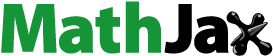
Abstract
The tolerance of the entomophagous fungus Lecanicillium muscarium to eight pesticides using a poisoned medium method in the laboratory was evaluated. The purpose was to identify compatible combinations between biopesticides based on pathogenic fungi and conventional pesticides. Pyridalyl slightly inhibited the germination of L. muscarium spores and very slightly inhibited hyphal growth at low concentrations. The maximum inhibition rate of pyridalyl was <30%. Difenoconazole very slightly inhibited the germination of L. muscarium of conidia, and thiamethoxam and dimethomorph both very slightly inhibited hyphal growth. In most treatments, the maximum inhibition rates of difenoconazole, dimethomorph, or thiamethoxam were <20%. Phoxim at a low concentration slightly inhibited both L. muscarium conidial germination and hyphal growth (maximum inhibition rate, <25%). The minimum inhibition rate of most chlorothalonil, triadimefon, or tetraconazole treatments exceeded 60%. At low concentrations, pyridalyl and phoxim showed weak inhibitory effects, and therefore, may be compatible with L. muscarium. These data will support recommendations for the use of pesticides in integrated pest management programmes that involve L. muscarium as the biocontrol agent.
1. Introduction
Biopesticides based on fungi as biological control agents are important pest management tools because most of them can be used to avoid the negative effects of synthetic pesticides (e.g. environmental pollution and killing the natural enemies of pests) (Marrone Citation2019). However, most biopesticides based on fungi have limited shelf-lives, act slowly, and have narrow target ranges (Faria and Wraight Citation2001; Jackson, Dunlap, and Jaronski Citation2010). Combining an effective biopesticide based on fungi and compatible pesticides in an integrated pest management (IPM) programme offers promise (Sain et al. Citation2019). It is necessary to investigate the effects of synthetic pesticides on various entomopathogenic fungi to determine which fungi and pesticides can be combined successfully in such programmes.
The entomopathogenic fungus Lecanicillium muscarium Zare and Gams (previously called Verticillium lecanii [Zimmerman]) are widely distributed ascomycete fungi of the order Hypocreales. Colonies of L. muscarium on PDA agar media grow white or pale yellow, cottony or velvety, turning cream in colour with mealy appearance (Upadhyay et al. Citation2014). The Lecanicillium genus is notable in that conidia are borne in slime balls and rarely in dry chains, unlike the other Hyprocreales fungi, which produce hydrophobic conidia (Jaronski Citation2014). L. muscarium can naturally infects various sucking pests (e.g. aphids, mites, thrips, and whiteflies) and plant-pathogenic nematodes that are economically important pests of horticultural crops (Gillespie Citation1986; Hazarika et al. Citation2009; Jackson, Heale, and Hall Citation2008; Meyer and Huettel Citation1993; Wang et al. Citation2004; Hamdi et al. Citation2011). L. muscarium could also be used as an effective hyperparasite of several rust and powdery mildew fungi (Allen Citation1982; Verhaar, Hijwegen, and Zadoks Citation1996; Askary et al. Citation1998). Some L. muscarium strains are very virulent and have high epizootic efficiencies against certain insects. Such strains have been used as commercial mycoinsecticides against aphids and whitefly (Hall Citation1981a, Citation1981b; Quinlan Citation1988).
Some studies on the compatibility of L. muscarium with pesticides have been published (Cuthbertson et al. Citation2010; Cuthbertson, Walters, and Deppe Citation2005; Hall Citation1981a, Citation1981b). However, the effects of some currently used pesticides on L. muscarium have not been evaluated, and different pesticides inhibit the germination and mycelial growth of entomopathogenic fungi species and strains to varying degrees (Anderson et al. Citation1989; Poprawski and Majchrowicz Citation1995; Todorova et al. Citation1998). In addition, the formulation of a pesticide will markedly affect its compatibility with fungi (Inglis et al. Citation2016; Morjan, Pedigo, and Lewis Citation2002; Celar and Kos Citation2020). In this study, the tolerance of L. muscarium Beijing-P strain to each of eight chemical pesticides that are currently used to control insect pests and diseases were examined to address whether these chemicals inhibit the conidial germination and hyphal growth of L. muscarium. The results will provide data for further studies on interaction between the pathogen and chemical pesticides, as well as their applications in integrated pests management programs.
2. Materials and methods
2.1. Fungus and pesticides
The L. muscarium strain Beijing-P was originally isolated from Trialeurodes vaporariorum on radish planted in a greenhouse of Beijing, China in 1986. The fungus was cultured on potato dextrose agar (PDA), which contained 200 g L−1 potato infusion, 20 g L−1 dextrose, and 20 g L−1 agar. The fungus was incubated at 23 °C in the dark. Eight synthetic pesticides were acquired from a local market for use in these experiments. The compatibility of each pesticide with the fungus was tested by performing in vitro bioassays for each pesticide at five concentrations. We used the recommended concentration for use in the field (R), a quarter of the recommended concentration (R/4), half of the recommended concentration (R/2), double the recommended concentration (2 R), and four times the recommended concentration (4 R). The active ingredients, formulations, suppliers, and chemical classifications of the pesticides used are shown in .
Table 1. Pesticides tested against Lecanicillium muscarium in this study.
2.2. Hyphal growth inhibition
A solution of the pesticide at the desired concentration was added to melted PDA medium (100 mL) in a flask and mixed thoroughly at 48 °C before solidification. About 20 mL of the medium was then added to each of a series of sterile Petri dishes (9 cm diameter). Each Petri dish was inoculated with a 7.5 mm diameter disc of a L. muscarium culture, which was placed in the centre using a sterile toothpick. The culture disc was removed 7 d later old L. muscarium culture on PDA. Three to six replicate Petri dishes were prepared for each pesticide concentration, and PDA medium containing no pesticides was used as the control. The Petri dishes were incubated at 23 °C for 5 d, then the diameter of the colony on each dish was determined using the cross method, using the mean of three measurements.
2.3. Inhibition of conidial germination
The fungus was cultured on PDA at 23 °C in the dark for 7 d. Fungi were harvested by rinsing the culture plate with sterile distilled water containing 0.1% v/v Tween 80, vortexing the conidial suspension for 2 min, and then passing the mixture through a single layer of gauze to collect the mycelia. The conidial concentration in the suspension was estimated using a microscope with a blood cell counting chamber, and then the concentration was adjusted to the desired concentration (2 × 106 conidia mL−1) by adding sterile distilled water. Each pesticide was dissolved in sterile distilled water containing 0.1% v/v Tween 80, and then the solution volume was adjusted to give the required pesticide concentration. For each test, an aliquot of the conidial suspension was mixed with a pesticide solution and 4% w/v glucose medium (1 + 1 + 1 by volume), and then the mixture was spread on a sterile glass slide with grooves. The slide was placed on sterile wet filter paper in a sterile Petri dish (9 cm diameter) and incubated at 23 °C in the dark. The conidial suspension with no pesticides was used as a control. Three or four replicate samples at each concentration were prepared. The percentage of conidia that germinated was determined after 24 h. Between 100 and 200 conidia (randomly selected) in each Petri dish were counted. Conidia were considered to have germinated if the germ tube was longer than the conidial diameter.
2.4. Statistical analyses
Conidial germination inhibition and hyphae growth inhibition (each as a percentage) in each treatment relative to the control were determined as described by Hokkanen and Kotiluoto (Citation1992), using the following equation:
where I, C, and P are percentage conidial germination inhibition or hyphae growth inhibition, conidial germination or hyphal growth of the fungus in the controls, and conidial germination or hyphae growth of the fungus in the medium containing pesticide, respectively. An arcsine square-root transformation of the percentage germination inhibition or hyphal growth inhibition data was performed before the data were subjected to one-way analysis of variance. Means were compared using Turkey’s multiple range tests (p = 0.05). The statistical analyses were performed using IBM SPSS statistics 20.0 software (IBM, Armonk, NY, USA).
3. Results and discussion
3.1. L. muscarium tolerates pyridalyl
Pyridalyl at the recommended concentration (R) and the two low concentrations (R/4 and R/2) very weakly inhibited L. muscarium hyphal growth, but at high concentrations (4 R and 2 R) it strongly inhibited hyphae growth (). The hyphal growth inhibition rates differed significantly among the five pyridalyl treatments (F = 83.28, df = 4, p = 0.002 < 0.05) (). All five pyridalyl treatments very weakly inhibited L. muscarium conidial germination, and significant inhibition was found at higher pyridalyl concentrations (F = 8.91, df = 4, p < 0.0001) (). The main targets of pyridalyl are lepidopteran pests, such as Plutella xylostella, an important pest of cruciferous vegetables, whereas the hosts of L. muscarium, such as aphid, thrip, and whitefly are also important pests of cruciferous vegetables. Therefore, the combination of these two agents could complementally control more insect pests and reduce application costs.
Figure 1. Inhibition of Lecanicillium muscarium conidial germination and hyphal growth by pyridalyl at different concentrations. R, R/4, R/2, 2R, and 4R indicate recommended concentration for use, a quarter of the recommended concentration, half of the recommended concentration, double the recommended concentration, and four times the recommended concentration, respectively. Colony morphology of L. muscarium grown on potato dextrose agar (PDA) containing pyridalyl at different concentrations at 5 d after inoculation (A). The plates were 9 cm in diameter. Control (PDA medium) did not contain any pesticides (A). Conidial germination inhibition rate and hyphal growth inhibition rate were determined relative to that in the control (see Materials and Methods) (B). In the box chart, uppermost point, lowest point, upper box edge, lower box edge, and line within the box represent the maximum, minimum, upper quartile, lower quartile, and median of three or four replicates, respectively. A single line in the box chart indicates 100% inhibition rate in all replicates. Data were arcsine square-root transformed before one-way analysis of variance. Means were compared using Turkey’s multiple range test. Different letters indicate significant differences among treatments (p < 0.05).
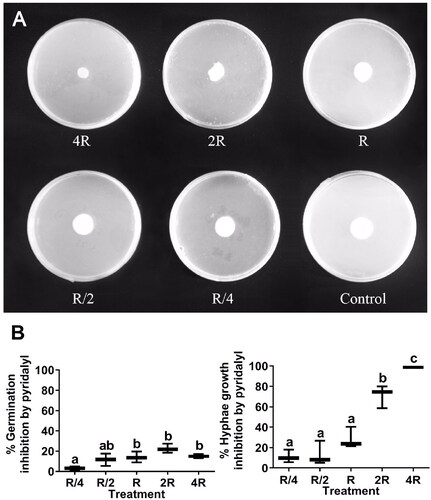
3.2. L. muscarium spores or hyphae tolerate difenoconazole, thiamethoxam, and dimethomorph
Difenoconazole completely inhibited L. muscarium hyphal growth, only very weakly inhibited conidial germination (). Neither hyphae growth nor conidial germination differed significantly among the five difenoconazole test concentrations (). Thiamethoxam and dimethomorph both strongly inhibited L. muscarium conidial germination, but only very weakly inhibited hyphal growth (). Notably, hyphal growth was enhanced in some of the thiamethoxam and dimethomorph treatments (). The conidial germination inhibition rates differed significantly among the five thiamethoxam treatments (F = 21.31, df = 4, p < 0.0001) (), but the hyphal growth inhibition rates did not. The hyphal growth inhibition rates differed significantly among the five dimethomorph treatments (F = 4.95, df = 4, p = 0.0053 < 0.05), so did the conidial germination inhibition rates (F = 186.9, df = 4, p < 0.0001) (). Previous studies have reported similar results for the effect of thiamethoxam on L. muscarium hyphal growth but contrasting results for its effect on conidial germination (Gurulingappa, Gee, and Sword Citation2011). To our knowledge, no other studies have explored the compatibility of L. muscarium with difenoconazole and dimethomorph. In the present study, we investigated the inhibitory effects on L. muscarium by direct exposure to pesticides. However, different inhibitory effects may occur when L. muscarium is exposed to pesticides sequentially. It was reported that copper oxychloride stimulated the insecticidal properties of Beauveria bassiana when it was used 2–4 days after B. bassiana application. However, when copper oxychloride was applied 2–4 days before, or in combination with B. bassiana, it showed inhibitory effects (Kouassi, Coderre, and Todorova Citation2003). Some IPM schemes require pesticides and entomopathogenic fungi to be applied sequentially, rather than simultaneously, under field conditions. In another study, exposure of L. muscarium to spiromesifen for 24 h resulted in very low conidial germination, at levels unsuitable for commercial application, but infectivity (hyphal growth) was not negatively affected when L. muscarium was applied to plants that had been sprayed with spiromesifen 24 h previously (Cuthbertson et al. Citation2008). In the present study, thiamethoxam and dimethomorph both strongly inhibited L. muscarium conidial germination, but only weakly inhibited hyphal growth. Based on this, these two pesticides are probably compatible with L. muscarium if applied sequentially in the field. The insecticidal spectra of thiamethoxam and L. muscarium are similar, so this study will lay the foundation for further research on whether the combination of this two pesticides can have a synergistic effect. Furthermore, whether the combination of thiamethoxam and L. muscarium can accelerate pests to produce resistance because of dosage reduction in the mixture need to be researched further. The main targets of dimethomorph are oomycete fungi that cause various plant diseases such as downy mildew, and late blight in cucumber, tomato, and grape. The hosts of L. muscarium, such as aphid, thrip, and whitefly are also important pests of these economic plants. Therefore, the combination of these two agents could control of plant diseases and insect pests simultaneously. In addition, the phenomenon that pesticides can strongly inhibited L. muscarium conidial germination, but only weakly inhibited hyphal growth is interesting and the mechanisms needed to be solved in future.
Figure 2. Inhibition of Lecanicillium muscarium conidial germination and hyphal growth by difenoconazole, thiamethoxam, and dimethomorph at different concentrations. Colony morphology of L. muscarium grown on potato dextrose agar (PDA) containing difenoconazole (A) and thiamethoxam (B) at different concentrations at 5 d after inoculation.The plates were 9 cm in diameter. Different letters indicate significant differences among treatments (p < 0.05) (C). The meanings of R, R/4, R/2, 2R, 4R and box chart, as well as data processing methods are all the same as .
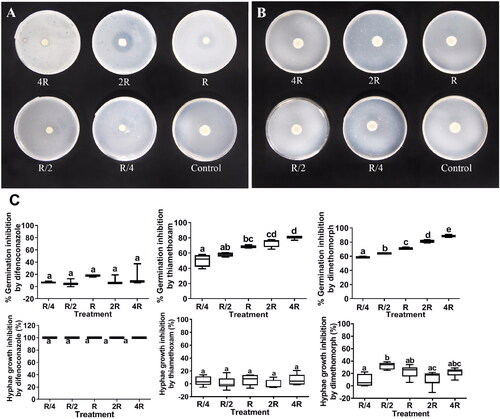
3.3. L. muscarium tolerates phoxim at low concentrations
Phoxim at low concentrations (R/4 and R/2) weakly inhibited L. muscarium conidial germination and hyphal growth (). The hyphal growth inhibition rates increased at higher concentrations (F = 165.70, df = 4, p < 0.0001), as did the conidial germination inhibition rates (F = 143.90, df = 4, p < 0.0001) (). The main targets of phoxim are lepidopteran insects pests, whereas L. muscarium are against homopetran insect pests. Therefore, the combination of these two agents could control of this two orders of insect pests simultaneously.
Figure 3. Inhibition of L. muscarium conidial germination and hyphal growth by phoxim at different concentrations. Colony morphology of L. muscarium grown on potato dextrose agar (PDA) containing phoxim at different concentrations at 5 d after inoculation (A). The plates were 9 cm in diameter. Different letters indicate significant differences among treatments (p < 0.05) (B). The meanings of R, R/4, R/2, 2 R, 4 R and box chart, as well as data processing methods are all the same as .
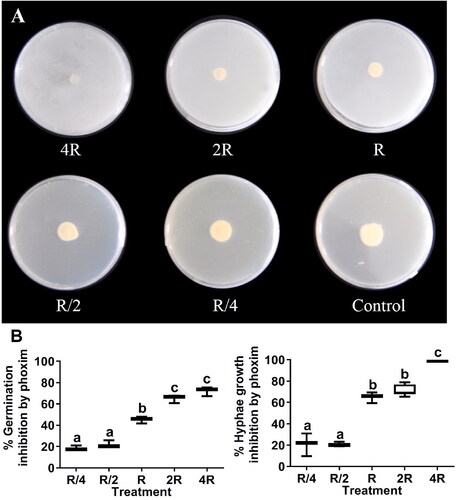
3.4. L. muscarium does not tolerate chlorothalonil, triadimefon, or tetraconazole
Chlorothalonil strongly inhibited both conidial germination and hyphal growth. The conidial germination inhibition rates differed significantly among the five chlorothalonil treatments (F = 146.10, df = 4, p < 0.0001), as did the hyphal growth inhibition rates (F = 5.2, df = 4, p < 0.0001) (). Similar results were found in a study performed by Ali et al. (Citation2012), but in another study, chlorothalonil was found to be very toxic and completely inhibited L. muscarium conidial germination and hyphal growth (Krishnamoorthy et al. Citation2007). Triadimefon and tetraconazole both extremely strongly inhibited L. muscarium conidial germination and hyphal growth, particularly hyphal growth, for which the inhibition rate was 100% (). The conidial germination inhibition rates differed significantly among the five triadimefon treatments (F = 4.06, df = 4, p = 0.0035 < 0.05), and among the five tetraconazole treatments (F = 3.43, df = 4, p = 0.0457 < 0.05). The hyphal growth inhibition rates did not differ significantly among the five triadimefon treatments or among the five tetraconazole treatments (). The results of a study conducted by Ali et al. showed that the triazole fungicides myclobutanil and propiconazole strongly decreased L.muscarium hyphal growth, but did not completely inhibit it (Ali et al. Citation2012).
Figure 4. Inhibition of Lecanicillium muscarium conidial germination and hyphal growth by chlorothalonil, triadimefon, and tetraconazole at different concentrations. The meanings of R, R/4, R/2, 2 R, 4 R and box chart, as well as data processing methods are all the same as . Different letters indicate significant differences among treatments (p < 0.05).
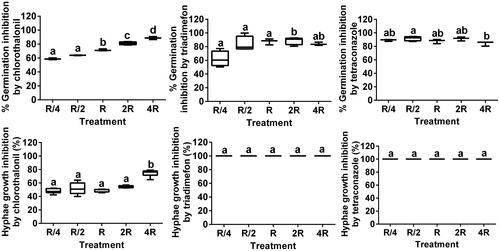
4. Conclusion
In summary, L. muscarium tolerated pyridalyl and phoxim (at low concentrations), both in terms of its conidial germination and hyphal growth. The conidial germination of L. muscarium, but not its hyphal growth, was inhibited by thiamethoxam and dimethomorph. On the basis of our results, we conclude that three insecticides, pyridalyl, phoxim, thiamethoxam, and one fungicide, dimethomorph, could be compatible with L. muscarium. These data will support recommendations for the use of pesticides in IPM programmes involving L. muscarium as the biocontrol agent.
Disclosure statement
No potential conflict of interest was reported by the authors.
Additional information
Funding
References
- Ali, S., Z. Huang, S. Zou, M. H. Bashir, Z. Wang, and S. Ren. 2012. “Effect of Fungicides on Growth, Germination and Cuticle-Degrading Enzyme Production by Lecanicillium muscarium.” Biocontrol Science and Technology 22 (9): 1047–1058. doi: 10.1080/09583157.2012.708394.
- Allen, D. J. 1982. “Verticillium Lecanii on the Bean Rust Fungus, Uromyces appendiculatus.” Transactions of the British Mycological Society 79 (2): 362–364. doi: 10.1016/S0007-1536(82)80132-0.
- Anderson, T. E., A. E. Hajek, D. W. Roberts, H. K. Preisler, and J. L. Robertson. 1989. “Colorado Potato Beetle (Coleoptera: Chrysomelidae): Effects of Combinations of Beauveria bassiana with Insecticides.” Journal of Economic Entomology 82 (1): 83–89. doi: 10.1093/jee/82.1.83.
- Askary, H., Y. Carriere, R. Belanger, and J. Brodeur. 1998. “Pathogenicity of the Fungus Verticillium Lecanii to Aphids and Powdery Mildew.” Biocontrol Science and Technology 8 (1): 23–32. doi: 10.1080/09583159830405.
- Celar, F., and K. Kos. 2020. “In Vitro Compatibility of Beauveria bassiana Strain ATCC 74040 with Copper Fungicides.” Journal of Applied Entomology 144: 1–8.
- Cuthbertson, A. G. S., L. F. Blackburn, P. Northing, W. Luo, R. J. C. Cannon, and K. F. A. Walters. 2008. “Further Compatibility Tests of the Entomopathogenic Fungus Lecanicillium muscarium with Conventional Insecticide Products for Control of Sweetpotato Whitefly, Bemisia tabaci on Poinsettia Plants.” Insect Science 15 (4): 355–360. doi: 10.1111/j.1744-7917.2008.00221.x.
- Cuthbertson, A. G. S., L. F. Blackburn, P. Northing, W. Luo, R. J. C. Cannon, and K. F. A. Walters. 2010. “Chemical Compatibility Testing of the Entomopathogenic Fungus Lecanicillium Muscarium to Control Bemisia tabaci in Glasshouse Environment.” International Journal of Environmental Science & Technology 7 (2): 405–409. doi: 10.1007/BF03326150.
- Cuthbertson, A. G. S., K. F. A. Walters, and C. Deppe. 2005. “Compatibility of the Entomopathogenic Fungus Lecanicillium Muscarium and Insecticides for Eradication of Sweetpotato Whitefly, Bemisia tabaci.” Mycopathologia 160 (1): 35–41. doi: 10.1007/s11046-005-6835-4.
- Faria, M., and S. P. Wraight. 2001. “Biological Control of Bemisia tabaci with Fungi.” Crop Protection 20 (9): 767–778. doi: 10.1016/S0261-2194(01)00110-7.
- Gillespie, A. T. 1986. “The Potential of Entomogenous Fungi as Control Agents for Onion Thrips, Thrips Tabaci.” British Crop Protection Council 34: 237–243.
- Gurulingappa, P., P. M. Gee, and G. A. Sword. 2011. “In Vitro and in Planta Compatibility of Insecticides and the Endophytic Entomopathogen, Lecanicillium lecanii.” Mycopathologia 172 (2): 161–168. doi: 10.1007/s11046-011-9410-1.
- Hall, R. A. 1981a. “Laboratory Studies on the Effects of Fungicides, Acaricides and Insecticides on the Entomopathogenic Fungus, Verticillium Lecanii.” Entomologia Experimentalis et Applicata 29 (1): 39–48. doi: 10.1111/j.1570-7458.1981.tb03040.x.
- Hall, R. A. 1981b. “The Fungus Verticillium Lecanii as a Microbial Insecticide against Aphids and Scales.” In Microbial Control of Pests and Plant Diseases, edited by H. D. Burges, 483–498. London, UK: Academic Press.
- Hamdi, F., J. Fargues, G. Ridray, B. Jeannequin, and O. Bonato. 2011. “Compatibility among Entomopathogenic Hyphocreales and Two Beneficial Insects Used to Control Trialeurodes vaporariorum (Hemiptera: Aleurodidae) in Mediterranean Greenhouses.” Journal of Invertebrate Pathology 108 (1): 22–29. doi: 10.1016/j.jip.2011.05.018.
- Hazarika, L. K., K. C. Puzari, S. Kalita, P. Das, and S. Saikia. 2009. “In Vitro Efficacy of Botanicals in Combination with Verticillium lecanii (Zimm.) Viegas for Management of Red Spider Mite in Tea.” Pesticide Research Journal 21: 16–21.
- Hokkanen, H. M. T., and R. Kotiluoto. 1992. “Bioassay of the Side Effects of Pesticides on Beauveria bassiana and Metarhizium Anisopliae: Standardized Sequential Testing Procedure.” Wld Pestic Rec Sci Bull 11: 148–151.
- Inglis, G. D., M. S. Goettel, T. M. Butt, and H. Strasser. 2016. “Use of Hyphomycetous Fungi for Managing Insect Pests.” In Fungi as Biocontrol Agents: Progress, Problems and Potential, edited by T. M. Butt, C. Jackson, and N. Magan, 23–69. Wallingford, UK: CABI Publishing.
- Jackson, C. W., J. B. Heale, and R. A. Hall. 2008. “Traits Associated with Virulence to the Aphid Macrosiphoniella sanborni in Eighteen Isolates of Verticillium lecanii.” Annals of Applied Biology 106 (1): 39–48. doi: 10.1111/j.1744-7348.1985.tb03092.x.
- Jackson, M. A., C. A. Dunlap, and S. T. Jaronski. 2010. “Ecological Considerations in Producing and Formulating Fungal Entomopathogens for Use in Insect Biocontrol.” BioControl 55 (1): 129–145. doi: 10.1007/s10526-009-9240-y.
- Jaronski, S. T. 2014. “Mass Production of Entomopathogenic Fungi: State of the Art.” In Mass Production of Beneficial Organisms Invertebrates and Entomopathogens, edited by J. Morales-Ramos, M. Rojas, and D. Shapiro-Ilan, 357–413. New York, USA: Academic Press.
- Kouassi, M., D. Coderre, and S. I. Todorova. 2003. “Effects of the Timing of Applications on the Incompatibility of Three Fungicides and One Isolate of the Entomopathogenic Fungus Beauveria bassiana (Balsamo) Vuillemin (Deuteromycotina).” Journal of Applied Entomology 127 (7): 421–426. doi: 10.1046/j.1439-0418.2003.00769.x.
- Krishnamoorthy, A., P. N. G. Visalakshi, A. M. Kumar, and M. Mani. 2007. “Influence of Some Pesticides on Entomopathogenic Fungus Lecanicillium (=Verticillium) Lecanii (Zimm.) ZARE & GAMS.” Journal of Horticultural Sciences 2 (1): 53–57. doi: 10.24154/jhs.v2i1.639.
- Marrone, P. G. 2019. “Pesticidal Natural Products – Status and Future Potential.” Pest Management Science 75 (9): 2325–2340. doi: 10.1002/ps.5433.
- Meyer, S. L. F, and R. N. Huettel. 1993. “Fungi and Fungus/Bioregulator Combinations for Control of Plant-Parasitic Nematodes.” In Pest Management: Biologically Based Technologies, edited by R. D. Lumsden, and J. L. Vaughn, 214–221. New York, USA: ACS Publications.
- Morjan, W. E., L. P. Pedigo, and L. C. Lewis. 2002. “Fungicidal Effects of Glyphosate and Glyphosate Formulations on Four Species of Entomopathogenic Fungi.” Environmental Entomology 31 (6): 1206–1212. doi: 10.1603/0046-225X-31.6.1206.
- Poprawski, T. J., and I. Majchrowicz. 1995. “Effects of Herbicides on in Vitro Vegetative Growth and Sporulation of Entomopathogenic Fungi.” Crop Protection 14 (1): 81–87. doi: 10.1016/0261-2194(95)91116-W.
- Quinlan, R. J. 1988. “Use of Fungi to Control Insects in Glasshouses.” In Fungi in Biological Control Systems, edited by M. N. Burges, 19–36. Manchester, UK: Manchester University Press.
- Sain, S. K., D. Monga, R. Kumar, D. T. Nagrale, N. S. Hiremani, and S. Kranthi. 2019. “Compatibility of Entomopathogenic Fungi with Insecticides and Their Efficacy for IPM of Bemisia tabaci in Cotton.” Journal of Pesticide Science 44 (2): 97–105. doi: 10.1584/jpestics.D18-067.
- Todorova, S. I., D. Coderre, R.-M. Duchesne, and J.-C. Côté. 1998. “Compatibility of Beauveria bassiana with Selected Fungicides and Herbicides.” Environmental Entomology 27 (2): 427–433. doi: 10.1093/ee/27.2.427.
- Upadhyay, V., D. Rai, M. Rana, P. Mehra, and A. K. Pandey. 2014. “Verticillium lecani (Zimm.): a Potential Entomopathogenic Fungus.” International Journal of Agriculture, Environment and Biotechnology 7 (4): 719–727. doi: 10.5958/2230-732X.2014.01380.1.
- Verhaar, M. A., T. Hijwegen, and J. C. Zadoks. 1996. “Glasshouse Experiments on Biocontrol of Cucumber Powdery Mildew (Sphaerotheca: Fuliginea) by the Mycoparasites Verticillium lecanii and Sporothrix Rugulosa.” Biological Control 6 (3): 353–360. doi: 10.1006/bcon.1996.0045.
- Wang, L., J. Huang, M. You, and B. Liu. 2004. “Time-Dose-Mortality Modelling and Virulence Indices for Six Strains of Verticillium lecanii against Sweetpotato Whitefly, Bemisia tabaci (Gennadius).” Journal of Applied Entomology 128 (7): 494–500. doi: 10.1111/j.1439-0418.2004.00879.x.