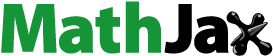
Abstract
Acne Vulgaris is one of the most common chronic inflammatory skin disorders that affect majority of teen-agers worldwide. Isotretinoin (ITT) is the drug of choice in the management of acne, but, it suffers from serious side-effects including hepatotoxicity, and some psychological disturbances following its oral intake. The objective of this study was to develop and optimize ITT loaded nanoemulsions (ITT-SNEDDS) and to incorporate resveratrol (RSV)in optimum formulation to decrease ITT side effects The ITT solubility was first tested in various essential oils, surfactants, and co-surfactants to select the essential nanoemulsion ingredients. Mixture design was applied to study the effect of independent variables and their interactions on the selected dependent responses. The developed ITT-SNEDDS were characterized for their globule size and ex vivo permeation. The optimized batch was further loaded with RSV and evaluated for in vitro and ex vivo permeation and for in vivo hepatotoxicity. The developed ITT-SNEDDS exhibited globule size below 300 nm, up to 272.27 ± 7.12 mcg/cm2.h and 61.27 ± 2.83% of steady-state flux (JSS) and permeability % respectively. Optimum formulation consisted of 0.15 g oil mixture, 0.6 g of surfactant (Labrasol), and 0.250 g co-surfactant (Transcutol). Permeability studies confirmed the enhanced permeation percentage of ITT (40.77 ± 1.18%), and RSV (29.94 ± 2.02%) from optimized formulation, with enhanced steady-state flux (JSS). In vivo studies demonstrated the superior hepatoprotective activity of optimized formulation compared to a different drug formulations and marketed product. Therefore, RVS loaded ITT-SNEDDS might be a successful strategy for acne management with improved action, and minimum side effects.
1. Introduction
Acne, the most frequent dermatological condition, is observed typically in adolescents, but it may affect individuals of all ages (Decker & Graber, Citation2012). The treatment effects can be assessed only after 2–3 months of use, and the therapy can be manipulated (reduced or maintained or increased) accordingly (Asai et al., Citation2016). Among several medications for dermatological application, isotretinoin (ITT) has been the most widely used drug. Chemically, ITT is (2Z, 4E, 6E, 8E)-3,7-Dimethyl-9-(2,6,6-trimethylcyclohex-1-enyl)nona-2,4,6,8-tetraenoic acid. The oral form of ITT (13-cis-retinoic acid) was initially approved by the USFDA (U.S. Food and Drug Administration) in 1982 to treat severe acne (Layton, Citation2009), and its application has been extended to reduce the development of sebum to significantly trim down the inflammatory lesions (Nelson et al., Citation2006). ITT has a unique importance in treating adult women with acne, as it is non-hormonal and non-antibiotic. ITT is effective because of the improved production of lipocalin through neutrophil gelatinase (anti-microbial protein). Over the decades, no other drugs or treatments have diminished the effectiveness of ITT. Nonetheless, despite these fascinating features, extremely low solubility and permissibility can limit the incorporation of ITT into a suitable vehicle and lead to poor patient compliance. Consequently, it is prudent to enhance the solubility and minimize the adverse effects using an appropriate carrier.
Stilbenes and natural phenolic compounds are found in various food sources, especially berries. One such compound is resveratrol (RSV), chemically 3,4,5-trihydroxystilbene, available in two different forms, cis-RSV and trans-RSV, with the trans form being a more stable compound (Baxter, Citation2008; Kala et al., Citation2012). RSV has been extensively researched because of its renowned biological activities. Pharmacologically, the compound has been shown to have anti-aging (De La Lastra & Villegas, Citation2007), anti-inflammatory (Baxter, Citation2008), cardio-protective (Das & Das, Citation2007), anti-oxidant (Hung et al., Citation2000), and anti-carcinogenic (Chen et al., Citation2015) effects. Additionally, RSV can decrease the levels of alanine aminotransferase (AST), aspartate aminotransferase (ALT), triglycerides, LDL-Cholesterol, and total cholesterol by regulating liver lipid metabolism (Peiyuan et al., Citation2017). RSV has been proven as an efficient anti-inflammatory and hepatosteatosis material in controlling the alcoholic liver disease. As Peiyuan et al. indicated, the hepatoprotective effect of RSV can be measured by regulating several pathways, such as apoptosis, oxidative stress, and swelling (Planas et al., Citation2012). Drug-mediated hepatotoxicity further confirmed the mechanism of RSV through the generation of free radicals and cytokines. RSV is significant in treating several liver diseases due to its antioxidant and anti-inflammatory properties.
The potential application of RSV as a therapeutic agent has been limited due to photosensitivity and partial intestinal absorption, thus decreasing bioavailability and causing extensive elimination and catabolism through ABC transporters (Karthikeyan et al., Citation2013; Vargas et al., Citation2016). Various formulations, including loading RSV into solid lipid nanoparticle, liposomes, and gelatin nanoparticles, have been applied to conquer these limitations (Teskač & Kristl, Citation2010; Coimbra et al., Citation2011; Abdelbary et al., Citation2017).
Topical route is a very well established pathway of drug administration that have the advantage of improved local therapeutic effect, convenience of use, and the possibility of stopping treatment if necessary (Satalkar et al., Citation2016). It also avoids drug metabolism in liver and hence maximizes the therapeutic benefit of drugs
Self-nano emulsifying delivery systems (SNEDDS), on dilution, produce nano-sized transparent systems called nanoemulsions (Khalid & El-Sawy, Citation2017; Hosny et al., Citation2019; Alshehri et al., Citation2020). These systems have been researched extensively, as they can offer higher stability and enhanced solubility, finally, improving the bioavailability of many insoluble or partially soluble therapeutic agents (Venkatesh & Mallesh, Citation2013; Wu et al., Citation2013). Nanoemulsions can improve the efficacy and functionality of several natural and chemical active moieties. Many therapeutic agents, like antioxidants, non-steroidal anti-inflammatory drugs, and lipids, were successfully formulated using nanoemulsions as novel carriers for topical application. Besides, nano-emulsion has also been used for the decontamination of radio nuclei and photodynamic therapy. Consequently, nanoemulsions demonstrate the enormous application in the area of dermatology (Aqil et al., Citation2016).
Topical SNEDDS have shown good properties compared to other topically applied drug delivery systems (Çinar, Citation2017; Alshehri et al., Citation2020). Such advantages include, being noninvasive, cost-effective, able to entrap a broad spectrum of hydrophilic and hydrophobic drugs, ability to protect several agents from hepatic metabolism and diminish their unwanted side effects. Moreover, SNEDDS’ components often acquire good anti-microbial and anti-viral characteristics which results in well preserved formulations with prolonged stability. SNEDDS are usually easily developed since they require no sophisticated procedures or high energy methods (Sarker, Citation2005; Hosny et al., Citation2020). Although SNEDDS resemble microemulsions (MEs) in many aspects, however, NEs are more kinetically stable and also have much lower globule size that allow them to enhance drug dissolution and permeation through skin ending up increasing the drug’s therapeutic efficiency (Pathania et al., Citation2018).
Numerous scientific works were carried out over the years on the application of essential oils in formulating nanoemulsions. As the nanoemulsion comprises translucent, unstable immiscible phases, the prepared system is prone to flocculation, sedimentation, and coalescence (de Campos et al., Citation2012). Accordingly, essential oils have been used in formulating the nanoemulsions to acquire high stability. Furthermore, the utilization of essential oils in formulating nanoemulsions is readily scalable, economic, and eco-friendly (Malhi et al., Citation2017).
Tea tree oil extracted from Melaleuca alternifolia has a unique application in the treatment of acne. Owing to improved bacteriostatic activity and minimizing lesions formation, tea tree oil has been used to treat seborrheic dermatitis and acne vulgaris (Wren & Stucki, Citation2003). Baobab oil (extracted from Digitata Adansonia L) is an anti-diabetic, hepatoprotective agent, and several unique properties, like nonirritating hydration, support its potential application in the cosmetic area, as it can soften the dry skin by enormously absorbing the nutrients (Naguib et al., Citation2017).
Nanotechnology is the concept of manipulating active agents to be in the size range from 1 to 1000 nm. It is considered as the magic bullet for solving different problems associated with many drugs (Shafiq et al., Citation2007; Ahmed et al., Citation2015; Safwat et al., Citation2017; Yehia et al., Citation2017). The potential nanotechnology strategy circumvented the physicochemical and pharmacokinetic constraints of RSV and ITT. Nevertheless, very few studies have addressed the critical in vivo results of developed systems. Accordingly, this research intended to optimize the nanoemulsion-based formulation of RSV and ITT using Mixture design to improve their bioavailability and reduce their toxicity. Animal models were used to assess the hepatotoxicity of the optimized formulation.
2. Materials and methods
2.1. Materials
ITT was purchased from Wuhan Senwayer Century Chemical Co., Ltd (China). Essential oils were obtained from Hayward, CA, USA. RSV, was procured from Water Import and Export Co., Ltd (China). Triglycerides, propylene glycol monolaurate, and glycerol monooleate, Labrasol, and transcutol were generously gifted by Gattefosse (France). All other reagents and chemicals used were of analytical grades.
2.2. Determination of ITT solubility
The solubility of ITT in various oils, surfactants, and co-surfactants was determined. An excess amount of ITT was dissolved in about 2 ml of each vehicle individually. The mixture was then shaken on a water bath (Model-1031, GFL Corporation, Germany) for 24 h, and the temperature was maintained at 37 ± 0.5° C. Once the equilibrium was attained, the resulting mixture was centrifuged (Sigma, 3K30 Centrifuge, UK) at 8° C for 1 h at 15,000 rpm. The formed supernatant was then diluted with a suitable quantity of methanol and analyzed quantitatively for ITT concentration using a UV-Visible spectrophotometer at 362 nm. The experiment was repeated three times, and the values were noted as mean ± standard deviation.
2.3. Pre-formulation studies
Nine nanoemulsion formulations were designed with different proportions of the selected oils, surfactant, and co-surfactant using different concentrations of ITT (20–60 mg) in the first formulation only, then ITT concentrations from 20 to 40 were used in formulations 2 and 3. After that, the drug concentration that yielded the lowest globule size in formulations from 1 to 3 was used in the rest of the formulations. The composition of the 9 formulations was presented in .
Table 1. Various formulation strategies to study the solubility of ITT.
The SNEDDs were prepared as follows; ITT was added to the oil phase mixture; subsequently, a mixture of surfactant and co-surfactant was added. The formed mixture was further sonicated using Ultrasonic Processors (VCX 750, USA). Finally, the formed dispersion was added to distilled water drop by drop, and nanoemulsion was formed spontaneously on mixing at room temperatures (Ahmed et al., Citation2018).
The prepared SNEDDs were evaluated for their globule size, as follows; a specific quantity of each formulation was diluted with distilled water to form an aliquot of 20 ml, and samples were analyzed at 25° C using a dynamic light scattering technique in Zetatrac particle size analyzer (Miccrotrac Inc, PA, USA) (Salem et al., Citation2020).
Later on, 20 mg RSV were added to the best three formulations that gave the lowest globule size to detect in change in droplet size in case of using two active agents.
2.4. Mixture design for the development of ITT loaded SNEDDS
Statistical optimization of response surface methodology (R.S.M) has proven a proficient tool in optimizing the various parameters in a multifarious process to obtain the desired responses; thus, it has been applied to formulate ITT-SNEDDS. Among several models, the mixture design was selected to study the interaction of independent variables. Three factors were selected, namely, percentages of oil mixture [tea tree oil/baobab oil in ratio 1:1] (X1), surfactant [labrasol] (X2), and cosurfactant [transcutol] (X3; ). Data from the previous pre-formulation studies were used to choose the levels of independent variables. All these components were used selectively in several ratios, with a total concentration equaling to 100%. The effect of all these selected variables on globule size (Y1), steady-state inflow (JSS) (Y2), and penetrability percentage (Y3) was studied (Shafiq et al., Citation2007). A total of 16 runs were projected, and the variables are summarized in . The correlations between the selected variables and responses were further analyzed by applying the regression equation using Statgraphics Centurion XV software-15.2.05 version (StatPoint, Inc., USA). SNEDDS formulations were optimized for minimum globule size, highest JSS, and maximum permeation percentage.
Table 2. Experimental mixture design (components levels and selected responses).
Table 3. Mixture design for the development of ITT Loaded SNEDDS.
2.5. Preparation of ITT nanoemulsion
The different formulations of ITT nanoemulsion presented in were prepared as previously mentioned in Section 2.3 (Ahmed et al., Citation2018).
2.6. Characterization of ITT nanoemulsion
Prepared ISN was characterized by various parameters to study the effect of selected variables.
2.6.1. Measurement of globule size
The globule size of the developed ITT loaded SNEDDS was measured as previously described in Section 2.3.
2.6.2. Ex vivo permeation studies to evaluate JSS and permeation percentage
Ex vivo permeation study was conducted according to a previously reported method with modification (Kurakula et al., Citation2015). Wister albino male rats were collected from the Animal House for Pharmacy faculty, King Abdulaziz University. Hair of rats’ abdominal region was removed. Animals were sacrificed and certain the shaved abdominal skin (2 cm × 2 cm) was obtained, freed from the underlying tissues and finally used as a model permeation membrane. The Obtained skin was soaked in a phosphate buffer of pH 7.2 for one day before the commencement of the study. The permeation efficiency of SNEDDS was studied with a simple, low head dissolution testing instrument (PT-DT70, Siemensstr, Germany). The receptor compartment was filled with 900 ml of phosphate buffer with continuous stirring at 7 rpm. The temperature of the buffer solution was maintained at 37 ± 0.5 °C. Afterward, the skin segment was placed between the donor and receptor compartment so that the dermal side of the skin should be in close contact with the receptor compartment. The donor compartment contained volumes of SNEDDS equivalent to 20 mg ITT. At specific intervals, (1, 2, 3, 4, 5, 6, 8, 12 h) aliquots of 2 ml samples were withdrawn and analyzed using a UV-Visible spectrophotometer (Jenway 6705, USA). RSV and ITT were quantitatively measured at 362 nm and 299 nm, respectively. Finally, JSS and permeation percentages were determined
2.7. Optimization of SNEDDS formulations
The obtained results from the experimental runs were further analyzed using ANOVA (Analysis of Variance) with multiple response optimization and RSM. Optimized formulation was selected based on the constraints of selected responses.
2.8. The rationale of mixture design
After the optimization of experimental runs, an optimized formulation was prepared according to the concentrations proposed by the software, and its various parameters were evaluated. The rationale for the selected experimental design was validated by comparing the experimental values with predicted values and calculating relative error (%). The following equation was used to calculate relative error (Khallaf et al., Citation2020).
(1)
(1)
2.9. In vitro release studies
In vitro, drug release studies were performed to calculate the amount of ITT and RSV released for the optimized formulation according to a previously described method (CitationAlvarez-Figueroa & Blanco-Mendez, 2001). Low head dissolution testing instrument (PT-DT70, Siemensstr, Germany) with dialysis cellulose membrane of 1.7 cm2 diffusion area (Cut off 14,000 Da pore size, Sigma-Aldrich, USA) was utilized. First, the dialysis membrane was soaked in phosphate buffer (pH 7.4) for 15 min at 25 ± 0.5° C. The receptor compartment was filled with phosphate buffer, and glass tubes covered with the dialysis membranes and filled with certain volume of the optimized formulation equivalent to 5 mg of each drug were placed in the medium with continuous rotations (75 rpm). The temperature was maintained at 37 ± 0.5° C throughout the experiment. Samples were withdrawn at various time intervals (1, 2, 3, 4, 6, 9, and 12 h), and ITT and RSV concentrations were analyzed at 362 and 299 nm, respectively.
2.10. Ex vivo permeation study of optimized formulation
The permeation study for optimum formulation was carried out as previously described in Section 2.6.2, however the permeation parameters for both ITT and RSV were calculated.
2.11. In vivo studies
Thirty-six male adult mice weighing 20.5 ± 1.5g were selected and adapted for seven days before the study. The animals were divided into six groups consisting of six animals in each group. Animal groups received the following treatments; group 1 received normal saline orally and served as negative control, while group 2 received oral aqueous dispersion of ITT. Moreover, group 3 received topical aqueous dispersion of ITT, group 4 received topical optimized ITT-SNEDDS formulation, group 5 received optimized topical ITT-RSV-SNEDDS and finally group 6 received the marketed topical cream. The dose of ITT in tested formulations was 5 mg/kg, and the treatment period was 14 days. The tested rats were maintained at temperature of 25 ± 0.5 °C and 30 − 70% of relative humidity and subjected to 12 h darkness and 12 h light cycle. At the end of the study period, animals were sacrificed with cervical dislocation, blood samples were instantly withdrawn and finally dysfunction of the liver was estimated by measuring AST, ALT, superoxide dismutase (SOD), malondialdehyde (MDA), and glutathione peroxidase (GSH-Px).
2.12. Statistical analysis
The in vivo results obtained were expressed as mean ± S.D and analyzed using Statistical Package for the Social Science, version 26 (IBM, SPSS). One-way ANOVA was followed by a post-hoc Tukey test to determine significant differences between quantitative variables. p Value <.05 was noted as statistically significant.
3. Results and discussion
3.1. ITT solubility studies
The solubility of ITT in assorted vehicles is depicted in . The highest ITT solubility (29.54 ± 11.69 µg/ml) was found with Labrasol (a non-ionic-emulsifier), and comparable results were observed with other low soluble drugs, as specified by Patel et al. (Djekic & Primorac, Citation2008) In fact, labrasol could be considered one of the finest surfactants in increasing the solubility of hydrophobic drugs and it is worthy to mention that, Labrasol was frequently used for preparation of various topical micro and nanoemulsions (Djekic & Primorac, Citation2008; Moghimipour et al., Citation2012). Such character of Labrasol makes it the most productive element in improving the permeability and the most ideal candidate for SNEDDS production.
Also, ITT exhibited highest solubility with transcutol co-emulsifier (24.34 ± 14.28 µg/ml). Transcutol is known for its great miscibility in polar and non-polar solvents, as well as its competence to increase the absorption and degree of permeability (Osborne & Musakhanian, Citation2018; Vyas et al., Citation2010). Thus, it can serve as a carrier (co-surfactant) in formulating nanoemulsions. Essential oils also improved the solubility of ITT to a reasonable extent. Based on these results, surfactant-Labrasol, co-surfactant-Transcutol P, and essential oils (tea tree oil and baobab oil) were selected to formulate the nanoemulsion.
3.2. Preformulation studies
Globule sizes of all the prepared formulations were evaluated and data were presented in . First, the concentration of ITT to be used for further formulations was optimized. Formulation 1, having higher concentrations of ITT (60 mg, 40 mg), resulted in particle size of 315.03 ± 10.92 nm and 182.45 ± 25.81. However, ITT at 20 mg concentration formed the globules with lesser size (123.46 ± 5.85 nm). The identical phenomenon was observed with formulations 2 and 3. As a result, ITT of 20 mg concentration was used consistently to prepare the remaining formulations. Accordingly, decreased globule size was observed. Formulation 7 had the smallest globule size of 99.64 ± 4.69 nm. Formulation-9 had an elevated globule size of 299.66 ± 11.12 nm, which can be attributed to a higher concentration of Labrasol (0.35 g) and Transcutol (0.35 g).
Table 4. Composition of ITT in various formulations and their globule sizes.
3.3. Optimization of ITT loaded SNEDDS
Mixture design were used to analyze the effect of selected independent variables on minimum globule size (Y1), maximum JSS (Y2), and high permeability percent (Y3) (Kurakula & Naveen, Citation2020). The observed responses of the 16 runs are given in . Globule size of all the trail batches was found to be in the range of 217.67–387 nm. JSS and permeability percentages were estimated in the range of 98.54–272.27 mcg/cm2.h and 26.67%–61.27%, respectively. All obtained results were evaluated for individual responses. The effect of selected variables was further analyzed statistically using ANOVA and fx model.
Table 5. Projected trail formulations and their observed responses according to Mixture design.
Based on the fit summary of responses (adjusted and predicted R2) and the sequential sum of squares (Type-I), different models were selected (). No models were misidentified with the highest order polynomial equations (Naveen et al., Citation2020). The accuracy of the model was further evaluated using the normal probability of studentized residuals. Externally studentized results were scattered around the straight line with a slight deviation. ANOVA was further used to quantitatively analyze the relationship between the selected independent variables and responses as follows;
Table 6. Fit summary for responses.
3.3.1. Globule size (Y1):
The selected model was statistically significant, F = 41.64, p < .01%. Polynomial equations were further generated using multiple regression analysis. Both p values and polynomial equations were used to estimate the true effect of variables. p Values less than .0500 were considered statistically significant. Values greater than .1000 indicate the model terms are not significant. If there are many insignificant model terms (not counting those required to support hierarchy), the model reduction could have been required. The Lack of Fit F-value (0.97) was non-significant, with only a 1.25% chance that the Lack of Fit F-value this large could occur due to noise.
Furthermore, Adeq Precision measures the signal to noise ratio. In general, a ratio greater than 4 is desirable. The obtained ratio (17.910) indicated an adequate signal to navigate the design space (Kurakula et al., Citation2016). ANOVA results revealed the significant statistical relationship between the components and responses at a 95% confidence level.
Based on ANOVA results, Response (Y1) was affected significantly by the synergistic effect of liner mixture (p < .0001) (Guo et al., Citation2019; Kurakula & Naveen, Citation2020) with the highest magnitude (). The polynomial equation can be further applied to predict the response from any given concentration of independent variables. The equation was generated where lower concentrations of independent factors can contribute to the formation of globules with minimum size.
Table 7. ANOVA results in three responses.
Final Equations with Coded Factors
Globule size (Y1) = 481.52 ×1 + 110.81×2 + 260.11×3
Several studies have reported that increased oil levels can rise the globular size. This can be due to the coalescence of oil droplets and the expected decrease in the added surfactants or co-surfactants concentrations with the corresponding increase in oil percentage (Ahmed et al., Citation2018). Conversely, increasing the proportion of labrasol can reduce the globule size, due to its amphiphilic characters which enable it to decrease interfacial tension between oil droplets and surrounding aqueous media leading to smaller globule size (Naguib et al., Citation2017). RSM has been applied to analyze the effects of these selected factors. Contour plots and 3-dimensional graphs for Response I are shown in . The mixture’s three components are depicted in the corners of the triangle, and the center portion illustrates the mixture.
3.3.2. Steady-state inflow (Jss, Y2)
The model F value (219.94, p < .0001) and Lack of Fit F-value (0.7014) for Response (Y2) confirmed that the selected model is significant and the lack of fit is not significant, relative to the pure error. Adeq Precision (17.910) indicated an adequate signal to navigate the design space. Based on ANOVA results (), for the cubic model, X1, X3, X2X3, X1X2X3, X2X3 (X2−X3) factors affected Response II antagonistically at a p value <.0001, and all remaining factors showed a synergistic effect. The generated polynomial equation shows that all these selected variables significantly affected JSS. Mixture design contour plots and 3D plots were similar to those for Y1. As discussed in the previous sections, higher concentrations of surfactants and oil mixture account for elevated and decreased JSS, respectively.
Final Equations with Coded Factors
JSS (Y2) = − 12298.76 ×1 + 3087.44 ×2 − 494.73 ×3 + 17529.79 X1X + 25207.50 X1X3 − 4205.41 X2X3 − 21726.95 X1X2X3 +17752.15 X1X2 (X1−X2) + 13269.82 X1X3 (X1−X3) − 5101.24 X2X3 (X2−X3)
% permeated (Y3)
Adequate Precision for the linear model of Y3 was 17.19, which is greater than 4, suggesting that the model can navigate the design space. ANOVA results confirmed the significant effect of the linear mixture (p < .0001). The selected three components are depicted in the corners of the triangle, and 3 D contour plots were drawn to study interaction effects. The fitted linear special regression equation was generated, as shown below.
Final Equations with Coded Factors
% Permeated = 5.02 ×1 + 69.94 ×2 + +45.46 ×3
The above results showed a higher significant effect of surfactant concentration compared to other variables. As the higher surfactant proportions can reduce the globule size, this can enhance the permeation percentage (Xia et al., Citation2013; Ahmed et al., Citation2014).
Labrasol was reported to inhibit the P-glycoprotein efflux transporters, thus could increase the permeation of several drugs. Labrasol might also cause stratum corneum fluidization through opening the tight junctions, which enhances permeability. All these reasons reinforce the potential application of surfactants and oils in formulating the nanoemulsions for topical delivery (Djekic & Primorac, Citation2008; Moghimipour et al., Citation2012). Transcutol was also reported to be able to penetrate and interact with the stratum corneum to modify the permeability of several therapeutic agents. Several studies have confirmed the enhanced solubility and permeability when using transcutol as a vehicle (Vyas et al., Citation2010).
The process was optimized by setting the goals for every response and simultaneously applying the Global desirability function (D). Based on these criteria, the desirability plot was generated with a D value of 0.918 (). In conclusion, a formulation with 0.15 g oil mixture, 0.6 g of surfactant (labrasol), and 0.250 g co-surfactant (Transcutol) fulfilled the optimum formulation requirements. Using these variables can result in a formulation with a globule size of 174.79 nm, JSS-343.86, and permeation percentage of 59.45%. These predicted results were very close to the actual ones, which were 176.5 nm, 355.2 mcg/cm2.h) and 62.5% for globule size, Jss and permeation percent, respectively.
3.4. Validation of experimental design
In the end, the experimental design was further validated to confirm accuracy. Optimized formulation was prepared with the given conditions and evaluated for globule size, JSS, and percentage permeation. Relative error was calculated by comparing the predicted and practical values, as shown in . Percentage bias was within the acceptable range (±5%), thus confirming the design’s preciseness. Optimum formulation loaded with RSV showed a globule size of 142.94 nm.
Table 8. Predicted vs. Experimental values for selected responses.
3.5. In vitro release/permeation study of optimized formulation loaded with RVS
The permeability percentage through cellulose membrane for RSV and ITT was found to be 42.52 ± 2.02% and 58.66 ± 0.77% after 12 h (). JSS values further supported this finding. ITT had more JSS values, resulting in higher permeation. In general, RSV has a slow permeation, but it can exhibit and retain antioxidant and anti-inflammatory effects on the skin.
Table 9. In vitro permeation and JSS result of optimized formulation loaded with RSV.
3.6. Ex vivo permeation study of optimized formulation loaded with RVS
Ex vivo studies were conducted using simple, low head dissolution apparatus to establish the effect of ITT-RSV formulation. The permeation was studied using rat full-thickness skin, and the results are summarized in . Increased permeation percentage of ITT (60.77 ± 1.18%) from the optimized nanoemulsion formulation was observed. This can be credited to the synergistic action of labrasol and transcutol to fluidize the cell membranes and inhibit P-glycoprotein efflux. Another rationale is the high permeation of nanoemulsion drug delivery systems. Nanoemulsion can act like a drug reservoir in which the loaded drug is released into the outer phase and then into the skin. illustrated the ex vivo permeation diagram of ITT and RSV from optimized RVS-ITT-SNEDDS formulation.
Table 10. Ex vivo permeation and JSS results of optimized formulation loaded with RSV.
3.7. In vivo studies
Despite several notable advancements, hepatic safety and hepatic cell regeneration products are inadequate for treating several liver disorders. Numerous herbal extract formulations were also used in this approach (Gupta et al., Citation2010; Anantha et al., Citation2012). RSV is a natural flavonoid with exceptional antioxidant properties (Rubio-Ruiz et al., Citation2019; Yu et al., Citation2019; Daye et al., Citation2020). Significant alteration in hepato specific liquid peroxidation and oxidative stress was observed with ITT. A similar result was observed in a few previous studies (Vieira et al., Citation2012). shows the elevation of ALT and AST levels observed with formulations containing ITT alone.
Table 11. The effect of various formulation ingredients on hepatoprotective activity in comparison to marketed formulation.
Research by Vieira et al. confirmed the elevated triglyceride levels beyond the normal range in 11% of patients, increased AST in 8.36%, and increased ALT levels in 7.3% of patients. Consistent with these results, a significant rise in MDA levels, lipid peroxidation, and reduction in the antioxidant enzymes was supported by a significant decrease in the SOD activity and GSH-Px ().
The pretreatment with a formulation containing ITT + RSV for 14 days significantly increased the antioxidant property and reduced the lipid peroxidation, which reduced overall oxidative stress. All changes obtained with RSV formulation (Group 5) were more beneficial in ameliorating hepatotoxicity compared to other groups. Additionally, these two groups differed significantly from a marketed product (Group 6). No significant difference (p > .05) emerged between RSV-ITT SNEDDS formulation and the control formula for all tests. To conclude, the advanced formulation was developed relative to the marketed product in terms of hepatoprotective efficacy.
4. Conclusion
In this study, topical nanoemulsion was prepared to study the relevance of hepatoprotective agents, and utilization of nanotechnology in enhancing hepatoprotective activity. Based on solubility studies, surfactant-Labrasol, co-surfactant-Transcutol P, and essential oils (tea tree oil, and baobab oil) were selected to formulate the nanoemulsion. A low concentration of ITT favored the formation of globules with smaller particle sizes. Mixture design was successfully applied to optimize the concentrations of various parameters according to the desirability approach. Formulation with 0.15 g of oil mixture, 0.6 g of surfactant (labrasol), and 0.250 g of co-surfactant (Transcutol) achieved the optimum formulation. The range of relative error was acceptable (<5%), confirming the preciseness of the design. In vitro and ex vivo permeation studies confirmed the enhanced percentage of permeation due to the presence of labrasol and transcutol in the formulation. In vivo studies further supported these results. The hepatoprotective activity of the prepared formulation was superior compared to formulations containing individual drugs and commercially marketed products. Accordingly; RSV-ITT-SNEDDS can be considered a promising nano platform for using ITT in acne management with minimum side effects.
Supplemental Material
Download ()Acknowledgments
This research work was funded by Institutional Fund Projects under grant no. (IFPHI-158-166-2020). Therefore, authors gratefully acknowledge technical and financial support from the Ministry of Education and King Abdulaziz University, DSR, Jeddah, Saudi Arabia.
Disclosure statement
The authors report no conflicts of interest.
Additional information
Funding
References
- Abdelbary A, Salem HF, Khallaf RA, Ali AM. (2017). Mucoadhesive niosomal in situ gel for ocular tissue targeting: in vitro and in vivo evaluation of lomefloxacin hydrochloride. Pharm Dev Technol 22:409–17.
- Ahmed OA, Badr-Eldin SM, Tawfik MK, et al. (2014). Design and optimization of self-nanoemulsifying delivery system to enhance quercetin hepatoprotective activity in paracetamol-induced hepatotoxicity. J Pharm Sci 103:602–12.
- Ahmed OA, Kurakula M, Banjar ZM, et al. (2015). Quality by design coupled with near infrared in formulation of transdermal glimepiride liposomal films. J Pharm Sci 104:2062–75.
- Ahmed TA, El-Say KM, Hosny KM, Aljaeid BM. (2018). Development of optimized self-nanoemulsifying lyophilized tablets (SNELTs) to improve finasteride clinical pharmacokinetic behavior. Drug Dev Ind Pharm 44:652–61.
- Alshehri S, Altamimi M, Hussain A, et al. (2020). Morphological transition of M. tuberculosis and modulation of intestinal permeation by food grade cationic nanoemulsion: In vitro-ex vivo-in silico GastroPlus™ studies. J Drug Delivery Sci Technol 60:101971.
- Alshehri S, Imam SS, Hussain A, et al. (2020). Flufenamic acid-loaded self-nanoemulsifying drug delivery system for oral delivery: from formulation statistical optimization to preclinical anti-inflammatory assessment. J Oleo Sci 69:1257–71.
- Alvarez-Figueroa MJ, Blanco-Mendez J. (2001). Transdermal delivery of methotrexate: iontophoretic delivery from hydrogels and passive delivery from microemulsions. Int J Pharm 215:57–65.
- Anantha KC, Siva RC, Manohar RA. (2012). Hepatoprotective effect of biherbal ethanolic extract against paracetamol-induced hepatic damage in albino rats. J Ayurveda Integr Med 3:198–203.
- Aqil M, Kamran M, Ahad A, Imam SS. (2016). Development of clove oil based nanoemulsion of olmesartan for transdermal delivery: Box- Behnken design optimization and pharmacokinetic evaluation. J Mol Liq 214:238–48.
- Asai Y, Baibergenova A, Dutil M, et al. (2016). Management of acne: Canadian clinical practice guideline. CMAJ 188:118–26.
- Baxter RA. (2008). Anti-aging properties of resveratrol: review and report of a potent new antioxidant skin care formulation. J Cosmet Dermatol 7:2–7.
- Chen S, Zhao X, Ran L, et al. (2015). Resveratrol improves insulin resistance, glucose and lipid metabolism in patients with non-alcoholic fatty liver disease: a randomized controlled trial. Digestive and Liver Disease 47:226–32.
- Çinar K. (2017). A review on nanoemulsions: preparation methods and stability. Trak Univ J Eng Sci 18:73–83.
- Coimbra M, Isacchi B, van Bloois L, et al. (2011). Improving solubility and chemical stability of natural compounds for medicinal use by incorporation into liposomes. Int J Pharm 416:433–42.
- Das S, Das DK. (2007). Anti-inflammatory responses of resveratrol. Inflamm Allergy Drug Targets 6:168–73.
- Daye M, Belviranli M, Okudan N, et al. (2020). The effect of isotretinoin therapy on oxidative damage in rats. Dermatol Ther e14111. doi:10.1111/dth.14333
- de Campos VE, Ricci-Júnior E, Mansur CR. (2012). Nanoemulsions as delivery systems for lipophilic drugs. J Nanosci Nanotechnol 12:2881–90.
- De La Lastra CA, Villegas I. (2007). Resveratrol as an antioxidant and pro-oxidant agent: mechanisms and clinical implications. Biochem Soc Trans. 35:1156–60.
- Decker A, Graber EM. (2012). Over-the-counter acne treatments: a review. J Clin Aesthet Dermatol 5:32–40.
- Djekic L, Primorac M. (2008). The influence of cosurfactants and oils on the formation of pharmaceutical microemulsions based on PEG-8 caprylic/capric glycerides. Int J Pharm 352:231–9.
- Guo P, Li N, Fan L, et al. (2019). Study of penetration mechanism of labrasol on rabbit cornea by Ussing chamber, RT-PCR assay, Western blot and immunohistochemistry. Asian J Pharm Sci 14:329–39.
- Gupta C, Vikram A, Tripathi DN, et al. (2010). Antioxidant and antimutagenic effect of quercetin against DEN induced hepatotoxicity in rat. Phytother Res 24:119–28.
- Hosny KM, Aldawsari HM, Bahmdan RH, et al. (2019). El Sisi AM. Preparation, optimization, and evaluation of hyaluronic acid-based hydrogel loaded with miconazole self-nanoemulsion for the treatment of oral thrush. AAPS PharmSciTech 20:297.
- Hosny KM, Alhakamy NA, Sindi AM, Khallaf RA. (2020). Coconut oil nanoemulsion loaded with a statin hypolipidemic drug for management of burns: formulation and in vivo evaluation. Pharmaceutics 12:1061.
- Hung LM, Chen JK, Huang SS, et al. (2000). Cardioprotective effect of resveratrol, a natural antioxidant derived from grapes. Cardiovasc Res 47:549–55.
- Kala R, Tollefsbol TO, Li Y. (2012). Potential of resveratrol in inhibiting cancer and slowing aging. J Nutr Food Sci s5:001.
- Karthikeyan S, Prasad NR, Ganamani A, Balamurugan E. (2013). Anticancer activity of resveratrol-loaded gelatin nanoparticles on NCI-H460 non-small cell lung cancer cells. Biomedicine & Preventive Nutrition 3:64–73.
- Khalid M, El-Sawy HS. (2017). Polymeric nanoparticles: promising platform for drug delivery. Int J Pharm 528:675–91.
- Khallaf RA, Aboud HM, Sayed OM. (2020). Surface modified niosomes of olanzapine for brain targeting via nasal route; preparation, optimization, and in vivo evaluation. J Liposome Res 30:163–73.
- Kurakula M, Ahmed OA, Fahmy UA, Ahmed TA. (2016). Solid lipid nanoparticles for transdermal delivery of avanafil: optimization, formulation, in-vitro and ex-vivo studies. J Liposome Res 26:288–96.
- Kurakula M, El-Helw AM, Sobahi TR, Abdelaal MY. (2015). Chitosan based atorvastatin nanocrystals: effect of cationic charge on particle size, formulation stability, and in-vivo efficacy. Int J Nanomed 10:321–34.
- Kurakula M, Naveen NR. (2020). In situ gel loaded with chitosan-coated simvastatin nanoparticles: promising delivery for effective anti-proliferative activity against tongue carcinoma. Mar Drugs 18:201.
- Kurakula M, Naveen NR. (2020). Prospection of recent chitosan biomedical trends: evidence from patent analysis (2009–2020. Int J Biol Macromol 165:1924–38.
- Layton A. (2009). The use of isotretinoin in acne. Dermatoendocrinol 1:162–9.
- Malhi HK, Tu J, Riley TV, et al. (2017). Tea tree oil gel for mild to moderate acne; a 12 week uncontrolled, open‐label phase II pilot study. Australas J Dermatol 58:205–10.
- Moghimipour E, Salimi A, Leis F. (2012). Preparation and evaluation of tretinoin microemulsion based on pseudo-ternary phase diagram. Adv Pharm Bull 2:141–7.
- Naguib SS, Hathout RM, Mansour S. (2017). Optimizing novel penetration enhancing hybridized vesicles for augmenting the in-vivo effect of an anti-glaucoma drug. Drug Deliv 24:99–108.
- Naveen NR, Kurakula M, Gowthami B. (2020). Process optimization by response surface methodology for preparation and evaluation of methotrexate loaded chitosan nanoparticles. Mater Today Proc.
- Nelson AM, Gilliland KL, Cong Z, Thiboutot DM. (2006). 13-cis Retinoic acid induces apoptosis and cell cycle arrest in human SEB-1 sebocytes. J Invest Dermatol 126:2178–89.
- Osborne DW, Musakhanian J. (2018). Skin penetration and permeation properties of Transcutol®-Neat or Diluted Mixtures. AAPS Pharmscitech 19:3512–33.
- Pathania R, Khan H, Kaushik R, Khan MA. (2018). Essential oil nanoemulsions and their antimicrobial and food applications. Curr Res Nutr Food Sci 6:626–43.
- Peiyuan H, Zhiping H, Chengjun S, et al. (2017). Resveratrol ameliorates experimental alcoholic liver disease by modulating oxidative stress. Evid Based Complement Alternat Med 2017:4287890.
- Planas JM, Alfaras I, Colom H, Juan ME. (2012). The bioavailability and distribution of trans-resveratrol are constrained by ABC transporters. Arch Biochem Biophys 527:67–73.
- Rauf A, Imran M, Butt MS, et al. (2018). Resveratrol as an anti-cancer agent: A review. Crit Rev Food Sci Nutr 58:1428–47.
- Rubio-Ruiz ME, Guarner-Lans V, Cano-Martínez A, et al. (2019). Resveratrol and quercetin administration improves antioxidant defenses and reduces fatty liver in metabolic syndrome rats. Molecules 24:1297.
- Safwat S, Hathout RM, Ishak RA, Mortada ND. (2017). Augmented simvastatin cytotoxicity using optimized lipid nanocapsules: a potential for breast cancer treatment. J Liposome Res 27:1–10.
- Salem HF, El-Menshawe SF, Khallaf RA, Rabea YK. (2020). A novel transdermal nanoethosomal gel of lercanidipine HCl for treatment of hypertension: optimization using Box-Benkhen design, in vitro and in vivo characterization. Drug Deliv Transl Res 10:227–40.
- Sarker D. (2005). Engineering of nanoemulsions for drug delivery. Curr Drug Deliv 2:297–310.
- Satalkar P, Elger BS, Hunziker P, Shaw D. (2016). Challenges of clinical translation in nanomedicine: a qualitative study. Nanomedicine 12:893–900.
- Shafiq S, Shakeel F, Talegaonkar S, et al. (2007). Development and bioavailability assessment of ramipril nanoemulsion formulation. Eur J Pharm Biopharm 66:227–43.
- Teskač K, Kristl J. (2010). The evidence for solid lipid nanoparticles mediated cell uptake of resveratrol. Int J Pharm 390:61–9.
- Vargas JE, Souto AA, Pitrez PM, et al. (2016). Modulatory potential of resveratrol during lung inflammatory disease. Med Hypotheses 96:61–5.
- Venkatesh M, Mallesh K. (2013). Self-nano emulsifying drug delivery system (SNEDDS) for oral delivery of atorvastatin-formulation and bioavailability studies. J. Drug Delivery Ther 3:131–40.
- Vieira AS, Beijamini V, Melchiors AC. (2012). The effect of isotretinoin on triglycerides and liver aminotransferases. Anais Brasileiros de Dermatologia 87:382–7.
- Vyas A, Saraf S, Saraf S. (2010). Encapsulation of cyclodextrin complexed simvastatin in chitosan nanocarriers: a novel technique for oral delivery. J Incl Phenom Macrocycl Chem 66:251–9.
- Wren S, Stucki A. (2003). Organic essential oils, indigenous cold pressed oils, herbs and spices in Sub-Saharan Africa. Int J Aromather 13:71–81.
- Wu Y, Li YH, Gao XH, Chen HD. (2013). The application of nanoemulsion in dermatology: an overview. J Drug Target 21:321–7.
- Xia HJ, Zhang ZJX, Hu Q, et al. (2013). A novel drug–phospholipid complex enriched with micelles: preparation and evaluation in vitro and in vivo. Int J Nanomed 8:545–54.
- Yehia R, Hathout RM, Attia DA, et al. (2017). Anti-tumor efficacy of an integrated methyl dihydrojasmonate transdermal microemulsion system targeting breast cancer cells: In vitro and in vivo studies. Colloids Surf B Biointerfaces 155:512–21.
- Yu S, Zhou X, Xiang H, et al. (2019). Resveratrol reduced liver damage after liver resection in a rat model by upregulating sirtuin 1 (SIRT1) and inhibiting the acetylation of high mobility group box 1 (HMGB1). Med Sci Monit 25:3212–20.