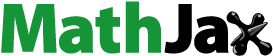
ABSTRACT
Depuration is a proven pre-harvest procedure that eliminates off-flavor from Atlantic salmon produced in recirculating aquaculture systems. However, applied research indicates that depurating salmon lose weight while fasting, which reduces farmgate revenue. Low-ration feeding was therefore evaluated as a method to mitigate weight loss while depurating 3–4 kg Atlantic salmon. Salmon implanted with passive-integrated-transponders were weighed, exposed to concentrated geosmin (GSM) and 2-methylisoborneol (MIB), and stocked in eight pre-disinfected partial reuse systems. Feed was either offered for 4 days or withheld entirely (n = 4) during a 6-day depuration period. Salmon from both treatments rapidly eliminated GSM and MIB, but slightly lower levels remained in fish that were fed (P < .05). Fed and fasted salmon lost 0.3 and 1.1% of their initial body weight, respectively (P < .05). Under the conditions of this study, feeding Atlantic salmon during depuration minimized weight loss without negatively impacting off-flavor elimination.
Introduction
Atlantic salmon (Salmo salar) and other finfish species produced in recirculating aquaculture systems (RAS) can bioaccumulate earthy and musty taints associated with the off-flavor compounds, geosmin (GSM) and 2-methylisoborneol (MIB), respectfully (Azaria and van Rijn Citation2018; Lindholm-Lehto and Vielma Citation2019). Geosmin and MIB are lipophilic compounds; therefore, fatty fish like Atlantic salmon are prone to off-flavor accumulation. However, the extent of off-flavor tainting depends on a variety of factors including ambient off-flavor concentrations, fish size, and water temperature (Howgate Citation2004). Fillets with GSM and MIB levels within the detectable range of the human palate are typically offensive to consumers and can lead to rejected product, negative perception, and lost revenue (Engle, Pounds, and van der Ploeg Citation1995; Tucker Citation2000). Therefore, implementation of effective off-flavor remediation protocols is necessary. At present, the only method that consistently eliminates off-flavor from RAS-produced fish is a finishing procedure known as depuration (Azaria and van Rijn Citation2018). To begin this process, fish are moved from RAS to flow-through or partial reuse systems where water containing negligible GSM and MIB is rapidly exchanged while withholding feed (Burr et al. Citation2012; Davidson et al. Citation2014, Citation2020). Fish then passively eliminate these compounds across their gills along a concentration gradient with the ambient water resulting in reduced concentrations that are no longer objectionable to consumers (Howgate Citation2004).
Depuration effectively reduces GSM and MIB-related off-flavors in RAS-produced Atlantic salmon and other fish species when proven methods are applied. However, there are drawbacks to this procedure including substantial water use (Davidson et al. Citation2020), capital expense for additional system infrastructure, increased labor and fish handling, and reduced fillet lipid levels when prolonged feed withholding is required (Burr et al. Citation2012). Notable fish weight loss can also occur during extended depuration periods. For example, Burr et al. (Citation2012) found that 2.4 kg Atlantic salmon lost 3.8% of their initial body weight after 5 days of purging and 5.8% after 20 days. The same study reported a significant drop in fillet lipid content from 8.2 to 5.1% after 20 days of feed withdrawal. These effects would result in reduced revenue for RAS facilities that sell whole salmon on a dollar per kg basis to customers who expect the health benefits provided by fillet lipids, including omega-3 fatty acids – eicosapentaenoic (EPA) and docosahexaenoic (DHA) (Swanson, Block, and Mousa Citation2012).
Therefore, methods that reduce salmon weight loss during depuration, such as shortening the purging duration through increased water flushing (Davidson et al. Citation2020) or via application of advanced oxidation technologies (Kropp et al. Citation2022) could have economic benefits for RAS operations. Additionally, feeding salmon during the depuration process could be a simple solution to prevent pre-harvest weight loss. However, food is typically withheld from fish undergoing depuration to eliminate waste from the digestive tract for sanitary processing (Borderías and Sánchez-Alonso Citation2011) and to reduce fish metabolism before slaughter (Hvas, Stien, and Oppedal Citation2020; Robb Citation2008). Fasting also minimizes waste excretion in finishing systems (Davidson et al. Citation2023) which could promote the growth of off-flavor-producing bacteria (i.e., actinomycetes) or enhance the production of GSM and MIB by these microorganisms (Blevins, Schrader, and Saadoun Citation1995; Sarker et al. Citation2014; Schrader Citation2020). Nevertheless, positive effects of feeding fish during the depuration process have been reported. For example, Schram et al. (Citation2021) found that feeding Nile tilapia (Oreochromis niloticus) during the depuration process led to slightly faster, albeit non-significant, GSM removal from the flesh of this species. However, the effects of feeding finfish species with substantially greater lipids, i.e., Atlantic salmon, during depuration had not been assessed, and comprehensive effects including weight loss, common off-flavor remediation, product quality, and economic feasibility had not been reported.
Accordingly, two experiments were carried out including i) a pilot study designed to characterize Atlantic salmon weight loss when applying in-house depuration procedures (Davidson et al. Citation2014, Citation2020), and ii) a depuration study comparing low-ration feeding versus the typical feed withholding approach to determine if feeding could reduce pre-harvest weight loss without negatively impacting off-flavor remediation. Atlantic salmon weight loss, GSM and MIB concentrations, fish processing yields, fillet proximate composition, and economics were assessed during the second study to understand the feasibility of feeding Atlantic salmon during the depuration process.
Material and methods
Study 1 – preliminary weight loss assessment
Mixed-sex diploid Atlantic salmon (Riverence, Rochester, WA) with a mean weight of 7.56 ± 0.32 kg, which is within the reported market-size range for farmed Atlantic salmon (NASDAQ Citation2022), were removed from a semi-commercial-scale RAS after one day of feed withdrawal. Upon removal from the RAS, each fish was anesthetized with 75 mg/L Tricaine Methanesulfonate (MS-222) (Popovic et al. Citation2012), weighed on a calibrated scale, and an intracelomic passive integrated transponder (PIT) (Biomark, Boise, ID) was inserted for future identification. These salmon were immediately stocked in two 5 m3 depuration tanks within separate, 13.6 o C partial reuse systems (). Seventeen fish were stocked in tank 1 and 45 fish were stocked in tank 2. In addition, 15 salmon were humanely euthanized, exsanguinated, and processed to capture the following baseline data: i) initial unprocessed weight, ii) weight after gill exsanguination, iii) visceral weight, and iv) head-on-gutted (HOG) carcass weight. Tagging, collection of initial fish weights, and baseline processing were conducted on day 0. Fifteen fish from tank 2 were removed and processed similarly on days 6, 10, and 14, respectively. The collected data were used to calculate processing yields and compartmented loss metrics to understand where weight loss was occurring in the fish body. Fillet color was also determined using a SalmoFan™ Lineal (DSM, Heerlen, Netherlands). Each PIT tagged salmon stocked in tank 1 was weighed on days 6, 10, and 14, and PIT tag data was analyzed to track weight loss over time. These fish were returned to the depuration tank after each sampling event. Experience gained during this study resulted in improved data collection procedures for a second experiment.
Study 2 – feeding vs. fasting
Atlantic salmon
Two groups of Atlantic salmon were used, including an all-female diploid strain (Stofnfiskur, Hafnarfjorður, Iceland) raised to 3–4 kg in six replicated RAS, and a mixed-sex population (Riverence, Rochester, WA) grown to 6–7 kg in partial reuse systems. Each group of fish was moved out of their respective production systems to separate 18 m3 holding tanks, which would later be used for off-flavor dosing. In the interim, these fish were fed to satiation over 48 equally spaced feeding events, using a fishmeal-based salmon diet with 42% protein and 26% fat (Cargill Inc., Minneapolis, MN). Two weeks after stocking, the 3–4 kg salmon were sedated with MS-222, and PIT tags were inserted. Large, 6–7 kg Atlantic salmon were purposed as filler fish to increase biomass densities in the study tanks; therefore, these fish were not tagged. After another 2-week recovery period (a total of 4 weeks in the holding tanks), the salmon were taken off feed for 2 days, then a baseline weight assessment was carried out. The next day, both groups of fish were exposed to concentrated GSM and MIB for 3 h (Sigma Aldrich, Burlington, MA) to increase concentrations of these compounds in fish flesh (Davidson et al. Citation2020, Citation2021). Immediately after, 280 PIT-tagged salmon and 192 untagged salmon were equally divided among eight identical depuration systems () resulting in ~275 kg fish biomass/tank and a corresponding fish density of ~55 kg/m3/tank. Feed was either offered for 4 days or withheld entirely over a 6-day period (n = 4 depuration systems), and time of stocking in the depuration systems was denoted as day 0.
Depuration systems
These experimental systems utilized a partial reuse design with a 5 m3 dual-drain culture tank, a gas conditioning column without packed media, and a low head oxygenator (). The systems had been emptied of fish and remained fallow for 2 months leading up to the study. Three days before the study began, the systems were cleaned, refilled, and disinfected by recycling water containing 200 mg/L hydrogen peroxide (35% Perox-Aid, Syndel, Ferndale, WA) for 24 h, and flow rates were measured and calibrated using a Model DXN Portable Ultrasonic Flow Meter (Dynasonics, Racine, WI). Each system recirculated approximately 340 L/min, and 26 L/min of makeup water was added continuously resulting in a 3.4-h hydraulic retention time, and cumulative use of approximately 800 L of makeup water/kg of fish during the 6-day depuration cycle. A 24-h photoperiod was provided via overhead light-emitting diode (LED) lights (4,000 lumen, 5,000K color temperature: Lithonia Lighting, Conyers, GA).
Feeding
The same fishmeal-based salmon diet with 42% protein and 26% fat (Cargill Inc., Minneapolis, MN) was delivered in four systems over 48 equally spaced events using 1 rpm T-Drum 2000 feeders (Arvotec, Huutokoski, Finland). A daily feeding rate of 0.7 kg of feed per tank was utilized, equating to 0.2% of the fish biomass. Salmon were offered feed from day 1.0 to 4.5 of the 6-day depuration period, where one day of initial feed withdrawal was provided to alleviate stress after stocking, and 1.5-days was implemented before slaughter to evacuate the gastrointestinal tract. Feeding activity was observed through plexiglass windows, and wasted feed was collected in radial flow settlers to assess feed consumption.
Fish sampling
A representative number of 3–4 kg salmon were randomly selected, humanely euthanized, and processed at the following study points: immediately after off-flavor exposure (7 fish), after 3 days of depuration (3 fish/tank), and after 6 days of depuration (5 fish/tank). Fewer fish were removed on day 3 to limit disruption of fish feeding response. Sacrificed fish were processed to characterize compartmented weight loss and processing metrics associated with blood, viscera, gastrointestinal contents, and the HOG carcass. To begin this procedure, salmon were weighed to assess post-depuration weight loss, and then the fish were exsanguinated and reweighed to assess blood loss. Next, the fish were eviscerated and weighed to record the mass of the HOG carcass. During this process, gastrointestinal tract contents were stripped into a plastic container to assess weight contribution of digesta and fecal material, and the gonads were separated and weighed to determine gonadosomatic index (GSI), an indicator of maturation status.
A consistent skin-on fillet section guided by dorsal and pectoral fin insertion, as shown in Davidson et al. (Citation2020), was collected from the same processed fish. Fillet color was assessed by the same person and confirmed by a second individual during each sampling event using a SalmoFan™. In addition, a separate group of 7 fish from the 3–4 kg population was humanely euthanized and processed on day 0. These fillets, along with those collected on day 6 were vacuum sealed, frozen, and delivered to West Virginia University’s Division of Food Sciences (Morgantown, WV) for proximate compositional analysis which was conducted using methods approved by the Association of Analytical Chemists (AOAC Citation1990).
Fish weight loss and processing calculations
The following calculations were utilized to determine weight loss and processing metrics. Weight/mass measurements are expressed in kg.
Off-flavor sampling and analysis
Skinless, 20–30 g fillet portions were removed from a consistent anterior location (Davidson et al. Citation2020) from the same processed fish on days 0, 3, and 6. Fillet portions were packaged separately in vacuum seal bags and frozen prior to shipment to U.S. Department of Agriculture, Agricultural Research Service (USDA-ARS), Thad Cochran Research Center (TCRC, University, MS). In preparation for analysis, each sample was cut into 1-cm cubes which were used to obtain distillate following methods outlined by Lloyd and Grimm (Citation1999). Each distillate sample was analyzed using solid phase microextraction-gas chromatography-mass spectrometry procedures (Davidson et al. Citation2019; Schrader et al. Citation2003, Citation2005), modified from the methods of Lloyd et al. (Citation1998). The instrumental detection limit for GSM and MIB in fillets was 1 ng/kg.
Glass scintillation vials (20 mL) were used to collect water from each depuration tank on days 0 to 6. Samples were refrigerated at 4–5°C and then shipped overnight in insulated coolers to TCRC for analysis of GSM and MIB. Off-flavor analysis in water followed procedures outlined in Davidson et al. (Citation2019), which were adopted and modified from Lloyd et al. (Citation1998). The instrumental detection limit for GSM and MIB in water was 1 ng/L.
Water chemistry sampling and analysis
Dissolved oxygen and temperature were recorded daily from LC3 Central Monitoring Systems integrated with Point Four™ RIU3s (Pentair Aquatic Ecosystems, Apopka, FL) and PRO-X DO probes (In Situ, Fort Collins, CO). Water samples were collected twice during the depuration period from tank side drains to assess background chemistry. Standard methods described in APHA (Citation2012) and HACH (Citation2015) were followed for analysis of alkalinity, total ammonia nitrogen (TAN), and total suspended solids (TSS). In addition, a portable analyzer and probe was used to measure carbon dioxide (Oxyguard International A/S, Farum, Denmark), and a HQ40d meter with PHC101 probe (Hach Company, Loveland, CO) was used to assess pH.
Economic analysis
At the time of reporting, market prices for farmed Atlantic salmon and aquafeed were volatile due to inflation and market instability. Therefore, an average price of $7.00 USD/kg for fresh, whole Atlantic salmon was estimated based on 2019–2022 reporting from the Norwegian salmon farming industry (NASDAQ Citation2022; Undercurrent News Citation2022). A price of $3.00/kg including freight was estimated for a RAS-specific diet based on in-house purchasing experience from commercial feed suppliers. Mean weight loss percentages from both treatments were multiplied by the estimated value per kg salmon and then multiplied again by current maximum and proposed annual production volumes from salmon RAS facilities including 2,000 mT, 5,000 mT, and 10,000 mT to estimate the cost implications of fish weight loss. Feeding costs were estimated based on a daily ration of 0.2% of fish biomass and added to revenue loss for that treatment. These calculations were carried out as follows:
Statistical analysis
Study 1 data including fish weights, weight loss metrics, processing yields, and fillet color were expressed as the mean ± standard error of sampled fish. Two fish with mean weights ≥10 kg, which exceeds relevant market-size (NASDAQ Citation2022), were excluded from data analysis. Additionally, when analyzing data from processed fish, several outliers associated with blood loss were identified using the interquartile range method and removed. Each data set was tested for normality using the Shapiro–Wilk test, and homogeneity of variance was confirmed. Fish weight, weight loss, and weight loss percentages measured over time for the same PIT tagged fish were tested for differences using repeated measures analysis of variance (ANOVA). Post-hoc, pairwise comparisons were then conducted via Bonferroni analysis. Data collected from processed fish including weights, weight loss, blood loss, visceral mass, processing loss, HOG yield, and fillet color was tested for differences over time using a one-way ANOVA. Differences between sampling days were assessed using a Tukey’s test.
During Study 2, weight loss, processing metrics, and product quality means were calculated by pooling data collected from fish from replicate tanks within treatment at each sampling point. One outlier associated with lipid level was identified using the interquartile range method and removed from the data set, and fish with GSI ≥ 2.0% were removed from analysis due to expected impacts on fillet proximate composition, lipid mobilization, and processing yields which are common in maturing Atlantic salmon (Davidson et al. Citation2018). Concentrations of GSM and MIB and water quality data were expressed as the grand mean and standard error across tanks within treatment (n = 4). Each data set was tested for normality using the Shapiro–Wilk test, and homogeneity of variance was confirmed. The Student’s t-test was then used to check for differences between treatments at each sampling point. Statistical analyses for both studies were carried out using SYSTAT software version (Version 13.2, San Jose, CA), and a probability level of 0.05 was used to determine differences between treatments for water quality, off-flavor, fish weight, weight loss, processing variables, fillet color, and proximate composition metrics.
Results
Study 1 – preliminary weight loss assessment
Pilot study results confirmed that Atlantic salmon lose weight over time when feed is continuously withheld during the depuration process. Salmon with an initial weight of 7.992 ± 0.308 kg lost 2.1 ± 0.3, 3.4 ± 0.2, and 4.3 ± 0.2% of their original body weight on days 6, 10, and 14, respectively. Weight loss percentage was significantly greater at each sampling point when the same PIT-tagged fish were repeatedly measured (P < .05). Conversely, weight loss percentage was not affected over the same intervals when comparing different groups of fish that were removed for processing (). Salmon that were processed on days 6, 10, and 14 lost 3.2 ± 0.3, 3.1 ± 0.5, and 3.6 ± 0.3% of their initial body weight, respectively (P > .05) (). Other notable findings included significantly lower blood loss percentage at day 10 versus days 0 and 14 and lower fillet color at days 6 and 10 versus days 0 and 14 (P < .05) ().
Table 1. Pilot study weight loss and processing data (mean ± standard error) from Atlantic salmon after 0, 6, 10, and 14 days of feed withdrawal during the depuration process.
Study 2 – feeding vs. fasting
Feeding observations and fish weight loss
Consumption of feed by the fish was observed through plexiglass windows and further validated via water chemistry. Significantly greater alkalinity, TAN, and TSS levels were measured in the feeding treatment (P < .05) (). Nevertheless, salmon did not feed aggressively, and substantial amounts of wasted feed were collected each day in radial flow settlers. Wasted feed was not quantified, but visual inspection indicated that approximately 50% of offered feed was uneaten; however, fed salmon lost less weight than fasted fish (P < .05). Fed and fasted salmon that were PIT-tagged and resampled lost 1.9 ± 0.7 and 6.6 ± 0.5 g of their initial weight per day, equating to 0.3 and 1.1% total weight loss, respectively (). In addition, 31.3% of salmon in the feeding treatment gained or maintained baseline weight compared to 9.2% of the fasted fish (). A similar trend in total weight loss was observed on day 6 in separate groups of fish that were processed (). In context to weight loss differences identified at the end of the study, it is important to note that a small amount of digesta, e.g., 0.1 ± <0.1% of the total fish body weight, was stripped from the digestive tract of fed salmon (); however, this did not influence differences in weight loss. In addition, visceral mass percentage and total processing loss, which was dominated by visceral discard, were significantly greater in fed salmon at day 6 (P < .05) (). Accordingly, HOG yield was significantly lower in fed salmon (P < .05). Moreover, gonadosomatic index averaged 0.3–0.7% and was similar between treatments () indicating that studied fish were immature and representative of typically marketed salmon.
Table 2. Water quality concentrations (mean ± standard error; n = 4 depuration systems) for feeding and fasting treatments during the 6-day depuration period.
Table 3. Weight loss metrics (mean ± standard error; n = 4 depuration systems) for 25 PIT-tagged salmon per tank from one day prior to transport to depuration systems through day 6 of the depuration period in feeding and fasting treatments.
Table 4. Fish processing metrics, compartmented weights, and weight loss (mean ± standard error) assessed on days 0, 3, and 6 of the depuration period.
GSM and MIB concentrations
Exposure to concentrated GSM and MIB before Study 2 increased concentrations of these off-flavor compounds in salmon flesh from 10 ± 1.3 and 64.3 ± 3.4 ng/kg, respectively to >1,450 ng/kg for each compound to begin the experiment. Thereafter, GSM and MIB levels in salmon flesh declined exponentially (). On day 3, GSM and MIB levels in salmon fillets were statistically similar between treatments (P > .05); however, differences were detected on day 6 (). Geosmin levels measured in fed and fasted salmon were 94.7 ± 7.6 and 122.9 ± 5.0 ng/kg, respectively, and MIB levels were 6.7 ± 0.8 and 10.1 ± 0.6 ng/kg, respectively (P < .05). Concentrations of both compounds were lower in salmon from the feeding treatment at the end of the study (P < .05).
Figure 2. Geosmin and MIB concentrations in Atlantic salmon flesh from feeding and fasting treatments during the 6-day depuration period (mean ± standard error; n = 4 depuration systems).

Figure 3. Geosmin and MIB concentrations in Atlantic salmon flesh from feeding and fasting treatments on days 3 and 6 of the depuration period (mean ± standard error; n = 4 depuration systems).

Moreover, low off-flavor concentrations were detected in depuration system water for both treatments. MIB peaked at 2.8 ± 0.6 ng/L on day 0 for the fasting treatment and 2.8 ± 0.6 ng/L on day 2 for the feeding treatment. Thereafter, waterborne MIB declined to levels at or below the minimum detection limit of 1 ng/L for both treatments. Geosmin levels in depuration system water were ≤1 ng/L throughout the study.
Product quality
SalmonFan™ fillet color scores averaged 29.3 ± 0.4 on day 0 before fish were divided into the depuration systems. On day 3, a significant drop in fillet color was measured in fed and fasted salmon, i.e., 26.4 ± 0.1 and 27.0 ± 0.2, respectively () compared to the original baseline (P < .05). However, fillet color recovered by day 6, reflecting similar scores as those recorded at the beginning of the study (). Fillet color was not affected by feeding treatment at any sampling point (P > .05). In addition, fillet proximate composition percentages, including moisture, crude fat, and ash were not affected by treatment and did not vary substantially from day 0 to day 6 (P > .05). However, fillet protein was significantly greater in fed salmon at day 6 compared to baseline levels (P < .05), albeit the difference between means was only a fraction of a percent ().
Figure 4. SalmoFan™ Lineal fillet color measurements (mean ± standard error of fish pooled/treatment (n)) at various sampling points before and during the 6-day depuration study.

Table 5. Fillet proximate composition (mean ± standard error) of fish sampled before the study and from feeding and fasting treatments after 6 days of depuration.
Economic analysis
When considering weight loss data from the fasting treatment, RAS operations producing 2,000, 5,000, and 10,000 mT of salmon per year and selling fish by weight as fresh, whole product would lose approximately $154,000, $385,000, and $770,000, respectively, when depurating salmon for 6 days (). A minor revenue deficit due to weight loss would still be incurred when feeding salmon during depuration; however, low ration feeding could save a salmon RAS operation $100,000, $250,000, and $500,000/year, respectively, at the previously described production volumes (). Economic analysis associated with the sale of a HOG product was not calculated, because weight loss in the sellable fish carcass appeared to be negligible.
Table 6. Economic implications of weight loss in fresh, whole Atlantic salmon that were fed or fasted during part of a 6-day depuration period expressed per unit fish weight and extrapolated to 2,000, 5,000 mT, and 10,000 mT commercial salmon RAS facilities. Values are reflected in U.S. dollars.
Discussion
Study 1 results confirmed that market-size Atlantic salmon lose weight during fasting periods that coincide with depuration. This outcome was similar to the results reported by Burr et al. (Citation2012), but the current studies were conducted under different experimental conditions including water temperature, Atlantic salmon size, fish origin/genetics, and duration of the preliminary feed withholding period (). The present studies also utilized an alternate approach to assess fish weight loss in addition to a slightly modified procedure employed by Burr et al. (Citation2012). This new approach, which included weighing the same individual, PIT-tagged fish on consecutive sample days, provided a better representation of fish weight loss over time. This assertion was corroborated, in part, by indistinct trends in fish weight loss that resulted when assessing different groups of fish of slightly different size during Study 1. Nevertheless, repeated fish sampling inhibits characterization of processing metrics, partitioned weight loss, fillet product quality, and maturation status; therefore, both sampling techniques provided value during the present studies.
Table 7. Comparison of methodologies, study metrics, and weight loss results between a similar depuration study (Burr et al. Citation2012) and the present studies.
In addition, after Study 1, it was concluded that residual waste in the gastrointestinal tract contributes to measured fish weight loss if the gut is not effectively cleared prior to baseline assessment. When reviewing the methods of Burr et al. (Citation2012), the authors realized that a preliminary feed withdrawal period was not provided before that study commenced; therefore, added weight was likely imparted by digesta and fecal waste which would have been subsequently eliminated via fish excretion (). This theory appears to be corroborated when comparing weight loss results from the present studies with those of Burr et al. (Citation2012) (). For example, Study 1 found that salmon that were deprived of food one day before baseline assessment lost 2.1% of their body weight after 6 days of depuration, while Burr et al. (Citation2012) reported 3.9% weight loss after just 5 days of depuration. This nearly 2-fold difference suggests that a portion of the loss reported by Burr et al. (Citation2012) was associated with excreted waste. Several studies provide further support, including Einen, Waagan, and Thomassen (Citation1998) who reported that market-size Atlantic salmon take ≤3 days to empty their gastrointestinal tract after cessation of feeding, and Davidson et al. (Citation2023) which measured a spike in excreted solids after stocking Atlantic salmon in a depuration system following one day of preliminary feed withdrawal. These considerations led to extension of the preliminary off-feed period from 1 to 2 days before the start of Study 2 and provided impetus to assess the weight contribution of digestive tract contents during the second experiment.
In addition, maturation status which significantly impacts processing yields and lipid levels in adult Atlantic salmon (Davidson et al. Citation2018) was not documented during Study 1. It was subsequently hypothesized that differing maturity status may have caused variation in weight loss over time when comparing different groups of market-size fish of slightly different mean sizes (). Therefore, GSI assessment was added during Study 2 to limit data variability, and salmon with GSI indicative of maturation were removed from analysis. Cumulatively, new perspectives gleaned during Study 1 led to optimization of data collection procedures for the second study.
During Study 2, which evaluated the effects of feeding and fasting during the depuration process, Atlantic salmon did not feed aggressively, and substantial amounts of uneaten feed were observed. However, the salmon still consumed enough feed to limit weight loss that otherwise occurred in fasted fish (). The economic importance of that mitigated weight loss is reflected in , which indicates that feeding would reduce revenue loss by >60% compared to the typical fasting approach when employing a 6-day depuration period. However, the economic impact likely depends on the form of the final product intended for sale. For example, RAS operations that sell market-size salmon as whole, uncut product to processors or distributors would be subject to the full value of pre-harvest weight loss shown in . However, anonymous communication with salmon RAS managers indicates that many operations either eviscerate or fillet fish before farmgate sale. As evidenced during Study 2, most of the salmon weight loss occurred in the visceral mass (); therefore, the impact of weight loss on the sale of HOG products would only be related to minor loss occurring in the edible fish carcass, which appears to be negligible (). The economics for sale of HOG products could also be influenced by decisions to pay for facility removal of fish processing waste (e.g., the viscera) on a per weight basis, or alternately, by value-added sale or sustainable reuse of processing byproducts (Choudhury et al. Citation2022).
Of equal importance to remediated weight loss, this study also found that feeding a low ration to depurating Atlantic salmon did not negatively impact off-flavor elimination rate. On the contrary, feeding resulted in significantly lower GSM and MIB levels in Atlantic salmon flesh after 6 days of depuration. Positive effects of feeding depurating fish were previously reported by Schram et al. (Citation2021) who found that feeding tilapia resulted in slightly faster GSM excretion due to increased gill ventilation rates. Conversely, Hvas, Stien, and Oppedal (Citation2020) reported that fasting Atlantic salmon demonstrated significantly lower metabolic rates when feed was initially withheld. Therefore, differences in salmon metabolism and gill ventilation rates in feeding and fasting fish likely contributed to the measured differences in GSM and MIB elimination between treatments during Study 2. However, the differences in the measured fillet off-flavor concentrations were small in magnitude () and possibly of limited importance to human taste or the required duration of the depuration period (Burr et al. Citation2012; Davidson et al. Citation2020).
Other practical information was gleaned that could be useful to land-based Atlantic salmon producers that opt to feed depurating fish. For example, due to the non-aggressive feed capture response, a modified feeding approach might be required. Uneaten feed was estimated at 50% of the total delivered amount, suggesting that the daily feeding rate could be reduced from 0.2% of the tank biomass to approximately 0.1%. Moreover, salmon may not adjust to a different automated feeding system or feed delivery location following transport from RAS; therefore, handfeeding could be considered as an alternate approach to match feed delivery rate with the rate of feed consumption. In the context of this discussion, it is important to note that extra fish handling was required to collect the required data and to transport salmon to depuration systems compared to typical harvest procedures (Summerfelt et al. Citation2009), which may have impacted fish feeding response.
In addition, water quality results from this study emphasize that cleaning and disinfection protocols should be applied between depuration stocking events if feeding is adopted. Added nutrients from feed and fish waste likely promote the growth of GSM and MIB-producing actinomycetes and the subsequent production of GSM and MIB. Schrader (Citation2020) reported that relatively high ammonia levels were associated with increased GSM and MIB production by Streptomyces luridiscabiei, a species of actinomycete originally isolated from a RAS. Moreover, Sarker et al. (Citation2014) found that GSM concentrations in rainbow trout fillets were related to increasing dietary phosphorus and associated phosphorous waste in RAS water. Increased nutrient concentrations imparted by feeding depurating salmon could have implications for reuse of depuration system effluents as supply water in RAS or compliance with local pollution discharge standards at RAS facilities (Davidson et al. Citation2023).
Additionally, findings related to salmon fillet color could be important to adoption of specific depuration procedures such as established purging duration or fish handling techniques. For example, decreased fillet color was observed at days 6 and 10 of Study 1 () and on day 3 of Study 2 (). However, by the end of both studies, fillet color had recovered to baseline SalmoFan™ Lineal scores of 29–30. Burr et al. (Citation2012) reported a similar trend, but with a drop in fillet color after 15 days of depuration and return to baseline levels by day 20. The perceived intensity of red/orange color of salmon fillets strongly influences consumer purchasing (Michie Citation2001; Steine, Alfnes, and Rørå Citation2005); therefore, additional research may be required to determine if fish transport, harvesting procedures, or other aspects of the depuration process impact fillet color in RAS-produced salmon.
Conclusions
Feeding market-size Atlantic salmon during the depuration process appears to be a feasible approach that minimizes weight loss without negatively impacting GSM and MIB elimination rate. Feeding salmon during depuration could also provide economic benefits for salmon RAS facilities. However, decisions to feed low rations during depuration likely depend on site-specific aspects including form of the final product at farmgate sale and associated economics, value-added use or sale of fish processing wastes, the frequency of depuration system cleaning and disinfection, and protocols for secondary use or discharge of depuration system effluents. Future research evaluating the implications of feeding over longer depuration periods may be beneficial to commercial salmon RAS operations.
Acknowledgments
This research was supported by Research Grant No. IS-5354-21 from BARD, The United States – Israel Binational Agricultural Research and Development Fund. Special thanks are extended to Freshwater Institute staff who supported this project including Curtis Crouse – fish production, Natalie Redman and Megan Murray – water and environmental chemistry analysis, Shanen Cogan and JC Stanley – construction and operations, Kata Sharrer – system drawings and schematics, and USDA-ARS biological science technician Phaedra Page – laboratory technical assistance. Gratitude is also extended to Drs. Jaap van Rijn and Snir Azaria for their collaboration on this project and to Dr Brett Kenney and Mr Sean Estep at West Virginia University for supporting product quality analyses.
Disclosure statement
No potential conflict of interest was reported by the author(s).
Data availability statement
Data will be made available upon request.
Additional information
Funding
References
- American Public Health Association (APHA). 2012. Standard methods for the examination of water and wastewater. 22nd ed. Washington, DC: American Public Health Association, American Water Works Association, and Water Environment Federation.
- AOAC (Association of Analytical Chemists). 1990. Official methods of analysis. 15th ed. Washington D.C., USA: AOAC International.
- Azaria, S., and J. van Rijn. 2018. Off-flavor compounds in recirculating aquaculture systems (RAS): Production and removal processes. Aquacultural Engineering 83:57–64. doi:10.1016/j.aquaeng.2018.09.004.
- Blevins, W. T., K. K. Schrader, and I. Saadoun. 1995. Comparative physiology of geosmin production by Streptomyces halstedii and anabaena sp. Water Science and Technology 31 (11):127–33. doi:10.2166/wst.1995.0419.
- Borderías, A. J., and I. Sánchez-Alonso. 2011. First processing steps and the quality of wild and farmed fish. Journal of Food Science 76 (1):R1–R5. doi:10.1111/j.1750-3841.2010.01900.x.
- Burr, G. S., W. R. Wolters, K. K. Schrader, and S. T. Summerfelt. 2012. Impact of depuration of earthy-musty off-flavors on fillet quality of Atlantic salmon, Salmo salar, cultured in a recirculating aquaculture system. Aquacultural Engineering 50:28–36. doi:10.1016/j.aquaeng.2012.03.002.
- Choudhury, A., C. Lepine, F. Witersa, and C. Good. 2022. Anaerobic digestion challenges and resource recovery opportunities from land-based aquaculture waste and seafood processing byproducts: A review. Bioresource Technology 354:127144. doi:10.1016/j.biortech.2022.127144.
- Davidson, J., N. Crouse, C. Redman, and B. Vinci. 2023. Water quality, waste production, and off-flavor characterization in a depuration system stocked with market-size Atlantic salmon Salmo salar. Journal of the World Aquaculture Society 54 (1):96–112. doi:10.1111/jwas.12920.
- Davidson, J., C. Grimm, S. Summerfelt, G. Fischer, and C. Good. 2020. Depuration system flushing rate affects geosmin removal from market-size Atlantic salmon Salmo salar. Aquacultural Engineering 90:102104. doi:10.1016/j.aquaeng.2020.102104.
- Davidson, J., P. B. Kenney, F. T. Barrows, C. Good, and S. T. Summerfelt. 2018. Fillet quality and processing attributes of post-smolt Atlantic salmon, Salmo salar, fed a fishmeal-free diet and a fishmeal-based diet in recirculation aquaculture systems. Journal of the World Aquaculture Society 49 (1):183–96. doi:10.1111/jwas.12452.
- Davidson, J., K. Schrader, E. Ruan, B. Swift, J. Aalhus, M. Juarez, W. Wolters, G. Burr, C. Good, and S. T. Summerfelt. 2014. Evaluation of depuration procedures to mitigate the off-flavor compounds geosmin and 2-methylisoborneol from Atlantic salmon Salmo salar raised to market-size in recirculating aquaculture systems. Aquacultural Engineering 61:27–34. doi:10.1016/j.aquaeng.2014.05.006.
- Davidson, J., S. Summerfelt, C. Grimm, G. Fischer, and C. Good. 2021. Effects of swimming speed and dissolved oxygen on geosmin depuration from market-size Atlantic salmon Salmo salar. Aquacultural Engineering 95:102201. doi:10.1016/j.aquaeng.2021.102201.
- Davidson, J., S. Summerfelt, K. K. Schrader, and C. Good. 2019. Integrating activated sludge membrane biological reactors with freshwater RAS: Preliminary evaluation of water use, water quality, and rainbow trout Oncorhynchus mykiss performance. Aquacultural Engineering 87:102022. doi:10.1016/j.aquaeng.2019.102022.
- Einen, O., B. Waagan, and M. S. Thomassen. 1998. Starvation prior to slaughter in Atlantic salmon (Salmo salar) I. Effects on weight loss, body shape, slaughter- and fillet-yield, proximate and fatty acid composition. Aquaculture 166 (1–2):85–104. doi:10.1016/S0044-8486(98)00279-8.
- Engle, C. R., G. L. Pounds, and M. van der Ploeg. 1995. The cost of off-flavor. Journal of the World Aquaculture Society 26 (3):297–306. doi:10.1111/j.1749-7345.1995.tb00258.x.
- HACH Company. 2015. HACH water analysis handbook. 8th ed. Loveland, Colorado, USA: Hach Company.
- Howgate, P. 2004. Tainting of farmed fish by geosmin and 2-methyl-iso-borneol: A review of sensory aspects and of uptake/depuration. Aquaculture 234 (1–4):155–81. doi:10.1016/j.aquaculture.2003.09.032.
- Hvas, M., L. H. Stien, and F. Oppedal. 2020. The metabolic rate response to feed withdrawal in Atlantic salmon post-smolts. Aquaculture 529:735690. doi:10.1016/j.aquaculture.2020.735690.
- Kropp, K., S. T. Summerfelt, K. Woolever, S. A. Johnson, D. W. Kapsos, C. Haughey, and T. P. Barry. 2022. A novel advanced oxidation process (AOP) that rapidly removes geosmin and 2-methylisoborneol (MIB) from water and significantly reduces depuration times in Atlantic salmon Salmo salar RAS aquaculture. Aquacultural Engineering 97:102240. doi:10.1016/j.aquaeng.2022.102240.
- Lindholm-Lehto, P. C., and J. Vielma. 2019. Controlling of geosmin and 2-methylisoborneol induced off-flavours in recirculating aquaculture system farmed fish—a review. Aquaculture Research 50 (1):9–28. doi:10.1111/are.13881.
- Lloyd, S. W., and C. C. Grimm. 1999. Analysis of 2-methylisoborneol and geosmin in catfish by microwave distillation-solid-phase microextraction. Journal of Agricultural and Food Chemistry 47 (1):164–69. doi:10.1021/jf980419x.
- Lloyd, S. W., J. M. Lea, P. V. Zimba, and C. C. Grimm. 1998. Rapid analysis of geosmin and 2-methylisoborneol in water using solid phase micro extraction procedures. Water Research 32 (7):2140–46. doi:10.1016/S0043-1354(97)00444-2.
- Michie, I. 2001. Causes of downgrading in the salmon farming industry. In Farmed fish quality, ed. S. C. Kestin and P. D. Warriss, Vol. 4, 129–36. Oxford, UK: Fishing News Books.
- NASDAQ. 2022. Accessed November 4, 2022. https://salmonprice.nasdaqomxtrader.com/public/report?1.
- Popovic, N. T., I. Strunjak-Perovic, R. Coz-Rakovac, J. Barisic, M. Jadan, A. P. Berakovic, and R. S. Klobucar. 2012. Tricaine methane-sulfonate (MS-222) application in fish anaesthesia. Journal of Applied Icthyology 28 (4):553–64. doi:10.1111/j.1439-0426.2012.01950.x.
- Robb, D. H. F. 2008. Welfare of fish at harvest. In Fish welfare, ed. E. J. Branson, 217–41. Oxford: Blackwell Publishing Ltd. doi:10.1002/9780470697610.ch14.
- Sarker, P., A. Pilotz, M. Auffret, É. Proulx, R. Villemur, M.-H. Deschamps, and G. Vandenburg. 2014. Reducing geosmin off-flavor compounds and waste outputs through dietary phosphorus management in rainbow trout aquaculture. Aquaculture Environment Interactions 6 (1):105–17. doi:10.3354/aei00119.
- Schrader, K. K. 2020. Effects of relevant ammonium chloride concentrations on biomass and off-flavor compound production by Streptomyces luridiscabiei originating from a recirculating aquaculture system. Journal of Applied Aquaculture 32 (4):380–85. doi:10.1080/10454438.2019.1610540.
- Schrader, K. K., S. Acuña-Rubio, R. H. Piedrahita, and A. M. Rimando. 2005. Geosmin and 2-methylisoborneol cause off-flavors in cultured largemouth bass and white sturgeon reared in recirculating-water systems. The North American Journal of Aquaculture 67 (3):177–80. doi:10.1577/A04-070.1.
- Schrader, K. K., N. P. D. Nanayakkara, C. S. Tucker, A. M. Rimando, N. Ganzera, and B. T. Schaneberg. 2003. Novel derivatives of 9,10-anthraquinone are selective algicides against the musty-odor cyanobacterium oscillatoria perornata. Applied and Environmental Microbiology 69 (9):5319–27. doi:10.1128/AEM.69.9.5319-5327.2003.
- Schram, E., C. Kwadijk, A. Hofman, A. Blanco, A. Murk, J. Verreth, and J. Schrama. 2021. Effect of feeding during off-flavour depuration on geosmin excretion by Nile tilapia (Oreochromis niloticus). Aquaculture 531:735883. doi:10.1016/j.aquaculture.2020.735883.
- Steine, G., F. Alfnes, and M. B. Rørå. 2005. The effect of color on consumer WTP for farmed salmon. Marine Resource Economics 20 (2):211–19. doi:10.1086/mre.20.2.42629470.
- Summerfelt, S. T., J. Davidson, G. Wilson, and T. Waldrop. 2009. Advances in fish harvest technologies for circular tanks. Aquacultural Engineering 40 (2):62–71. doi:10.1016/j.aquaeng.2008.12.001.
- Swanson, D., R. Block, and S. A. Mousa. 2012. Omega-3 fatty acids EPA and DHA: Health benefits throughout life. Advances in Nutrition 3 (1):1–7. doi:10.3945/an.111.000893.
- Tucker, C. S. 2000. Off-flavor problems in aquaculture. Reviews in Fisheries Science 8 (1):45–88. doi:10.1080/10641260091129170.
- Undercurrent News. 2022. Accessed December 6, 2022. https://www.undercurrentnews.com/data/prices/atlantic-salmon/.