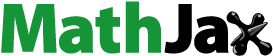
ABSTRACT
The stabilisation of expansive clay subgrades using recycled glass (RG) was proposed, as a sustainable ground improvement technique. Previous studies mainly focused on using RG powder with contents up to 10%, while the current study utilised sand-size particles and up to 40% RG content. Physical properties, compressibility, strength characteristics, and long-term climatic effects on the volumetric behaviour of stabilised clay were investigated. Volumetric responses of stabilised clay were analysed through a constitutive model developed for environmentally stabilised clay. The experimental results revealed that the plasticity of mixtures decreased by 30% as RG content increased to 40%. By using larger RG particles, the strength and bearing capacity increased by about 45% and 130% with the addition of 25% RG. However, adding about 6% of glass powder was sufficient to increase the strength and bearing capacity to about 100% and 200%, respectively. The swell-shrink results suggested that the maximum swelling was achieved in the second cycle in which the clay classification was converted from medium to high expansive clay. The experimental results were also compared and discussed with corresponding data collected from the literature. The outcomes of this study advance the prediction and understanding of the mechanical behaviour of RG-stabilised clay.
Introduction
Expansive soils have been known to be problematic in causing severe distresses to civil infrastructures. These soils are susceptible to moisture content changes causing significant volume changes (Al-Taie et al. Citation2019, Estabragh et al. Citation2021). These volumetric strains may exceed 10%, inducing differential movements resulting in surface cracking and distresses to infrastructures, especially light structures such as pavements of roads (Barman and Dash Citation2022). This has resulted in the loss of billions of dollars in repairs and rehabilitation of civil infrastructures making improvement technics for expansive soil stabilisation a critical topic of interest for geotechnical and pavement engineers and practitioners.
Various soil improvement and stabilisation techniques have been developed including chemical and mechanical stabilisation methods. Over decades, extensive studies investigated the suitability of using chemical additives, in particular lime, cement and fly ash, in enhancement of soil properties. These studies showed that the chemical stabilisation method has achieved significant improvements in increasing soil workability, durability, strength and decreasing soil plasticity (Yaghoubi et al. Citation2013, Abdullah et al. Citation2019, Al-Taie et al. Citation2019). Nevertheless, there are several drawbacks in using chemical techniques including two deleterious chemical reactions, being carbonation and the reaction with the sulphate salt existing in the soil that resulted in soil distress, swelling and degradation which generally cause the loss of strength (Ibtehaj et al. Citation2014). Furthermore, chemical stabilisation is known to have negative environmental impacts, mainly the emission of CO2 during the production of stabilisers such as cement (Indraratna et al. Citation2013). In spite of the abovementioned drawbacks, chemical additives are still widely used in many applications in geotechnical engineering practice, such as subgrade improvements, channel linings, earth dams and embankments, since the advantages they offer, such as reliability, availability and certainty in effectiveness outweigh their disadvantages (Firoozi et al. Citation2017).
Mechanical stabilisation involves compaction (Wang et al. Citation2013), pre-wetting (Nelson et al. Citation2015), wetting-drying cycles (Estabragh et al. Citation2013), reinforcement (Yadav and Tiwari Citation2017), replacement of expansive layer with an inert material (Bharadwaj Citation2013) and the use of solid wastes (Abukhettala and Fall Citation2021). In the mechanical stabilisation process, highly skilled staff and time-consuming laboratory testing programmes are not needed. Furthermore, this method of stabilisation does not pose a threat to the environment; rather, when waste materials are utilised for the mechanical stabilisation, a sustainable waste management technique is implemented automatically as an alternative to the unsustainable landfilling approach (Tahmoorian et al. Citation2022). Cabalar et al. (Citation2014), Cabalar et al. (Citation2016), Cabalar et al. (Citation2017), Cabalar et al. (Citation2018) and Cabalar et al. (Citation2020) stated the suitability of implementing waste materials such as tyre buffings, crushed brick, dragged asphalt, crushed concrete, waste ceramic, biopolymer and steel computer numerical control milling waste spirals as alternative materials to enhance the performance of road pavement subgrades.
The focus of the current study is the stabilisation of expansive clay using recycled/waste materials, in particular recycled glass. The growing waste materials stockpiles and lack of landfill areas highlight the necessity of innovative schemes to find a purpose for using waste and recycled materials. This can decrease the request for construction materials sourced from natural resources, thereby contributing to the sustainability of our natural environment (Senanayake et al. Citation2022).
Glass waste, which is the source of recycled glass (RG), is a solid waste placed at the top of the list in terms of production with a volume of global production of approximately 130 million tons (Ferdous et al. Citation2021). In Australia, about 850 thousand tons of glass are used annually, of which only 40% are regained for recycling, and hence, a significant amount of waste is dumped in landfills. The glass bio-disintegration usually requires about 50 decades which adds to the importance of using recycled glass in potential applications. The properties of the crushed glass including small strain stiffness, high permeability, and high crushing resistance have made it an appealing replacement for natural sands in geotechnical applications such as soil stabilisation, embankments and other construction activities that require sand (Javed and Chakraborty Citation2020). Recycled glass particles are generally angular shaped with some flat particles (Disfani et al. Citation2011, Arulrajah et al. Citation2014, Cabalar and Demir Citation2022)
Numerous researchers have discussed the feasibility of utilising recycled glass in road construction. Arulrajah et al. (Citation2014) and Disfani et al. (Citation2011) investigated using glass mixtures as unbound aggregates used for road base or subbase layers. They reported that the mixtures of recycled glass, recycled concrete and waste excavation rock are qualified to be used in pavement subbase applications but are not fully suitable for pavement base application. Disfani et al. (Citation2017) investigated the mixtures of recycled glass and crumbed rubber and showed that the mixture of rigid particles of glass and soft particles of tire crumbs in their optimum ratio can be a suitable fill material for lightweight highway embankments. Mohajerani et al. (Citation2017) presented an overview of studies carried out to reuse crushed glass as an aggregate in unbound base and subbase applications and the outcome showed the potential of using crushed glass in construction materials. Mohsenian Hadad Amlashi et al. (Citation2020) investigated the feasibility of using recycled glass as an aggregate in pavement structures. The results revealed that limestone aggregates showed less drainage potential compared to recycled glass. Drainage properties play an important role in the performance of the granular base and subbase layers when the interaction of water and the pavement structure is likely. Yaghoubi et al. (Citation2022) and Teodosio et al. (Citation2022) recommended utilising recycled glass in backfilling deep sewer trenches located in non-trafficable areas.
Recently, several studies have investigated the use of recycled glass in improving the geotechnical properties of expansive soils, as summarised in . It was common to see research that incorporated cement binders as a chemical stabilisation. Also, all the studies mentioned in , except for Yaghoubi et al. (Citation2021), applied static experimental testing to recycled glass with particle sizes smaller than 0.4 mm, i.e. glass powder, while particle sizes greater than 0.4 mm were disregarded.
Table 1. Sources studied on the effect of adding glass particles on the subgrade behavior
The recycled glass powder particles have been found to exhibit pozzolanic characteristics (Kalakada et al. Citation2020). The potential for pozzolanic reaction increases as the particle size decreases. Unlike the sand-size RG used in this study, glass powder partially acts by chemical stabilisation of soils rather than an entirely mechanical stabilisation. In the current study, sand size RG was used due to fewer studies on non-powder RG. In Australia, sand size RG is available in large quantities and its cost is 25–50% less than that of natural sand. However, the additional process to produce glass powder results in greater recycling cost and energy in comparison to producing sand size glass (Ogundairo et al. Citation2019).
The compression response of clay–aggregate blends is essentially controlled by the clay or aggregate constituent up to a ‘transition content’ where either the clay or aggregates are dominant (Cabalar et al. Citation2014). That is, the mechanical response of the blend below the transition aggregate content is governed by clay and the behaviour above that content is governed by aggregate particles. At small aggregate contents, aggregate particles are far away to contact and thus the blend behaviour is mainly governed by the clay. When more aggregate particles are added to the clay matrix, extra voids would appear between the clay and aggregate particles during the sample preparation and consequently cause higher compressibility of the blend. However, as the clay dry density is constant, adding further aggregate also results in increasing the compactness of the clay restrained among the aggregate particles during the compaction. Jafari and Shafiee (Citation2004]) reported that the trapped clay particles are compressed under higher stresses and are thus harder than clays located in other zones of the mixture. The harder clay parts act like bridges between adjacent aggregate particles, which are also part of the force chain. Therefore, the force chain is more stable, and the compression index is less than that of pure clay (Yaghoubi et al. Citation2021).
Another area that was not previously researched is the effect of ‘cyclic swell–shrink’, which is one of the methods used for investigating the swell–shrink due to the climatic effect, on the swelling potential for the long term (Tripathy et al. Citation2002, Al-Taie et al. Citation2020). One-dimensional consolidation theory for unsaturated soils was applied to represent the climatic effect on the volumetric behaviour of expansive soils. This required the measurement of swelling and shrinkage potential under controlled suction. Suction is known to produce hydraulic hysteresis with void ratio during wetting and drying cycles (Fleureau et al. Citation1993). However, the moisture content produced a linear correlation with void ratio during cyclic wetting and drying (Fleureau et al. Citation2002) and this was validated by Tripathy et al. (Citation2002) during studying the behaviour of compacted expansive soils under swell-shrink cycles by using moisture content and void ratio. This behaviour was attributed to the fact that both void ratio and moisture ratio showed a hysteresis with suction, which was cancelled out when void ratio and moisture ratio were considered together (Kodikara Citation2012). Therefore, several researchers followed the cyclic wetting and drying procedure proposed by Tripathy et al. (Citation2002) to investigate the climatic effect on volumetric behaviour and consequently on the structural design (Peng et al. Citation2007, Tripathy and Subba Rao Citation2009, Yazdandoust and Yasrobi Citation2010, Estabragh et al. Citation2015, Ikeagwuani and Nwonu Citation2019, Al-Taie et al. Citation2020). For compacted clays, high swelling values are expected in the second cycle of swell-shrink which could be more than double those obtained from the first cycle (Tripathy et al. Citation2002). Thus, it is crucial to consider at least one method of evaluating climatic effects in the investigations to emulate the field conditions for long-term applications.
Although several studies have explored the utilisation of glass for the stabilisation of clay, the previous research mainly focused on powdered glass, glass fine and cement glass powder with small percentage. There is a lack of rigorous research in the literature concerning the stabilisation of expansive clay with coarse crushed glass, readily available in vast quantities in various areas such as Melbourne, Australia, at high contents. Furthermore, to the best of the authors’ knowledge, the climatic effect on the volumetric behaviour of RG-stabilised clay has not been studied. All chemical and mechanical stabilisation methods improve expansive soil properties, however, there are factors that affect the degree of improvement. For mechanical stabilisation using recycled glass, the factors include the size of particles and the content. These factors identify the percentage of glass that should be added to achieve a desired improvement. To date, no comprehensive research interpreted the complete scenario of the effect of the RG size and content on the volumetric and strength behaviours of RG-stabilised expansive clay subgrades. In this study, a comprehensive investigation on the mechanical behaviour of clay soil stabilised with glass at different particle size distributions and contents was carried out. The current study considered the effect of adding high recycled glass contents up to 40% comprising particle sizes up to 2.36 mm on the mechanical properties of expansive clay. Furthermore, the long term performance of the stabilised clay was studied through investigating the climatic effect on the volumetric behaviour of the stabilised clay. Finally, the effect of the glass particle sizes and proportions on physical and mechanical soil properties was determined by comparing the findings obtained from this research with the corresponding values obtained from the literature as presented in . This study promotes the application of recycled materials in the geotechnical and pavement industries through improving the performance of problematic subgrade soils. Thus, recycled glass can be a construction material for soil stabilisation which is economically beneficial and environmentally safe.
Methodology
To investigate the impact of recycled glass (RG) content and size on the mechanical characteristics and swell-shrink behaviour of clay-recycled glass (C-RG) mixtures, first, the physical properties of C-RG mixtures with gravimetric RG contents of 0%, 10%, 20%, 30% and 40% comprising particle sizes up to 2.36 mm were determined. This was carried out through the determination of the specific gravity, grain size analysis, Atterberg limits and compaction tests. Secondly, a series of oedometer tests were conducted on the C-RG mixtures to explore their compressibility and swelling responses. Thirdly, unconfined compressive strength (UCS) and California bearing ratio (CBR) tests were carried out to determine the bearing capacity of C-RG mixtures under static loading. Next, swell-shrink cycles were applied as one of the widely used methods of simulating the effect of climatic wetting-drying cycles. Finally, the outcome of this study was compared with previous studies on the stabilisation of clay using RG and the effect of adding different sizes and contents of RG to clay was investigated.
Materials
Two types of materials were used being a type of expansive clay and recycled glass, as shown in . The expansive clay was collected from a depth of up to 1 m at the western suburbs of Melbourne in Australia. The collected clay was desiccated at the temperature of 50°C for 4 days. The desiccated clay was next crushed and ground using a laboratory-scale crusher and a soil grinder. Specific gravity (Gs) (ASTM-D854 Citation2010), liquid limit (LL), plastic limit (PL) and plasticity index (PI) (ASTM-D4318 Citation2017) were measured to be 2.71, 60%, 24.4% and 35.6%, respectively. With these results, the clay was classified as clay with high plasticity (CH) following ASTM-D2487 (Citation2011). According to ASTM-D7928 (Citation2017), the grain size distribution curve of the clay is depicted in showing that the fine fraction of the soil was about 97.8% (silt and clay) while the sand content was only 2.2%. The optimum moisture content (OMC) and maximum dry density (MDD) based on standard Proctor compaction (ASTM-D698 Citation2012) were 14.6 kN/m3 and 22.3%, respectively.
The RG was provided by local recycling facilities in Victoria, Australia. The specific gravity of the RG was measured according to the ASTM-C127 (Citation2012) procedure to be 2.48. The particle size distribution presented in shows that the maximum particle size (Dmax), coefficient of uniformity (Cu) and coefficient of curvature (Cc) were 2.36 mm, 7.30 and 1.12, respectively. Following the Unified Soil Classification System (USCS) (ASTM-D2487 Citation2011), RG can be classified as well-graded sand (SW).
Four clay-glass mixtures with gravimetric RG contents of 10%, 20%, 30% and 40% were proposed to investigate the impact of glass content on the mechanical behaviour of expansive clay with clay as the benchmark. The mix ratios were selected to cover a wide range of RG contents with 10% RG content intervals. The maximum RG content of 40% was selected since it was presumed that at higher contents, the behaviour of the mixture would be more dominated by sand-size particles rather than clay. After observing the compaction and oedometer test results exhibiting a significant change between 20% and 30% RG contents, for further evaluation, samples with 25% RG contents were also prepared for swell-shrink testing.
Preparation of clay-glass mixtures and testing procedures
Oedometer test
Following the ASTM-D2435/D2435M (Citation2011), the one-dimensional consolidation test was performed to investigate the compressibility characteristics of the mixtures such as compression index, swelling index, initial void ratio and swelling characteristics. The cylindrical samples with a diameter of 50 mm and a height of 20 mm were prepared at the OMC and MDD obtained from the standard Proctor compaction test. The samples were inundated in water and left for swelling under a stress of 6.7 kPa until a non-considerable volume change was recorded. The stress of 6.7 kPa was suggested to classify the expansivity of the clay and clay-glass mixture according to Seed et al. (Citation1962) proposed method. The expansivity of soils refers to the volume change potential as a result of adsorption of water by soil’s minerals. A sequence of stresses was next applied including 12.5, 25, 50, 100, 200, 400, 800, 1600, 400, 100 and 25 kPa (ASTM-D2435/D2435M Citation2011). At the end of testing, void ratio vs log stress was depicted and the influence of glass content on compression index (Cc), swell index (Cs) and the amount of swelling was measured.
Unconfined compressive strength (UCS) test
Following the procedure described in ASTM-D2166 (Citation2016), the UCS tests were conducted on the mixtures with 0%, 10%, 20%, 30% and 40% of RG at their corresponding OMC and MDD. The test was carried out at a rate of strain of 1.0 mm/min to assure the drying of the sample is minimal by completing a test in less than 15 min. The loading continued until the stress declined after reaching a peak value. The axial stress vs axial strain and USC versus RG were next depicted and assessed.
California bearing ratio (CBR) test
Following ASTM-D1883 (Citation2016) procedure, the CBR samples were prepared by applying standard Proctor compaction effort on the C-RG mixtures at their standard compaction OMC. A load of 4.5 kg was set on the top of the samples and the samples were inundated in water to saturate for seven days. This load was to simulate the confining effect of overlying pavement layers (VicRoads Citation1998).
Cyclic swell-shrink and state paths
To study the long-term climatic impact on the volumetric behaviour, and thus, the influence on the structural design for clay and RG-stabilised clay foundations, swell-shrink cycle test (Al-Taie et al. Citation2020), which is one of several proposed testing methods for investigating climatic effects, was conducted. For this, the impact of RG stabilisation was investigated on the swell-shrink path after reaching an equilibrium where the same swelling and shrinkage values were achieved. The C-RG mixtures were prepared at their corresponding OMC and MDD and were subjected to swell-shrink cycles under the stress of 6.7 kPa according to the procedure recommended by Tripathy et al. (Citation2002). The swell-shrink tests were carried out using an oedometer device placed in a room with a controlled temperature to allow the samples to shrink under the stress of 6.7 kPa and the temperature of 40 ± 0.5°C during the shrinkage process. The loading plate was perforated to allow the injection of water into the samples during swelling using a medical syringe as shown in .
Swell-shrink cycles
The target stress of 6.7 kPa was implemented on at least six identical 50 mm by 20 mm samples. The samples were then submerged in water to swell under the room temperature (approximately 25°C). The vertical deformations were measured using a dial gauge with a 0.01 mm accuracy. Once a negligible change in swelling (<0.5%) was detected, the shrinkage process began. First, water around the ring cell was taken out and the temperature was gradually raised to 40 ± 0.5°C for the shrinkage process. The stress was kept at 6.7 kPa and the vertical deformations were recorded to complete the first cycle of swell-shrink. The sample temperature was next reverted to 25°C in approximately 2–3 h. The samples were then submerged in the water again to begin the next swell-shrink cycle. These steps were repeated 5 times until achieving the equilibrium that is by reaching the same deformations during swelling and shrinkage.
Void ratio-moisture content paths
The void ratio changes due to the variation in the moisture content were measured at equilibrium. A medical syringe was used to inject various pre-determined amounts of water into each sample through the holes provided in the loading plate (). The experimental testing was completed after no further deformation was observed. Void ratios were calculated, after the test, by measuring the weight, volume and moisture content of the samples. For the oven-dried samples, the sample’s volume was determined using the kerosene displacement technique. The shrinkage of soil samples inevitably led to a decrease in the sample diameter and was accompanied by shrinkage cracks. By knowing the sample moisture content, the mass and the volume, the void ratio was determined.
The model proposed by Gould et al. (Citation2011) was used to determine the swell-shrink path represented by the void ratio-moisture content relationship of environmentally stabilised clay. The model is expressed in Equations (1) and (2):
(1)
(1)
(2)
(2) where: e is the void ratio; Mc is the moisture content;
,
,
,
, and
are fitting parameters.
The fitting parameters of the model can be explained in idealised void ratio-moisture content paths of the environmentally stabilised clay shown in (Gould et al. Citation2011). Parameters of
dominate the moisture contents where the slope of the swell-shrink path decreases and increases, respectively. The
parameter dictates the change rate of the slope around points
and
. The parameter of
can move the curve vertically. The parameter of
impacts the gradient of the proportional shrinkage zone.
Figure 3. Moisture content vs void ratio at equilibrium according to Gould et al. (Citation2011).

Results and discussions
Compaction characteristics
The impact of RG on the compaction characteristics (OMC and MDD) was investigated by conducting standard Proctor compaction tests on RG-stabilised samples. The variation of OMC and MDD versus RG is presented in , showing that an increase in the RG content from 0% to 30% resulted in an 11% increase and 22.7% decrease in the MDD and OMC, respectively. The same behaviour was noticed for clay specimens stabilised with RG powder at various contents (Ikara et al. Citation2015, Blayi et al. Citation2020, Javed and Chakraborty Citation2020, Cabalar and Demir Citation2022).
Figure 4. Compaction test results: (a) standard compaction curves, (b) effect of RG content on compaction characteristics.

The decrease in the OMC was due to the higher water absorption potential of clay compared to RG particles. Also, adding more RG increased the MDD which consequently decreased the available voids in the sample which could potentially be filled with water. The reduction in the MDD that was observed after adding 40% of RG will be discussed later.
Consistency limits
Consistency limits tests were carried out to determine the effect of RG on the plastic behaviour of stabilised samples. The consistency limit results presented in show that the liquid limit (LL), plasticity index (PI), and Linear shrinkage (LS) values varied from 60% to 46%, 35.4% to 24.5% and from 17.2% to 13%, respectively. Therefore, the maximum reductions achieved in LL, PI and LS were 23%, 31% and 24%, respectively. These reductions were due to adding non-plastic recycled glass which led to the reduction of the clay plasticity. Similar reduction trends were observed in expansive clay specimens stabilised with RG powder at contents ≥ 15% (Başarı Citation2012, Ikara et al. Citation2015, Ibrahim et al. Citation2021). However, the reduction in PI decreased to approximately 20% when less than 15% RG powder was added (Javed and Chakraborty Citation2020). This indicated that the size of particles had a minor effect on consistency limits when the RG was added at high proportions.
It is important to mention that further reductions in the LL, PI and LS were anticipated for all mixtures. However, the proportion of particles finer than 0.425 mm (the maximum particle size used in the consistency limits test) for the recycled glass was approximately 29% (). This indicated that the mixture of, e.g. RG-40 had about 60% clay and 11.6% glass finer than 0.425 mm. As a result, the decrease in consistency limits relied on the proportion of glass particles finer than 0.425 mm.
Oedometer
Effect of recycled glass content on compressibility
shows the compression curves of various samples in terms of global void ratio (e) and vertical stress (σ). The initial global void ratio e0 was determined using Equation (3):
(3)
(3) where Msc and Msg are the clay and RG dry mass, respectively, V is the sample’s total volume (39.3 cm3), ρw is the water density, and Gsc and Gsg are the specific gravities of the clay and RG, respectively. As observed in (a), all the compression curves are nearly linear under high stress levels and the gradient of the line is known as the compression index Cc ( = Δe/Δlogσ). The initial void ratio values decreased by 7%, 16%, 30% and 32% after adding 10%, 20%, 30% and 40% RG, respectively. In the clay-aggregate mixture, a smaller global void ratio generally displays a denser state and lower potential for compressibility which is confirmed in (b). (b) also shows that the effect of glass in the reduction of void ratio and compressibility became negligible after adding 30% RG and no further decrease was noticed in compressibility after adding 40%. The change in global void ratios of the mixtures with 30% and 40% was only 2%, therefore, it was expected that no significant change in the behaviour would be noticed after adding more RG contents. The same characteristics as Cc was also observed for swell index Cs as illustrated in (b). The swell index represents the slope of unloading path in (a) (Cs = Δe/Δlogσ).
Effect of recycled glass content on swelling
One-dimensional swell test results presented in show a 3.8% swell for clay samples. According to the Seed et al. (Citation1962) classification shown in , the clay was categorised as a medium-expansive soil. Adding 25% RG reduced the swelling potential up to about 80% to convert the categorisation from a medium to a low expansive soil (). This occurred because of the addition of non-plastic material to the expansive soil working as an inert material, and hence the reduction of the swelling potential. However, adding the same RG content but in form of RG powders contributed to a 50% decrease in swelling (Blayi et al. Citation2020). This was due to the fact that large particles did not only decrease the plasticity of the mixture but also had a role in confining finer particles and avoiding further swelling. In the current study, the swelling test results recommended the 25% RG content as the optimum following the approach explained in Al-Taie et al. (Citation2021).
Table 2. Classification of reactive soils.
Unconfined compressive strength
shows that growing the RG content from 0% to 20% resulted in a 45% increase in the UCS value, and decreased after adding 30% and 40% RG. However, the results achieved by Javed and Chakraborty (Citation2020), Blayi et al. (Citation2020) and (Babatunde et al. Citation2019) showed that the UCS increased by 75% to 100% when RG powder was added in contents less than 10%. The increase in the UCS followed the clay-aggregate mechanism previously discussed. As RG increased, the compactness of the trapped clay fraction between aggregate particles increased and trapped clay lumps acted as bridges between the aggregate particles and consequently the strength increased. In contrast, replacing compacted clay particles with aggregate would decrease the cohesion between the particles. Technically, the angle of friction increases as aggregate content increases, however, the effect of the loss of cohesion was more prominent as the sample could not stick together without the confinement. As the unconfined compressive tests were carried out under confining pressures equal to zero, the value of UCS decreased after adding 30% and 40% RG. The curves presented in (a) showed a more visible peak as RG content increased and this is an indication of the C-RG mixtures becoming more brittle as the RG content increased.
California bearing ratio
With the addition of recycled glass up to 30%, a steady increase was noticed in the soaked CBR values from 3 to 7 (130% increase) as demonstrated in . However, the CBR values decreased at 40% RG. A similar pattern was observed when clay specimens were stabilised with RG powder, however, the CBR values increased by 100%−300% with the addition of 5–10% RG (Benny et al. Citation2017, Babatunde et al. Citation2019, Blayi et al. Citation2020). The reduction in CBR may be due to the excess RG particles which were not mobilised during compaction and replaced the fine particles of clay with aggregate particles, thereby a reduced density and decreased bond between clay-RG particles.
Cyclic swell-shrink
Based on swelling test findings, the optimum RG content was found to be 25%, therefore the RG-0, RG-10, RG-20 and RG-25 mixtures were chosen to be tested under cycles of swell-shrink.
During the drying process, it was observed that shrinkage induced visible cracks in the samples and a reduction in the sample diameter. The length and width of visible cracks and the reduction in a sample diameter increase as soil expansivity increases. As this study selected a soil with a ‘Medium’ expansive classification, it was expected to detect short and narrow visible cracks and the effect of the soil expansivity appeared in the reduction of soil sample diameter. The visible cracks and reduction in diameter decreased as the RG content increased, as shown in . This is an evidence of the contribution of RG to decreasing the plasticity and swelling/shrinkage potential of the expansive clay. In , the deformations are transformed into strains and the paths of vertical strain for the clay and RG-stabilised samples under a stress of 6.7 kPa are demonstrated. At the end of the fifth cycle, the vertical strains for the clay and glass-stabilised samples with 10%, 20% and 25% glass contents were 7.80%, 3.30%, 1.83 and 0.8%, respectively. Using the plot of , the variations in swelling and shrinkage with swell-shrink cycles were determined and demonstrated in .
Figure 10. States of RG-stabilized clay after four cycles of shrinkage (a) RG-0 (b) RG-10 (c) RG-20 and (d) RG-25.

As an example, the swelling values of A, B and C in were measured as the variation of strains between points a and a*, b and b*, c and c* in , respectively. The values of shrinkage of D, E and F in were measured as the variation of the strain between points a* and b, b* and c, c* and d in , respectively.
and suggest that the maximum swelling was reached at the end of the second cycle and the swelling values after equilibrium for RG-0, RG-10, RG-20 and RG-25 were 2, 1.3, 1.1 and 1 times those obtained at the end of the first cycle. This shows that the risk of achieving a significant increase in swelling at the end of the second cycle decreased as RG content increased. Based on the abovementioned observations, if a pavement is designed based on the swelling potential obtained from the first cycle, the structure will experience higher deformations in the second cycle. The swell achieved at the end of the second cycle was sufficient to convert the clay classification from medium to high expansive soil. However, by increasing the RG content up to the optimum, the swelling obtained from the first cycle is sufficient for considering the subgrade as the low-expansive type for pavement design.
Void ratio-moisture content state paths
At the equilibrium, the variation in the void ratio with the moisture content changes was determined for the clay and RG-stabilised samples under the stress of 6.7 kPa. Using Equations (1) and (2) suggested by Gould et al. (Citation2011), the relationships between the void ratio and the moisture content were depicted (). Each point in represents an individual sample. shows that the clay sample reached the maximum swelling at about 37% moisture content. When the same sample was desiccated to the moisture content of 13%, the maximum shrinkage was achieved. The RG-stabilised samples with 10%, 20% and 25% RG contents reached the maximum swelling at about 33%, 30% and 29% moisture content, respectively. However, the same samples experienced maximum shrinkage when they were desiccated to a moisture content of 10%.
For the clay sample, the gradient of the swell-shrink path (φ) defined in Equation (4) was found to be 0.98 and this gradient was reduced to 0.58, 0.34 and 0.12 after stabilisation with 10%, 20% and 25%, respectively. The gradient of the swell-shrink path is M (Equation 5), as shown in .
(4)
(4)
(5)
(5)
(6)
(6) where ew is the moisture ratio. The major volume change shown in occurred through the linear part of the S-shaped curve. For the clay sample, the main change in volume started when the sample reached about 60% saturation and the change in volume became minimal at 85% saturation. However, for the stabilised samples, the main change in volume began once the sample achieved about 40% saturation, however, no significant change in volume was observed in the degree of saturation greater than 90%. This was due to the changes in the pore structure of the clay after stabilisation with glass. The swelling of the clay sample is greater than that of the RG stabilised samples, which is an indication of the clay sample void ratio being greater than that of the stabilised samples, as previously shown in form of dry density in . The void ratio-degree of saturation relationship is a reverse relationship at constant moisture contents. Hence, adding a particular amount of water to the clay and RG-stabilised samples resulted in achieving a degree of saturation for a RG-stabilised sample higher than that of the a clay sample.
Comparison of the current study findings with the literature
Experimental data from the literature shown in were collected to investigate the effect of glass particle size and content on clay properties including consistency limits, compaction characteristics, UCS and CBR values. For an improved understanding, the effect of adding the RG on the clay soil properties was investigated by calculating the ratio of the stabilised soil property to the corresponding value for unstabilised soil. For instance, the MDD of the selected clay used in the current study was 14.6 kN/m3. After adding 10%, 20%, 30% and 40% RG, the MDDs were determined and found to be 14.9, 15.4, 16.2 and 16 kN/m3, respectively. That means the MDD of the clay soil increased by 1.02 (i.e. 14.9/14.6), 1.05, 1.11 and 1.10 after increasing the RG content to 10%, 20, 30% and 40%, respectively.
Compaction characteristics
presents a significant decrease in the OMC and an increase in the MDD after adding up to 30% RG. It is also observed that beyond 30% of RG, the effect of adding RG on OMC and MDD was less significant. This is due to the fact that increasing the RG content produces an increase in the compactness of the clay and the extra voids created by compacting clay are filled with the added glass and hence, for a constant volume, the mass of the mixture increases and the density of mixture increases. When the compactness of the clay reaches the maximum, the additional RG particles replace the compacted clay particles and as the specific gravity of RG is less than the clay, the density decreases.
The general trends of the OMC and MDD ratios with increasing the RG content are demonstrated in Equations (6) and (7). Hence, regardless of the RG particle size, the OMC and MDD ratios of a particular expansive clay after adding various RG content can be predicted.
(6)
(6)
(7)
(7)
It should be noted that the equations above are only applicable to mixtures of expansive clay and recycled glass.
Consistency limits
The LL and PI values versus RG content were plotted as presented in . From this figure, it was noticed that the values of LL and PI were scattered for all samples stabilised with RG contents less than 10% and then the trend became uniform at greater RG contents. (b) also shows that in the current study, the larger size RG particles have less effect on the PI as only particles passing sieve No. 40 (0.425 mm) are considered in this testing methodology.
The LL and PI ratios at various RG contents can be predicted using Equations (8)–(10):
(8)
(8)
(9)
(9)
(10)
(10)
UCS and CBR
and show that the maximum change in the UCS and CBR increased as the RG content and the particle size decreased. The study of Javed and Chakraborty (Citation2020) used glass with Dmax = 0.075 mm and the maximum UCS and CBR ratios achieved were 2.1, and 6.7 after adding 8% RG. However, the current study used the RG with Dmax = 2.36 mm and the maximum UCS and CBR ratios were 1.5 and 2.3 after adding 20% and 30% RG, respectively. By comparing Benny et al. (Citation2017) study with Javed and Chakraborty (Citation2020) and the current study, it was found that although Benny et al. (Citation2017) used RG with Dmax = 0.075 mm, the UCS and CBR ratios were found to be 1.2 and 2. These values were lower than the corresponding values obtained from the current study that used the RG with Dmax = 2.36. This outcome negates the trend shown at the first sight in that UCS and CBR increased as the RG content decreased. In fact, the maximum ratios in UCS and CBR depend on the MDD of the clay and the UCS and CBR ratios increased as the MDDs increased. showed that the lowest and highest MDDs achieved by Benny et al. (Citation2017) and Javed and Chakraborty (Citation2020) were 14.6 and 18.3 kN/m3, respectively. Interestingly, the minimum and maximum UCS and CBR ratios were also respectively observed through these studies. It should be noted that both the current study and Benny et al. (Citation2017) used clay with MDD equivalent to 14.6 kN/m3. However, the UCS and CBR ratios were higher than the corresponding values measured by Benny et al. (Citation2017). This is due to the MDD at the optimum RG content obtained in the current study (16.2 kN/m3) was higher than that of Benny et al. (Citation2017) study (14.9 kN/m3). This is an indicator of the contribution of the larger size particles to the increase in the density of the RG stabilised clay.
and also showed that the optimum RG content increased as the Dmax increased. It is well known that the UCS and CBR values increased as the glass particle contacts increased. For two C-RG mixtures with two RG particle sizes (fine and coarse) but with the same RG content, the fine particles have a surface area higher than that of the coarse particle and consequently more particle contacts. Therefore, a lower RG content is sufficient to achieve maximum UCS and CBR values. However, with coarse RG particles, a greater RG content is required to achieve the same particle contacts as with fine RG particles. Also, as the unconfined compressive strength of coarse particles is higher than that of the fine particles (Hoang et al. Citation2020, Simatupang et al. Citation2020), the stabilised soil reached the maximum UCS and CBR with values higher than that of the fine particles.
Conclusion
This study investigated the effects of recycled glass (RG) content and particle size on the mechanical and shrink-swell behaviour of clay-recycled glass mixtures. In the current study, a type of RG with particle sizes up to 2.36 mm was mixed with expansive clay at 10–40% RG contents. A series of oedometer, unconfined compressive strength and CBR tests were carried out on clay-glass mixtures. In addition, the climatic effect on the volumetric behaviour of the mixtures was investigated by performing the swell-shrink cycle test and analysing the test results through a well-established constitutive model developed for the volume change behaviour of clays. The results of the current study were compared with the literature and the impact of adding RG at various contents and sizes was investigated. The main findings were as follows:
A 30% decrease in the plasticity of clay was observed with the addition of 40% RG. The effect of RG on PI decreased as particle size increased.
The OMC and MDD decreased and increased, respectively, as the RG content increased until the RG content reached the threshold content. The threshold was found to be approximately 25%.
The prediction of LL, PI, OMC and MDD ratios with glass content was proposed.
The maximum UCS and CBR ratios depended on the MDD of the clay and these ratios increased as the MDD of the clay increased.
About 45% and 130% increase in UCS and CBR values when 25% of larger RG particles were added. However, adding about 6% of glass powder was sufficient to increase the UCS and CBR to about 100% and 200%, respectively.
The swell-shrink cycle results for the clay revealed that the maximum swelling was achieved at the end of the second cycle, while transforming the clay categorisation from a medium to a high expansive clay. This finding suggested reviewing the current pavement design methods as presently, the climate effect may be underestimated.
At the optimum RG content of 25%, the swelling obtained from the first cycle could be taken for the pavement design considerations without the need for the second cycle.
The findings of the current study aimed to promote the application of recycled glass in the stabilisation of problematic subgrades as an alternative to conventional stabilisation methods. The future areas of work in this regard can be the construction of a trial site for performance monitoring, as well as investigating the mechanical behaviour of the clay-recycled glass mixtures under repeated loading conditions.
Data availability statement
All data, models, and code generated or used during the study appear in the published article.
Disclosure statement
No potential conflict of interest was reported by the author(s).
Additional information
Funding
References
- Abdullah, H.H., Shahin, M.A., and Sarker, P., 2019. Use of fly-ash geopolymer incorporating ground granulated slag for stabilisation of kaolin clay cured at ambient temperature. Geotechnical and Geological Engineering, 37 (2), 721–740.
- Abukhettala, M., and Fall, M., 2021. Geotechnical characterization of plastic waste materials in pavement subgrade applications. Transportation Geotechnics, 27, 100472.
- Al-Taie, A., et al., 2019. Collapse and swell of lime stabilized expansive clays in void ratio–moisture ratio–net stress space. International Journal of Geomechanics, 19 (9), 04019105. doi:10.1061/(ASCE)GM.1943-5622.0001488. Available from: https://browzine.com/articles/326402102.
- Al-Taie, A., et al., 2020. Effect of swell–shrink cycles on volumetric behavior of compacted expansive clay stabilized using lime. International Journal of Geomechanics, 20 (11), 04020212.
- Al-Taie, A., Disfani, M., and Yaghoubi, E., 2021. Suitability of swelling and collapse theory proposed based on virgin compression surface. Soils and Foundations, 61 (1), 113–128. Available from: http://www.sciencedirect.com/science/article/pii/S0038080620337562.
- Arrieta Baldovino, J.D.J., et al., 2020. Sustainable use of recycled-glass powder in soil stabilization. Journal of Materials in Civil Engineering, 32 (5), 04020080.
- Arulrajah, A., et al., 2014. Recycled-glass blends in pavement base/subbase applications: laboratory and field evaluation. Journal of Materials in Civil Engineering, 26 (7), 04014025.
- ASTM-C127, 2012. Standard test method for density, relative density (specific gravity), and absorption of coarse aggregate. West Conshohocken: ASTM International.
- ASTM-D1883, 2016. Standard test method for California bearing ratio (cbr) of laboratory-compacted soils. West Conshohocken: ASTM International.
- ASTM-D2166, 2016. Standard test method for unconfined compressive strength of cohesive soil. West Conshohocken, PA: ASTM International.
- ASTM-D2435/D2435 m, 2011. Standard test method for one-dimensional consolidation properties of soils using incremental loading. West Conshohocken: ASTM International.
- ASTM-D2487, 2011. Standard practice for classification of soils for engineering purposes (unified soil classification system). West Conshohocken: ASTM International.
- ASTM-D4318, 2017. Standard test method for liquid limit, plastic limit, and plasticity index of soils. West Conshohocken: ASTM International.
- ASTM-D698, 2012. Standard test methods for laboratory compaction characteristics of soil using standard effort (12 400 ft-lbf/ft3 (600 kn-m/m3)). West Conshohocken: ASTM International.
- ASTM-D7928, 2017. Standard test method for particle-size distribution (gradation) of fine-grained soils using the sedimentation (hydrometer) analysis. West Conshohocken: ASTM International.
- ASTM-D854, 2010. Standard test methods for specific gravity of soil solids by water pycnometer. West Conshohocken: ASTM International.
- Attom, M., 2018. The use of waste glass material to control soil swelling pressure. International conference on technological challenges for better world. Philippines.
- Babatunde, O., Sani, J., and Sambo, A., 2019. Black cotton soil stabilization using glass powder. International Journal of Innovative Research in Science, Engineering and Technology, 8 (5), 5208–5214.
- Barman, D., and Dash, S.K., 2022. Stabilization of expansive soils using chemical additives: A review. Journal of Rock Mechanics Geotechnical Engineering, 14 (4), 1319–1342.
- Başarı, E., 2012. Effect of granular waste glass on soil propertiesed.^eds. 3rd international conference on new developments in soil mechanics and geotechnical engineering, proceedings bookNear East University, Nicosia, North Cyprus, 479-484.
- Benny, J.R., et al., 2017. Effect of glass powder on engineering properties of clayey soil. International Journal of Engineering Research and Technology, 6 (5), 228–231.
- Bharadwaj, A., 2013. Effect of soil replacement option on surface deflections for expansive clay profiles: PhD thesis, Arizona, USA: Arizona State University.
- Blayi, R.A., et al., 2020. Strength improvement of expansive soil by utilizing waste glass powder. Case Studies in Construction Materials, 13, e00427.
- Cabalar, A., Abdulnafaa, M., and Karabash, Z., 2016. Influences of various construction and demolition materials on the behavior of a clay. Environmental Earth Sciences, 75 (9), 1–9.
- Cabalar, A.F., Awraheem, M.H., and Khalaf, M.M., 2018. Geotechnical properties of a low-plasticity clay with biopolymer. Journal of Materials in Civil Engineering, 30 (8), 04018170.
- Cabalar, A.F., and Demir, S., 2022. Geotechnical properties of a bentonite treated with waste glass grains. Arabian Journal of Geosciences, 15 (9), 1–9.
- Cabalar, A., Hassan, D., and Abdulnafaa, M., 2017. Use of waste ceramic tiles for road pavement subgrade. Road Materials and Pavement Design, 18 (4), 882–896.
- Cabalar, A.F., Ismael, I.A., and Yavuz, A., 2020. Use of zinc coated steel cnc milling waste for road pavement subgrade. Transportation Geotechnics, 23, 100342.
- Cabalar, A.F., Karabash, Z., and Mustafa, W.S., 2014. Stabilising a clay using tyre buffings and lime. Road Materials and Pavement Design, 15 (4), 872–891.
- Disfani, M., et al., 2011. Recycled crushed glass in road work applications. Waste Management, 31 (11), 2341–2351.
- Disfani, M.M., et al., 2017. Shear and compression characteristics of recycled glass-tire mixtures. Journal of Materials in Civil Engineering, 29 (6), 06017003.
- Estabragh, A., et al., 2013. Stabilised expansive soil behaviour during wetting and drying. International Journal of Pavement Engineering, 14 (4), 418–427.
- Estabragh, A., Babaei, M., and Javadi, A., 2021. Effect of glycerol on the behaviour of an expansive soil during wetting and drying cycles. International Journal of Pavement Engineering, 22 (10), 1284–1294.
- Estabragh, A., Parsaei, B., and Javadi, A., 2015. Laboratory investigation of the effect of cyclic wetting and drying on the behaviour of an expansive soil. Soils and Foundations, 55 (2), 304–314.
- Fauzi, A., Djauhari, Z., and Fauzi, U.J., 2016. Soil engineering properties improvement by utilization of cut waste plastic and crushed waste glass as additive. International Journal of Engineering and Technology, 8 (1), 15.
- Ferdous, W., et al., 2021. Recycling of landfill wastes (tyres, plastics and glass) in construction–a review on global waste generation, performance, application and future opportunities. Resources, Conservation and Recycling, 173, 105745.
- Firoozi, A.A., et al., 2017. Fundamentals of soil stabilization. International Journal of Geo-Engineering, 8, 1–16.
- Fleureau, J.-M., et al., 1993. Behavior of clayey soils on drying-wetting paths. Canadian Geotechnical Journal, 30 (2), 287–296.
- Fleureau, J.-M., et al., 2002. Aspects of the behaviour of compacted clayey soils on drying and wetting paths. Canadian Geotechnical Journal, 39 (6), 1341–1357. Available from: http://doi.org/10.1139/t02-100 [Accessed 1 September 2014].
- Gould, S.J.F., et al., 2011. A void ratio - water content - net stress model for environmentally stabilized expansive soils. Canadian Geotechnical Journal, 48 (6), 867–877. Available from: http://ezproxy.lib.swin.edu.au/login?url = http://search.ebscohost.com/login.aspx?direct = true&db =iih&AN = 65536729&site = ehost-live&scope = site.
- Hoang, T., et al., 2020. Engineering properties of biocementation coarse-and fine-grained sand catalyzed by bacterial cells and bacterial enzyme. Journal of Materials in Civil Engineering, 32 (4), 04020030.
- Ibrahim, H.H., Mawlood, Y.I., and Alshkane, Y.M., 2021. Using waste glass powder for stabilizing high-plasticity clay in erbil city-Iraq. International Journal of Geotechnical Engineering, 15 (4), 496–503.
- Ibtehaj, T.J., et al., 2014. Soil stabilization using lime: advantages, disadvantages and proposing a potential alternative. Research Journal of Applied Sciences, Engineering and Technology, 8 (4), 510–520.
- Ikara, I., Kundiri, A., and Mohammed, A., 2015. Effects of waste glass (wg) on the strength characteristics of cement stabilized expansive soil. American Journal of Engineering Research, 4 (11), 33–41.
- Ikeagwuani, C.C., and Nwonu, D.C., 2019. Emerging trends in expansive soil stabilisation: a review. Journal of Rock Mechanics and Geotechnical Engineering, 11 (2), 423–440.
- Indraratna, B., Athukorala, R., and Vinod, J., 2013. Estimating the rate of erosion of a silty sand treated with lignosulfonate. Journal of Geotechnical and Geoenvironmental Engineering, 139 (5), 701–714.
- Jafari, M. and Shafiee, A., 2004. Mechanical behavior of compacted composite clays. Canadian Geotechnical Journal, 41 (6), 1152–1167.
- Javed, S.A., and Chakraborty, S., 2020. Effects of waste glass powder on subgrade soil improvement. World Scientific News, 144, 30–42.
- Kalakada, Z., et al., 2020. Utilisation of coarse glass powder as pozzolanic cement—a mix design investigation. Construction and Building Materials, 240, 117916.
- Kodikara, J., 2012. New framework for volumetric constitutive behaviour of compacted unsaturated soils. Canadian Geotechnical Journal, 49 (11), 1227–1243. doi:10.1139/t2012-084. Available from: http://ezproxy.lib.swin.edu.au/login?url = http://search.ebscohost.com/login.aspx?direct = true&db = iih&AN = 87623281&site = ehost-live&scope = site.
- Mohajerani, A., et al., 2017. Practical recycling applications of crushed waste glass in construction materials: A review. Construction and Building Materials, 156, 443–467.
- Mohsenian Hadad Amlashi, S., et al., 2020. Physical and hydraulic properties of recycled glass as granular materials for pavement structure. Canadian Journal of Civil Engineering, 47 (7), 865–874.
- Nelson, J.D., et al., 2015. Soil treatment and moisture control: foundation engineering for expansive soils. Hoboken, NJ: John Wiley & Sons.
- Ogundairo, T., et al., 2019. Sustainable use of recycled waste glass as an alternative material for building construction–a reviewed.^eds. IOP conference series: materials science and engineeringIOP Publishing, 012073.
- Peng, X., Horn, R., and Smucker, A., 2007. Pore shrinkage dependency of inorganic and organic soils on wetting and drying cycles. Soil Science Society of America Journal, 71 (4), 1095–1104.
- Seed, H.B., Woodward, R.J., and Lundgren, R., 1962. Prediction of swelling potential for compacted clays. Journal of the Soil Mechanics and Foundation Division, ASCE, 88 (SM3), 53–87.
- Senanayake, M., et al., 2022. Evaluation of rutting resistance and geotechnical properties of cement stabilized recycled glass, brick and concrete triple blends. Transportation Geotechnics, 34, 100755.
- Simatupang, M., et al., 2020. The mechanical properties of fly-ash-stabilized sands. Geosciences, 10 (4), 132.
- Sohail, S., and Honna, M., 2018. Comparative study on soil stabilization using powdered glass and sodium hydro-oxide additives. International Journal for Innovative Research in Science & Technology, 4 (12), 86–93.
- Tahmoorian, F., et al., 2022. Evaluation of volumetric performance of asphalt mixtures containing recycled construction aggregate (rca). International Journal of Pavement Engineering, 23 (7), 2191–2205.
- Teodosio, B., et al., 2022. Satellite imaging techniques for ground movement monitoring of a deep pipeline trench backfilled with recycled materials. Remote Sensing, 15 (1), 204.
- Tripathy, S., and Subba Rao, K.S., 2009. Cyclic swell–shrink behaviour of a compacted expansive soil. Geotechnical and Geological Engineering, 27 (1), 89–103.
- Tripathy, S., Subba Rao, K.S., and Fredlund, D.G., 2002. Water content - void ratio swell-shrink paths of compacted expansive soils. Canadian Geotechnical Journal, 39 (4), 938–959. Available from: http://www.scopus.com/inward/record.url?eid = 2-s2.0-0036025550&partnerID = 40&md5 = 7ed010b5373efc52515b6573d314c2cb.
- Vicroads, 1998. Guide to general requirements for unbound pavement materials. Victoria, Australia.
- Wang, Q., et al., 2013. The effects of technological voids on the hydro-mechanical behaviour of compacted bentonite–sand mixture. Soils and Foundations, 53 (2), 232–245.
- Yadav, J., and Tiwari, S., 2017. Effect of waste rubber fibres on the geotechnical properties of clay stabilized with cement. Applied Clay Science, 149, 97–110.
- Yaghoubi, E., et al., 2021. Improving expansive clay subgrades using recycled glass: resilient modulus characteristics and pavement performance. Construction and Building Materials, 302, 124384.
- Yaghoubi, E., et al., 2022. Recycled aggregate mixtures for backfilling sewer trenches in nontrafficable areas. International Journal of Geomechanics, 22 (3), 04021308.
- Yaghoubi, E., Azadegan, O., and Li, J., 2013. Effect of surface layer thickness on the performance of lime and cement treated aggregate surfaced roads. Electronic Journal of Geotechnical Engineering, 18, 1081–1094.
- Yazdandoust, F., and Yasrobi, S.S., 2010. Effect of cyclic wetting and drying on swelling behavior of polymer-stabilized expansive clays. Applied Clay Science, 50 (4), 461–468.