data were collected by the same senior physician.
Postural sway
Body sway can be assessed by measuring centre of pressure (COP) displacement on a supporting surface using a force platform. Force platforms can also be used to collect other postural sway variables such as sway velocity and ellipse area [Citation40]. In this study, balance was assessed using the Satel® (40 Hz) force platform. Participants stood still, barefoot on the platform, arms by their sides, feet forming an angle of 30°, heels separated by 2 cm and eyes focused on a visual reference mark fixed 170 cm in front. The standardised instructions were to stay still without speaking [Citation41]. Assessments were performed in a closed and quiet room. The physical therapist from the centre performed all tests.
Eight conditions were assessed on the force platform:
Eyes open on a static firm platform (SEO)
Eyes closed on a static firm platform (SEC)
Eyes open on a foam surface (FEO)
Eyes closed on a foam surface (FEC)
Eyes open on an unstable platform that performed anterior-posterior oscillations (APEO)
Eyes-closed on an unstable platform that performed anterior-posterior oscillations (APEC)
Eyes-open on an unstable platform that performed medial-lateral oscillations (LEO)
Eyes-closed on an unstable platform that performed medial-lateral oscillations (LEC)
Three consecutive measurements were performed for conditions 1, 2, 3, and 4 and 1 for conditions 5, 6, 7, and 8 [Citation42]. The eyes closed conditions (EC) were recorded to measure the impact of vision on balance with the CG. The recording duration was 51.2 s for eyes-open (EO) measurements and 25.6 s for EC [Citation43]. The resting time between each evaluation was 30 s.
Pain
Mean joint pain intensity was assessed using a numeric pain rating scale (NPRS, between 0 = no pain and 10 = intolerable pain) at W0 and W4 before the postural sway assessments.
Outcomes: COP-based balance variables and pain
Outcomes were measured pre-intervention (W0) and immediately post-intervention (W4) (). The primary outcome was the change in mean COP velocity (in mm/s) from W0 to W4. This is the most common and reliable variable reported [Citation40,Citation42,Citation43]. A decrease in mean velocity after the intervention suggested an improvement in postural balance.
Secondary outcomes:
90% confidence ellipse area of COP (mm2)
Romberg quotient (RQ = sway area EC/sway area EO). The RQ indicates the contribution of vision to postural control. High values indicate compensation by a vision to maintain balance.
Global joint pain, expressed on the NPRS.
Statistical analysis
Although sway velocity is the most used variable in the literature and the most reliable stabilometric variable [Citation40], it has not yet been studied in hEDS.
Participant characteristics are described by the group. The summary statistics for quantitative variables are mean and standard deviation (SD), or median and quartiles (Q1,Q3) as appropriate. For categorical variables, the summary statistics are numbers with percentages. Statistical analyses of patient characteristics were performed using the Mann Whitney U, Chi-squared, and Fisher tests for between-group comparisons. Intra-group and inter-group comparisons of outcomes were performed using median differences and 95% confidence intervals. Considering the small number of participants, effect sizes were estimated using Hedges’ g. According to Cohen’s definition [Citation44], an effect size of 0.2 is considered as small, an effect size of 0.5 is considered as medium and an effect size of 0.8 is considered as large. Outcome analysis was performed as intention-to-treat. Missing data were imputed with the Last Observation Carried Forward or the Next Observation Carried Back methods. All statistical calculations were performed using R software version 4.2.0 (https://cran.r-project.org/).
Results
Sample
Thirty-six individuals met the inclusion criteria and were randomized between February 2019 and January 2021. Seventeen individuals were randomly assigned to the PT + CG group and 19 to the PT group. Two people subsequently declined to participate for professional reasons, and 2 dropped out before the first evaluation on the force platform because of an acute exacerbation of the disease that contraindicated rehabilitation (). Thus, data for 32 participants (88.9%) were available for the primary analyses (29 females and 3 males). The demographic and clinical characteristics of the 32 participants at baseline are presented in ; there were no between-group differences. Baseline sway velocities and areas did not differ between groups.
Figure 2. Flow chart. PT + CG: Physiotherapy and Compression Garments; PT: Physiotherapy; LOCF: Last Observation Carried Forward; NOCB: Next Observation Carried Back
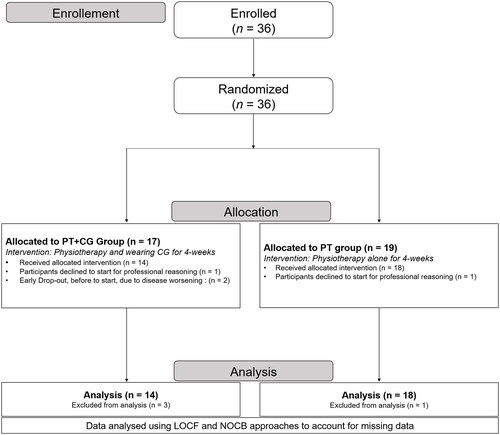
Table 1. Clinical and demographic characteristics of participants in the physiotherapy alone group (PT) and the physiotherapy and compression garments (PT + CG) group.
Adherence
Both the CG and the physiotherapy sessions were well tolerated, with no discomfort, allergy or other adverse event reported. Participants in the PT + CG group attended fewer physiotherapy sessions than the PT group: mean (SD) respectively 10.31 (1.91) and 11.55 (1.3); p = 0.003. For the PT + CG group, mean CG wearing time was 10.40 (1.82) h/d for 26 (4.5) d.
Immediate effects of the CG
presents the immediate effects of the CG on postural sway variables. These effects were only analysed in the PT + CG group. Mean COP velocity decreased with the CG in all conditions, apart from SEO; effect sizes were between 0.51 and 0.64. The sway area decreased with the CG for APEO, APEC, and FEC; effect sizes were between 0.36 and 0.43.
Table 2. Immediate effect of the compression garments in static and dynamic conditions with eyes open and eyes closed.
Effects at 4 weeks
Primary Outcome
presents the effects at 4 weeks for both groups. After 4 weeks of intervention, sway velocity decreased in the PT + CG group for 4 conditions (Δ1): median difference (Q1;Q3) APEO: 5.66 (1.27;24.29), FEC: 5.42 (0.31;30.88), APEC:13.02 (0.76;45.08), and LEC: 16.22 (3.84;42.26); effect sizes between 0.46 and 0.93.
Table 3. Outcomes at 4 weeks in both groups in the static and dynamic conditions with eyes open and eyes closed.
No statistically significant improvement was found in the PT group after the 4 weeks of intervention (Δ2).
Sway velocity decreased more in the PT + CG group than the PT group for LEC Δ3 (intergroup comparison [Δ1 − Δ2]): 18.84 (4.36;39.23); the effect size was large, at 0.93.
Secondary Outcomes
At 4 weeks, the sway area decreased in the PT + CG group (Δ1) in APEO, LEO, SEC, FEC, APEC, and LEC; effects sizes 0.09 to 0.48. The Romberg quotient decreased in the foam and medial-lateral plate conditions; effect sizes respectively 0.98 and 0.21. No differences were found for the PT group (Δ2) for sway area or Romberg quotient.
Sway area decreased more in the PT + CG group than the PT group for LEC Δ3: 1367 (146;3274); effect size 0.45. The Romberg quotient decreased more in the PT + CG group than the PT group for foam condition Δ3: 0.73 (0.09;1.69); effect size 0.98.
Global joint pain
Pain reduced in both groups (). In the PT group, the median difference was 1.5 points, 95% CI <0.001 to 2.5; effect size 0.39. In the PT + CG group, the median difference was 3.5, 95% CI 1 to 6; effect size 0.79. The median between-group difference (Δ3) was 1, 95% CI −1 to 4; effect size 0.55.
Figure 3. Global joint pain at W0 and W4 in both groups. The global joint pain was measured using a Numeric Pain Rating Scale. 0: no pain and 10: intolerable pain.
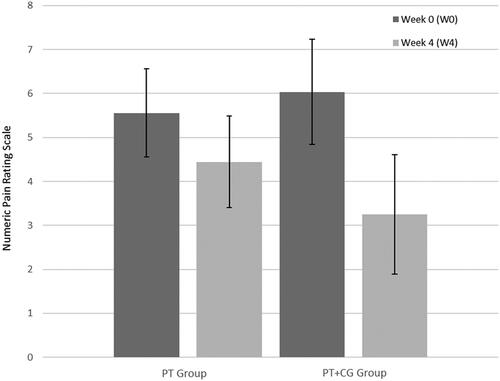
Discussion
This randomized controlled trial of the effect of wearing CG in addition to a physiotherapy program on balance measured in static and dynamic conditions on a force platform in people with hEDS found significant improvements in balance (i.e. sway velocity), immediately and after 4 weeks of use of CG, as hypothesised. Pain reduced to a larger extent in the PT + CG group, however, the between-group difference was not significantly different, in contrast with our hypothesis.
The results of this study extend those of the pilot study of the effects of CG and insoles on balance in people with hEDS described in the introduction [Citation16]. The results for the 6 participants included in that study showed an immediate decrease in sway area in static conditions with EO and EC [Citation16]. Those results together with the results of the present study suggest that wearing CG immediately improves postural stability in both static and dynamic conditions in people with hEDS.
Furthermore, after 4 weeks of intervention, there was a significant decrease in the medial-lateral condition with visual deprivation (LEC condition) for sway velocity (effect size: 0.93) and area (effect size: 0.45) which was greater in the PT + CG group than the PT group. Therefore, CG appear to improve stability in dynamic conditions [Citation16,Citation42]. Moreover, this effect may have been underestimated since the PT + CG group underwent fewer physiotherapy sessions than the PT group.
The LEC condition is particularly challenging for people with hEDS because it disturbs the balance in 2 different ways. Firstly, it is performed with no visual input, which is difficult for people with hEDS because they have an increased visual dependence for balance compared to healthy individuals [Citation6,Citation15,Citation16]. The results of the Romberg quotient (>1 at baseline), confirmed that the participants relied on vision to control their postural balance [Citation6,Citation16] in compensation for their proprioceptive impairments. Secondly, tasks on an unstable plate are known to induce instability during standing, requiring higher levels of sensory input to maintain balance, compared to a firm surface [Citation45]. With no visual input, participants had to rely more on their other sensory systems to maintain balance, especially proprioception [Citation7,Citation8,Citation46,Citation47].
These results confirm our hypothesis that physiotherapy combined with CG improves balance control in dynamic conditions. The differences between static and dynamic conditions could be explained by the fact that dynamic conditions allow better discrimination of postural variables [Citation47].
The effect of CG on balance on unstable surfaces in people with hEDS likely results from the exteroceptive input that compensates for proprioception impairments. Dupuy et al. suggested that the lack of visual information increases the requirement for information from other systems to maintain balance [Citation16]. The exteroceptive stimulation of cutaneous receptors provided by the CG has previously been found to be more effective in situations of increased sway, such as when standing on an unstable surface or in conditions of visual deprivation, than in more stable conditions [Citation48].
Loose ligaments and connective tissue disorders, which characterize hEDS, could influence intrinsic information on posture and movement, and could therefore lead to less adapted postural reactions [Citation6,Citation37]. The external pressure provided by the CG stimulates the cutaneous receptors, thus providing an additional source of feedback and improving sensorimotor responses [Citation34,Citation35,Citation49,Citation50]. The only pilot study assessing the effects of CG in people with hEDS suggested that this compensatory treatment could enhance the somatosensory information and thus improve balance [Citation16]. Moreover, studies in athletes and non-athletes without EDS demonstrated beneficial effects of CG on proprioception and balance [Citation34,Citation51,Citation52].
However, after 4 weeks, there was no between-group difference in sway velocity or area on the plate that was unstable in the anterior-posterior plane. The larger between-group difference in the medial-lateral instability condition could result from an effect of CG on ankle stability. The ankle joints are particularly affected by medial-lateral instability and injuries (recurrent sprains) in hEDS [Citation53]. Therefore, balancing on a medial-lateral oscillating plate could be particularly difficult and therefore more improved by CG than balancing on an anterior-posterior oscillating plate. At baseline, 88% of participants reported experiencing repeated ankle sprains.
Pain decreased in both groups at the end of the study; however, the between-group difference was not significant, suggesting that the CG did not have a specific effect on pain. An uncontrolled study of 36 people with hEDS found a global decrease in pain (from 3.5/10 to 2.5/10) after wearing a compressive jacket for 28 d; but, like the present study, the change was not significant [Citation36]. Some authors have suggested that the cutaneous input from the CG could have an analgesic effect via the gate control system [Citation34,Citation54]. However, the results of our study do not fully confirm that hypothesis.
Limitations and perspectives
This study has several limitations. Firstly, the sample size is small; however, in the domain of rare diseases, it is relatively large [Citation1,Citation2]. It would be interesting to determine if there is a long-term effect of wearing CG, and whether the effects remain after removing the CG. We did not perform assessments without the CG at 4 weeks, therefore we do not know if the immediate effect of the CG was maintained over time. Longer-term studies are needed to determine if people with hEDS should wear CG throughout their lives. Studies are also required to determine the functional long-term effects of CG on other dynamic balance tasks (such as complex locomotor tasks), falls, joint instability, quality of life, and autonomy. This study was not designed to evaluate the effectiveness of the physiotherapy. The minimal number of hours of daily wearing to observe efficacy is unknown, the participants in this study wore the CG on average 10 h 40 per day and tolerance was good; further studies should determine the appropriate wearing time.
Conclusions
This randomized controlled trial is the first to assess the effects of CG in adjunction to physiotherapy on balance in people with hEDS. Balance variables were evaluated in static and dynamic conditions, immediately and after 4-weeks of intervention. The results seem to support the use of CG combined with a physiotherapy program to improve dynamic balance in people with hEDS. Currently available treatments used by EDS experts are only symptomatic or preventive, designed to avoid complications; no curative treatment exists. This study brings some new insights into the efficacy of CG in hEDS. Future studies are necessary to confirm the functional impact of CG and their benefits on joint complications, especially for ankle sprains.
Acknowledgements
We would like to thank patients with hEDS who participated in this study; Lyvia Sala and Martin Peschanski, physiotherapists, and Camille Jarnet, nurse, for their great contribution to the acquisition of the data. We thank Lara Jorge for her contributions to data analysis, interpretation, writing, editing, and revision of the manuscript. We thank Johanna Robertson for language editing.
Disclosure statement
No potential conflict of interest was reported by the author(s).
Additional information
Funding
References
- Malfait F, Francomano C, Byers P, et al. The 2017 international classification of the Ehlers–Danlos syndromes. Am J Med Genet C Semin Med Genet. 2017;175(1):5–7.
- Tinkle B, Castori M, Berglund B, et al. Hypermobile Ehlers–Danlos syndrome (a.k.a. Ehlers–danlos syndrome type III and Ehlers–Danlos syndrome hypermobility type): clinical description and natural history. Am J Med Genet C Semin Med Genet. 2017;175(1):48–69.
- Castori M, Tinkle B, Levy H, et al. A framework for the classification of joint hypermobility and related conditions. Am J Med Genet C Semin Med Genet. 2017;175(1):148–157.
- Bénistan K, Martinez V. Pain in hypermobile Ehlers-Danlos syndrome: new insights using new criteria. Am J Med Genet A. 2019;179(7):1226–1234.
- Galli M, Cimolin V, Rigoldi C, et al. Gait strategy in patients with Ehlers-Danlos syndrome hypermobility type: a kinematic and kinetic evaluation using 3D gait analysis. Res Dev Disabil. 2011;32(5):1663–1668.
- Rombaut L, Malfait F, De Wandele I, et al. Balance, gait, falls, and fear of falling in women with the hypermobility type of Ehlers-Danlos syndrome. Arthritis Care Res. 2011;63(10):1432–1439.
- Clayton HA, Cressman EK, Henriques DYP. Proprioceptive sensitivity in Ehlers-Danlos syndrome patients. Exp Brain Res. 2013;230(3):311–321.
- Rombaut L, De Paepe A, Malfait F, et al. Joint position sense and vibratory perception sense in patients with Ehlers-Danlos syndrome type III (hypermobility type). Clin Rheumatol. 2010;29(3):289–295.
- Ferrell WR, Tennant N, Sturrock RD, et al. Amelioration of symptoms by enhancement of proprioception in patients with joint hypermobility syndrome. Arthritis Rheum. 2004;50(10):3323–3328.
- Smith TO, Jerman E, Easton V, et al. Do people with benign joint hypermobility syndrome (BJHS) have reduced joint proprioception? A systematic review and meta-analysis. Rheumatol Int. 2013;33(11):2709–2716. Springer
- Scheper M, Rombaut L, de Vries J, et al. The association between muscle strength and activity limitations in patients with the hypermobility type of Ehlers–Danlos syndrome: the impact of proprioception. Disabil Rehabil. 2017;39(14):1391–1397.
- Sahin N, Baskent A, Cakmak A, et al. Evaluation of knee proprioception and effects of proprioception exercise in patients with benign joint hypermobility syndrome. Rheumatol Int. 2008;28(10):995–1000.
- Rombaut L, Malfait F, De Wandele I, et al. Muscle mass, muscle strength, functional performance, and physical impairment in women with the hypermobility type of Ehlers-Danlos syndrome. Arthritis Care Res. 2012;64(10):1584–1592.
- Voermans NC, Knoop H, van de Kamp N, et al. Fatigue is a frequent and clinically relevant problem in Ehlers-Danlos syndrome. Semin Arthritis Rheum. 2010;40(3):267–274.
- Galli M, Rigoldi C, Celletti C, et al. Postural analysis in time and frequency domains in patients with Ehlers-Danlos syndrome. Res Dev Disabil. 2011;32(1):322–325.
- Dupuy EG, Leconte P, Vlamynck E, et al. Ehlers-Danlos syndrome, hypermobility type: impact of somatosensory orthoses on postural control (a pilot study). Front Hum Neurosci. 2017;11:21–33. DOI:10.3389/fnhum.2017.00283
- Bates AV, McGregor AH, Alexander CM. Prolonged standing behaviour in people with joint hypermobility syndrome. BMC Musculoskelet Disord. 2021;22(1):1–8.
- Schubert-Hjalmarsson E, Öhman A, Kyllerman M, et al. Pain, balance, activity, and participation in children with hypermobility syndrome. Pediatr Phys Ther. 2012;24(4):339–344.
- Engelbert RHH, Juul-Kristensen B, Pacey V, et al. The evidence-based rationale for physical therapy treatment of children, adolescents, and adults diagnosed with joint hypermobility syndrome/hypermobile Ehlers Danlos syndrome. Am J Med Genet C Semin Med Genet. 2017;175(1):158–167.
- Hakimi A, Bergoin C, Mucci P. Immediate and 6-week after effects of a rehabilitation program for Ehlers–Danlos syndrome hypermobile type patients: a retrospective study. Am J Med Genet A. 2020;182(10):2263–2271.
- Reychler G, De Backer MM, Piraux E, et al. Physical therapy treatment of hypermobile Ehlers–Danlos syndrome: a systematic review. Am J Med Genet. 2021;185(10):2986–2994.
- Chopra P, Tinkle B, Hamonet C, et al. Pain management in the Ehlers–Danlos syndromes. Am J Med Genet C Semin Med Genet. 2017;175(1):212–219.
- Keer R, Simmonds J. Joint protection and physical rehabilitation of the adult with hypermobility syndrome. Curr Opin Rheumatol. 2011;23(2):131–136.
- Simmonds JV, Herbland A, Hakim A, et al. Exercise beliefs and behaviours of individuals with joint hypermobility syndrome/Ehlers–Danlos syndrome–hypermobility type. Disabil Rehabil. 2019;41(4):445–455.
- Palmer S, Terry R, Rimes KA, et al. Physiotherapy management of joint hypermobility syndrome – a focus group study of patient and health professional perspectives. Physiother. 2016;102(1):93–102.
- Castori M, Morlino S, Celletti C, et al. Management of pain and fatigue in the joint hypermobility syndrome (a.k.a. Ehlers-Danlos syndrome, hypermobility type): principles and proposal for a multidisciplinary approach. Am J Med Genet A. 2012;158A(8):2055–2070.
- Bathen T, Hångmann AB, Hoff M, et al. Multidisciplinary treatment of disability in ehlers-danlos syndrome hypermobility type/hypermobility syndrome: a pilot study using a combination of physical and cognitive-behavioral therapy on 12 women. Am J Med Genet. 2013;161(12):3005–3011.
- Ferrell WR, Tennant N, Baxendale RH, et al. Musculoskeletal reflex function in the joint hypermobility syndrome. Arthritis Rheum. 2007;57(7):1329–1333.
- Laferrier JZ, Muldowney K, Muldowney K. A novel exercise protocol for individuals with Ehlers Danlos syndrome: a case report. J Nov Physiother. 2018;8(2):382.
- Liaghat B, Skou ST, Jørgensen U, et al. Heavy shoulder strengthening exercise in people with hypermobility spectrum disorder (HSD) and long-lasting shoulder symptoms: a feasibility study. Pilot Feasibility Stud. 2020;6(1):97.
- Daman M, Shiravani F, Hemmati L, et al. The effect of combined exercise therapy on knee proprioception, pain intensity and quality of life in patients with hypermobility syndrome: a randomized clinical trial. J Bodyw Mov Ther. 2019;23(1):202–205.
- Rombaut L, Malfait F, De Wandele I, et al. Medication, surgery, and physiotherapy among patients with the hypermobility type of Ehlers-Danlos syndrome. Arch Phys Med Rehabil. 2011;92(7):1106–1112.
- Ghai S, Driller MW, Masters RSW. The influence of below-knee compression garments on knee-joint proprioception. Gait Posture. 2018;60:258–261.
- Ghai S, Driller M, Ghai I. Effects of joint stabilizers on proprioception and stability: a systematic review and meta-analysis. Phys Ther Sport. 2017;25:65–75.
- Angelakos I, Mills C, O’Halloran J. The effects of compression garments on stability and lower limb kinematics during a forward lunge. J Hum Kinet. 2020;71(1):59–68.
- Chaléat-Valayer E, Denis A, Zelmar A, et al. VETCOSED study: efficacy of compressive garments for patients with hypermobile Ehlers–Danlos syndrome on shoulder stability and muscles strength. Disabil Rehabil. 2020;44:3165–3172.
- Rigoldi C, Cimolin V, Camerota F, et al. Measuring regularity of human postural sway using approximate entropy and sample entropy in patients with Ehlers-Danlos syndrome hypermobility type. Res Dev Disabil. 2013;34(2):840–846.
- Scheper MC, Pacey V, Rombaut L, et al. Generalized hyperalgesia in children and adults diagnosed with hypermobility syndrome and Ehlers-Danlos syndrome hypermobility type: a discriminative analysis. Arthritis Care Res. 2017;69(3):421–429.
- Juul-Kristensen B, Schmedling K, Rombaut L, et al. Measurement properties of clinical assessment methods for classifying generalized joint hypermobility—a systematic review. Am J Med Genet C Semin Med Genet. 2017;175(1):116–147.
- Ruhe A, Fejer R, Walker B. Center of pressure excursion as a measure of balance performance in patients with non-specific low back pain compared to healthy controls: a systematic review of the literature. Eur Spine J. 2011;20(3):358–368.
- Gagey P-M. Recording conditions – Normes13. Man Ther Posturology Rehabil J. 2016;14:1–3.
- Pinsault N, Vuillerme N. Test-retest reliability of centre of foot pressure measures to assess postural control during unperturbed stance. Med Eng Phys. 2009;31(2):276–286.
- Scoppa F, Capra R, Gallamini M, et al. Clinical stabilometry standardization. Basic definitions – acquisition interval – sampling frequency. Gait Posture. 2013;37(2):290–292.
- Cohen J. Statistical power analysis for the behavioural sciences. Hillside (NJ): Lawrence Earlbaum Associates; 1988.
- Silva P de B, Mrachacz-Kersting N, Oliveira AS, et al. Effect of wobble board training on movement strategies to maintain equilibrium on unstable surfaces. Hum Mov Sci. 2018;58:231–238.
- Mallik AK, Ferrell WR, Mcdonald AG, et al. Impaired proprioceptive acuity at the proximal interphalangeal joint in patients with the hypermobility syndrome. Br J Rheumatol. 1994;33(7):631–637.
- Paillard T, Noé F. Techniques and methods for testing the postural function in healthy and pathological subjects. Biomed Res Int. 2015;2015:1–15.
- Baige K, Noé F, Bru N, et al. Effects of compression garments on balance control in young healthy active subjects: a hierarchical cluster analysis. Front Hum Neurosci. 2020;14:582514.
- Wong DWC, Lam WK, Chen TLW, et al. Effects of upper-limb, lower-limb, and full-body compression garments on full body kinematics and free-throw accuracy in basketball players. Appl Sci. 2020;10(10):3504.
- Baige K, Noé F, Paillard T. Wearing compression garments differently affects monopodal postural balance in high-level athletes. Sci Rep. 2020;10(1):1–7.
- Woo MT, Davids K, Liukkonen J, et al. Immediate effects of wearing knee length socks differing in compression level on postural regulation in community-dwelling, healthy, elderly men and women. Gait Posture. 2018;66:63–69.
- Michael JS, Dogramaci SN, Steel KA, et al. What is the effect of compression garments on a balance task in female athletes? Gait Posture. 2014;39(2):804–809.
- Ericson WB, Wolman R. Orthopaedic management of the Ehlers–Danlos syndromes. Am J Med Genet C Semin Med Genet. 2017;175(1):188–194.
- Melzack R, Wall PD. Pain mechanisms: a new theory. Science. 1965;150(3699):971–979.