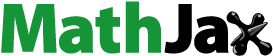
Abstract
Full thickness cutaneous wound therapy and regeneration remains a critical challenge in clinical therapeutics. Recent reports have suggested that mesenchymal stem cells exosomes therapy is a promising technology with great potential to efficiently promote tissue regeneration. Multifunctional hydrogel composed of both synthetic materials and natural materials is an effective carrier for exosomes loading. Herein, we constructed a biodegradable, dual-sensitive hydrogel encapsulated human umbilical cord-mesenchymal stem cells (hUCMSCs) derived exosomes to facilitate wound healing and skin regeneration process. The materials characterization, exosomes identification, and in vivo full-thickness cutaneous wound healing effect of the hydrogels were performed and evaluated. The in vivo results demonstrated the exosomes loaded hydrogel had significantly improved wound closure, re-epithelialization rates, collagen deposition in the wound sites. More skin appendages were observed in exosomes loaded hydrogel treated wound, indicating the potential to achieve complete skin regeneration. This study provides a new access for complete cutaneous wound regeneration via a genipin crosslinked dual-sensitive hydrogel loading hUCMSCs derived exosomes.
1. Introduction
Skin tissue is the largest organ of human body, which possesses several key functions including protective barrier effect against pathogeny and mechanical stress, body thermoregulation as well as sense of touch, pressure, vibration, and pain (Liu et al., Citation2018; Newberry et al., Citation2018). Skin tissue defects were often caused by trauma, burns, surgery, or diabetic complications. Cutaneous wound healing is a complex process of injured skin regeneration involving a series of coordinated phases, including inflammatory, proliferative, and remodeling phases (Kasuya & Tokura, Citation2014; Ferreira & Gomes, Citation2018). In recent years, direct transplanting stem cells for tissue regeneration therapy has been extensively researched and revealed the positive effects (Li et al., Citation2017; Wang et al., Citation2017; Zhang et al., Citation2017, Citation2018; Hsu et al., Citation2019). However, such application method could cause complications such as secretion of pro-inflammatory molecules, nonspecific cell differentiation, and immune protective responses from the transplanted stem cells (Alizadeh et al., Citation2019a, Citation2019b; Simorgh et al., Citation2019; Shafei et al., Citation2020). Meanwhile, emerging research suggests the therapeutic effects of stem cells are more notably dependent on paracrine pathway signals, particularly exosomes (Lai et al., Citation2015; Ha et al., Citation2020). Exosomes are small membrane vesicles ranging from 30 to 150 nm in diameter which contain complex RNA, proteins, and endogenous factors (Tricarico et al., Citation2017). As one of the most important products secreted by cells, exosomes could mediate intercellular communication and facilitate wound closure and tissue regeneration (Shabbir et al., Citation2015; Cooper et al., Citation2018; Fitzsimmons et al., Citation2018). Recent researches have demonstrated that exosomes could significantly accelerate cell proliferation, migration, and angiogenesis as well as collagen deposition around the wound area (Shabbir et al., Citation2015; Zhang et al., Citation2015; Liang et al., Citation2016). Such phenomena were contributed to the available paracrine signaling and complex inclusions in the exosomes stimulate the recipient cells which regulate the cell behaviors (Shabbir et al., Citation2015; Fitzsimmons et al., Citation2018; Wang et al., Citation2019; Nooshabadi et al., Citation2020). For example, it was verified that prompting angiogenesis appeared in a rat skin wound model due to human umbilical cord MSCs derived exosomes induced β-catenin activation by transferring Wnt-4 (Zhang et al., Citation2015). Different signaling pathways could be activated as exosomes arrived target cells to promote the expression of various growth factors including STAT3, interleukin-6 (IL-6), stromal cell-derived factor-1 (SDF-1), insulin-like growth factor-1 (IGF-1), and HGF in the wound healing process (Rani & Ritter, Citation2016). These studies imply that MSCs derived exosomes could be a more promising therapy for cutaneous wound healing compared to direct stem cells transplanting.
Wound dressing has significant promoting effects on wound healing process. The disadvantages of traditional medical cotton gauze are frequently replacement, wound adhesive, and vulnerable to second trauma. In comparison, hydrogel is capable to cover and protect cutaneous and avoid second trauma during dressing change. Hydrogels are physical or chemical cross-linked three-dimensional hydrophilic polymeric networks, which possess the capacity to absorb abundant amount of water in aqueous solution without going through the dissolution process (Leijten et al., Citation2017; Kazeminava et al., Citation2018; Soleymani et al., Citation2019). The properties of wound dressing hydrogel such as good adhesive capacity with skin, biocompatibility, tissue-like characteristics have attracted enormous attention in the tissue regeneration and biomedicine field (Trkov et al., Citation2010). Hydrogel is versatile to serve as protective barrier, tissue scaffold, drug loading carrier, and exosomes encapsulation matrix in clinical application. The incorporated active pharmaceutical ingredient or bioactive factors in the hydrogel could retain more longer stability and achieve sustained release effect, comparing to the direct administration method (Rice et al., Citation2013). All these characteristics made hydrogel a practical access to carry large number of exosomes and apply on the wound surface (Zhou et al., Citation2013; Li et al., Citation2016; Tao et al., Citation2017). In the last years, various kinds of hydrogels composed of biomaterial and synthetic material were investigated for exosomes delivery in tissue regeneration. For instance, Shi et al. prepared a chitosan/silk composite hydrogel matrix with both biodegradable and biocompatible properties; the freeze-dried hydrogel matrix loaded human gingival MSC-derived exosomes accelerated wound healing in a diabetic rat skin defect mode (Shi et al., Citation2017). Zhang et al. prepared a thermosensitive chitosan-based hydrogel which encapsulated human placenta MSCs-derived exosomes extracted by centrifugation method. This study suggested that chitosan-based hydrogel significantly promoted the stability of exosomal inclusions such as micro-RNAs and proteins as well as the half-life of exosomes in vivo. Meanwhile, the firefly luciferase imaging of angiogenesis implied angiogenesis was induced by exosomes loaded in hydrogels under both in vitro and in vivo conditions (Zhang et al., Citation2018). Wang et al. invented a novel injectable self-healing polypeptide-based hydrogel which possessed inherent antibacterial activity and pH-responsive sustained adipose-derived MSCs-derived exosomes release profile. The immunohistochemical results revealed more skin appendages and less scar tissue appeared in the exosomes loaded hydrogel treated diabetic full-thickness cutaneous wound group (Wang et al., Citation2019).
The hydrogels encapsulated various kinds of MSCs-derived exosomes therapy method representing a promising access to clinical cutaneous wound healing treatment. However, to the best of our known, there was few studies of human umbilical cord MSCs-derived exosomes encapsulated in hydrogels applied in skin wound healing therapy.
In this study, exosomes were isolated from human umbilical MSCs culture supernatant via ultracentrifugation method. The obtained exosomes were identified by transmission electron microscope (TEM), laser particle size distribution (LPSD) measurement, and western blot (WB). Thermo and pH-sensitive hydrogel composed of poloxamer 407 (P407) and chitosan derivate carboxymethyl chitosan (CMCS) was synthesized by crosslinking reaction with a natural nontoxic crosslinker genipin (GP), and the characterization of hydrogel was analyzed. The dual-sensitive hydrogel possessed low toxicity properties on human umbilical cord-mesenchymal stem cells (hUCMSCs) cells. The characteristics of good liquidity and rapid in situ gelation function improved wound shape adaptation. Proper viscosity property of the hydrogel ensured the capability of wound tissue adhesion and long-lasting protective layer forming. Fine lattice internal micro structure of hydrogel promoted water absorption, air penetration and maintained wound site moist. High degradation rate guaranteed low residual amount of covering material and convenience of dressing change without second trauma. In addition to the above characteristics, the hydrogel provided in vitro sustain release profiles of therapeutic active factors as a suitable drug delivery carrier. Thus, hUCMSCs derived exosomes encapsulated in the hydrogel were applied on the cutaneous wound in rat model to explore the effect of skin tissue regeneration (). Histological method including HE staining and Masson-trichrome staining was performed to investigate the wound closure rates, re-epithelialization, and collagen deposition effects. The immunohistochemical staining of TNF-α and IL-1β was performed to investigate the anti-inflammatory action of exosomes. All the data demonstrated the dual-sensitive hydrogel loading exosomes could promote wound healing process and reduce inflammatory response than other treatment methods.
2. Materials and methods
2.1. Materials
Carboxymethyl chitosan (viscosity: 100–200 mPa.s, deacetylation degree ≥95%) was purchased from Shanghai Macklin Biochemical Co., Ltd. (Shanghai, China); P407 was purchased from Beijing Solarbio Science & Technology Co., Ltd. (Beijing, China); genipin was purchased from Beijing Ouhe Technology Co. Ltd. (Beijing, China); the fresh hUCMSCs culture supernatant was kindly donated by Shenyang Kangwei Medical Laboratory Co., Ltd. (Shenyang, China); purified anti-human CD9 antibody (RenGen Biosciences, Shenyang, China); purified anti-human CD63 antibody (RenGen Biosciences, Shenyang, China); purified anti-human Alix antibody (RenGen Biosciences, Shenyang, China); goat anti-mouse IgG (H + L) HRP conjugate (Invitrogen, Carlsbad, CA); nitrocellulose blotting membrane (GE Healthcare, Chicago, IL); all other reagents used in the study were analytical grade or higher.
2.2. Isolation and identification of hUCMSCs derived exosomes
The WX + ultracentrifuge (Thermo Scientific, Waltham, MA) instrument was employed to isolate and purify exosomes from the hUCMSCs culture supernatant. The operation procedures were acted at 4 °C as follows: first, the culture supernatant was centrifuged under 300 G in 10 min to remove cells; then, the supernatant was centrifuged under 2000 G in 10 min to remove dead cells; after that, the supernatant was centrifuged under 10,000 G in 30 min to remove cell debris; the clarified supernatant was ultracentrifuged under 100,000 G in 70 min to obtain pellets composed of exosomes and impure proteins, the pellets were washed and resuspended in PBS and then ultracentrifuged at the same condition. The finally isolated pellets were objective pure exosomes and stored at −80 °C.
The surface characteristic markers of hUCMSCs derived exosomes were detected via WB method, including CD9, CD63, and Alix. The morphology of the exosomes was detected by a Hitachi H-7650 electronic TEM (Tokyo, Japan). Five microliters of exosomes PBS diluted sample was pipetted on an amorphous carbon film coated copper grid, then stained with 2% phosphotungstic acid (pH 6.8) and incubated 30 min at room temperature. The images of samples were taken under 80 kV working voltage. The particle size distribution of exosomes was measured by a Zetasizer Pro (Malvern Instruments Ltd., Malvern, UK) at 25 °C.
2.3. Preparation of thermo and pH-sensitive hydrogel
CMCS (30 mg) were dissolved in 1 ml saline under magnetic stirring at 37 °C to form a transparent solution. Then, P407 (180 mg) was added into the solution as thermo sensitive agent, and the solution was stirred for several minutes until the polymers totally dissolved. Crosslink agent GP (0.2 mg) was added into the solution to induce the synthesize reaction with CMCS, the reaction was performed under 50 rpm magnetic stirring at 37 °C for 2 h to formulate a viscous solution. Finally, the above isolated exosomes (20 μg) were resuspended in 50 μl PBS and mixed into the solution under stir at 4 °C to form a homogeneous hydrogel.
2.4. Characterization of thermo and pH-sensitive hydrogel
The pure hydrogel was lyophilized to get a loose and porous stent; the microstructure of hydrogel stent surface was analyzed by scanning electron microscopy (SEM, HITACHI, SU3500, Tokyo, Japan). To investigate the degradation rate of the hydrogel, the lyophilized hydrogel stent was immersed in pH 6.5 PBS buffer for 24 h at 35 °C to reach swelling equilibrium status. The predetermined amount of completely expanded hydrogels was incubated into the pH 6.5 at 35 °C PBS culture medium for 240 h. At each sampling time point, the hydrogels were taken out from culture medium, the excess water on the surface of the hydrogels was carefully wiped out with paper towel before weighing. The degradation rate was calculated according to the following equation:
In this equation, W0 and Wt represent the weights of expanded hydrogels before and after incubating in culture medium, respectively.
2.5. In vitro dialysis dissolution test
The in vitro release profile of hydrogel encapsulated exosomes was tested via dynamic dialysis method. The molecule weight of dialysis membrane was between 8000 and 12,000 relative molecular mass. Then, 1 ml above prepared hydrogel contained 20 μg exosomes was pipetted into the dialysis bag. The dialysis bag was immersed and incubated into 10 ml PBS dissolution medium of pH 6.5, working temperature was set at set at 35 ± 0.5 °C. Periodically, same amount of preheated dissolution medium was replenished following the collection of 1 ml sample. The quantitative determination of the released exosomes was performed by a micro BCA protein assay kit (Beyotime, Shanghai, China).
2.6. Rat cutaneous wound modeling
Twelve specified pathogen free (SPF) grade female SD rats (4–5 weeks old) were purchased from Beijing Hua Fu Kang Biotechnology Co. Ltd. (Beijing, China) and Department of Laboratory Animal Science of China Medical University (ethics review approval no. CMU2020169). The experimental animals were preliminary narcotized by enflurane gas, and then totally narcotized via intraperitoneal injection of pentobarbital sodium (30 mg/kg). The dorsal hair of the rats was shaved and the back skin was disinfected before operation. The operation of wound modeling was implemented to create four round shaped full-thickness dermal defects with 8 mm diameter. The wounds of each rat were classified as one negative control group treated with saline (control group), one pure hydrogel treatment group (300 μl hydrogel covered the wound), and two exosomes loaded treatment groups (300 μl hydrogel containing 6 μg exosomes covered the wound). Then, the paraffin bandages were used to fix the wounds and the dressings. The wound dressings were exchanged every three days. All operated rats were reared in separated cages in an SPF level animal laboratory. The macroscopic wound healing effect was studied by taking photographs of wounds with cell phone digital camera on 0-, 4-, 7- and 14-days post-surgery. The wound healing ratio was expressed as the percentage of the reduction wound area. Image J software was employed to measure the remained wound area and calculate the wound healing ratio (Bethesda, MD).
2.7. Histopathological staining
Six rats were humanely sacrificed at the end of 7th and 14th day post-surgery, respectively. The wound samples were harvested, fixed in 10% (v/v) paraformaldehyde, and embedded in paraffin. Wound site tissue cross sections (5 μm) were mounted on slides for histopathological investigation. Standard protocol of hematoxylin–eosin (H&E) was performed on the slices to investigate the wound healing process. Masson’s trichrome (MT) staining was performed to measure the collagen fiber deposition in the wound site. Immunohistochemistry (IHC) including TNF-α, IL-1β was performed to investigate the influence on inflammatory reaction.
The stained slices were observed and photographed by an optical microscope (Eclipse 80i, Nikon, Tokyo, Japan) with a digital camera (DS-Ri2, Nikon, Tokyo, Japan). Image J with IHC profiler plugins was used to analyze the expression amounts of the markers (Bethesda, MD).
2.8. Statistical analysis
Data from at least three individual experiments are listed as mean ± standard deviation (mean ± SD). One-way analysis of variance was performed to distinguish the significant difference.
3. Results
3.1. Exosomes characterization
The isolated and purified exosomes derived from hUCMSCs were identified by TEM, laser size detector, and WB method (). As shown in , the isolated exosomes revealed spherical-like morphology during the TEM tests. The average particle size of exosomes was 60.1 ± 4.7 in accordance with the TEM results, and the polydispersity index (PDI) was 0.279 revealing relatively uniform distribution of exosomes particle size. The diameters of isolated exosomes ranged approximately from 30 to 150 nm and were consistent with previously reported results of size and shape from other cell lines (Li et al., Citation2017). Meanwhile, WB analysis demonstrated high expression of CD9 and CD63 and slightly expression of Alix (). The results indicated that exosomes were successfully isolated from hUCMSCs and consistent with the reports (Wang et al., Citation2016; Yim et al., Citation2016).
3.2. Hydrogel property
The thermo and pH-sensitive property and viscosity of the hydrogel were demonstrated in our previous published study (https://doi.org/10.1080/21691401.2021.1879104). The morphology of the lyophilized hydrogel stent cross-section was detected by SEM and is shown in . The lyophilized hydrogel possessed fine lattice structure with pore sizes ranging between 100 and 200 μm, this porosity property of the hydrogel could improve drug loading rate and facilitate drug release behavior in clinical application.
Biodegradation rate is a key factor of hydrogel applied as tissue engineering scaffold in the therapy of skin impair, since the scaffold could enable cell proliferation and extracellular matrix production in healing process (Gantwerker & Hom, Citation2012; Aderibigbe & Buyana, Citation2018). The determination of hydrogel biodegradation rate was performed in 240 h and the results are demonstrated in . The hydrogels incubated in PBS culture medium gradually degraded in 240 h and the degradation rate increased after 72 h. Moreover, the dissolution profiles revealed in demonstrated the loaded exosomes sustained released from the hydrogel and reached over 85% cumulative release amount after 72 h.
3.3. In vivo wound healing study
presents the macroscopic healing statue of the full cutaneous skin impair sites which treated with saline (control), pure hydrogel, and exosomes loaded hydrogel. Visual observation of wound sites in mice showed there was no infection phenomenon occurred during the experiment. All the treated wounds achieved a remarkable decrease in wound size during the experiment, while the control group revealed less wound closure rate at 4 and 7 days compared to the treated groups. The hydrogel and EXO group possessed the best wound closure speed and the most efficient healing effect with almost complete closure of the wound at 14 days. Consistent with the visual observation, the quantitative wound closure rates ( calculated by Image J (Bethesda, MD) showed that the hydrogel and EXO group revealed faster wound healing speed than other two groups at all the recording time points with 98.90 ± 1.2% closure rate on day 14, meanwhile, other two groups achieved 74.76 ± 4.3% (control) and 86.87 ± 3.6% (hydrogel) final healing rate, respectively. The pure hydrogel group also exhibited better wound healing promotion effect compared to saline, due to suitable moisture retention ability and unique biocompatibility could promote wound healing process by facilitating re-epithelialization, indicating the potential of the hydrogel as wound cover material (Hu et al., Citation2016; Atoufi et al., Citation2019). Moreover, several previous studies demonstrated that the therapy of exosomes loaded in hydrogel could active trophic signal pathways, affecting cell migration, proliferation, and angiogenesis in wound healing process (Shi et al., Citation2015; Zhang et al., Citation2015; Khanmohammadi et al., Citation2017).
3.4. Histological evaluation of wound site
During the wound healing process, the stem cells around the wound site could be induced by the exosomes by paracrine signaling pathways to proliferate and differentiate to repair the tissue impair, angiogenesis, and re-epithelialization would occur in the meantime. H&E staining results are shown in , the results illustrated that the control group formed few neo epidermis on 7th day, meanwhile, more granulation tissue and epidermis-like cell arrangement formed in the wound sites of both hydrogel and hydrogel and EXO groups. At day 14, skin appendages could be clearly observed in both hydrogel and hydrogel and EXO groups, the hydrogel group possesses significantly higher number of dermal appendages than other two groups in the wound sites, indicating the exosomes prominently facilitated the wound healing effect on skin wound repair and regeneration.
Figure 7. Histological analysis of the wounds in different experimental conditions. (A) H&E staining microscopic images of healing wound sites. (B) MT staining of collagens in wound sites. Scale bar: 100 μm.

Neo-collagen deposition is capable to provide habitable microenvironment for cell proliferation, migration and differentiation, which possess significant influence on tissue regeneration (Chang et al., Citation2013). MT staining results shown in indicate that more collagen deposition occurred in the hydrogel and EXO group on 14th day, the newly formed collagen fibers were relatively well-organized. Compared to the treated groups, the collagen deposition in control group revealed a relative low expression quantity and could be hardly seen on 14th day. In addition, more skin appendages could be observed in the hydrogel and EXO group and few skin appendages in hydrogel group, outcome was consistent with the H&E staining results.
The immunohistochemical staining of TNF-α and IL-1β, which were biomarker associated with inflammation reaction, was performed to investigate the anti-inflammatory and immunoregulation of the exosomes. The main function of TNF regulates the functions of immune cells. The inflammation reactions including fever, apoptotic cell death, cachexia could be triggered by TNF; moreover, TNF could respond to sepsis via IL-1 and IL-6 producing cells (Xu et al., Citation2019). As shown in , the hydrogel and EXO group revealed the lowest expression quantity of TNF-α and IL-1β at 7th and 14th day compared to other groups. The hydrogel group revealed slightly lower expression of TNF-α than control group, however, the expression of IL-1β was almost the same as control group at 7th and 14th day, indicating the hydrogel hardly possessed anti-inflammation effect. Thus, the results demonstrated the exosomes loaded in hydrogel could inhibit inflammatory reactions in healing process.
Figure 8. Histochemical analysis of TNF-α and IL-1β expression in wound sites. (A, C) Immunohistochemistry staining images for TNF-α and IL-1β at 7 and 14 days post operation, respectively. Scale bar: 50 μm. (B, D) Quantitative analysis of relative density of TNF-α and IL-1β at 7 and 14 days after surgery, respectively.

4. Discussion
The key influence factors of wound healing therapy could be attributed to wound dressing and effective therapeutic active factors. Multi-functional wound dressings promoting wound healing process were studied and developed in recent years and have attracted a lot of attention.
Zhao et al. developed a kind of physical double-network self-healing antibacterial and NIR/pH stimulus sensitive hydrogel, which could prevent multidrug-resistant bacterial infection and promote wound closure and wound healing process (Zhao et al., Citation2020). Li et al. first invented and reported a series of antioxidant conductive injectable self-healing hydrogels, aiming to synchronize biomechanical and biochemical activity of the hydrogels for wound healing acceleration effect (Li et al., Citation2020). Furthermore, the synergistic effect of multi-functional hydrogel and therapeutic active factors is a fascinating approach for full-thickness wounds therapy (Shi et al., Citation2017; Qu et al., Citation2018; Liang et al., Citation2019). Mesenchymal-derived EXOs were effective therapeutic active factors for promoting wound healing process, due to the ability to activate signaling pathways related to promotion of cell migration, vascularization, collagen deposition, and inflammatory response (Rosca et al., Citation2018; Nooshabadi et al., Citation2020). However, the method of direct injection to the wound may suffer exosomes loss and escape leading to a consequent decrease in efficacy. Thus, it is expected that the combination of functional hydrogel and exosomes could avert potential safety risks in direct stem cell injection and improve wound healing therapy efficiency.
In our study, we have introduced the GP crosslinked hydrogel as scaffold for wound healing process, the hydrogel presented biodegrade property and capable for exosomes loading and sustained release behavior. The hydrogel showed good wound protection effect and moisture retention function without stimulating or proinflammatory effects on wound. The biodegradability of the hydrogel revealed the potential for in vivo injection application, which is consistent with the previous studies reporting the promising method of introducing synthetic material into natural material for in vivo biodegradable tissue engineering scaffold (Kaisang et al., Citation2017; Xu et al., Citation2018). Furthermore, the exosomes released from the hydrogel significantly improve the wound healing effect on 4th day as shown in , this phenomenon not only proved the therapeutical function of hUCMSCs derived exosomes, but also validated the relevance between in vivo and in vitro release behavior of exosomes from the hydrogel. This cell-free therapeutic method for skin wound healing possesses great advantages compared with direct application of hUCMSCs, due to the side effects including secretion of proinflammatory molecules, undesired cell differentiation and immune-protection responses of planted stem cells (Alizadeh et al., Citation2019b; Shafei et al., Citation2020). The exosomes significantly improved wound healing and tissue regeneration, reduced inflammatory response during the experiment. Such efforts could be attributed to the abundant amount of growth factors such as VEGF, bFGF, and TGFB1 contained in the exosomes, which have been proved to support the angiogenesis potential for cells (Cooper et al., Citation2018). Thus, these results proved the combination of exosomes and hydrogel could enhance skin wound healing and tissue regeneration in full thickness cutaneous wound therapy.
5. Conclusions
In this study, we demonstrated the feasibility of GP crosslinked hydrogel for exosomes encapsulation and full thickness cutaneous impair therapy. The isolated hUCMSCs derived exosomes could release from the hydrogel as well as improve wound healing and tissue regeneration of skin appendages in the meantime. The combined treatment method contributed to accelerated cell proliferation and migration, neo collagen deposition and alleviative inflammatory responses. These results indicated a promising way to treat acute skin wound.
Disclosure statement
The authors declare no conflict of interest.
Additional information
Funding
References
- Aderibigbe BA, Buyana B. (2018). Alginate in wound dressings. Pharmaceutics 10:42.
- Alizadeh R, Bagher Z, Kamrava SK, et al. (2019a). Differentiation of human mesenchymal stem cells (MSC) to dopaminergic neurons: a comparison between Wharton's Jelly and olfactory mucosa as sources of MSCs. J Chem Neuroanat 96:126–33.
- Alizadeh R, Zarrintaj P, Kamrava SK, et al. (2019b). Conductive hydrogels based on agarose/alginate/chitosan for neural disorder therapy. Carbohydr Polym 224:115161.
- Atoufi Z, Kamrava SK, Davachi SM, et al. (2019). Injectable PNIPAM/hyaluronic acid hydrogels containing multipurpose modified particles for cartilage tissue engineering: synthesis, characterization, drug release and cell culture study. Int J Biol Macromol 139:1168–81.
- Chang J, DeLillo N, Khan M, Nacinovich M. (2013). Review of small intestine submucosa extracellular matrix technology in multiple difficult-to-treat wound types. Wounds 25:113–20.
- Cooper D, Wang C, Patel R, et al. (2018). Human adipose-derived stem cell conditioned media and exosomes containing MALAT1 promote human dermal fibroblast migration and ischemic wound healing. Adv Wound Care (New Rochelle) 7:299–308.
- Ferreira ADF, Gomes DA. (2018). Stem cell extracellular vesicles in skin repair. Bioengineering (Basel) 6:4.
- Fitzsimmons REB, Mazurek MS, Soos A, Simmons CA. (2018). Mesenchymal stromal/stem cells in regenerative medicine and tissue engineering. Stem Cells Int 2018:8031718.
- Gantwerker EA, Hom DB. (2012). Skin: histology and physiology of wound healing. Clin Plast Surg 39:85–97.
- Ha DH, Kim HK, Lee J, et al. (2020). Mesenchymal stem/stromal cell-derived exosomes for immunomodulatory therapeutics and skin regeneration. Cells 9:1157.
- Hsu L-C, Peng B-Y, Chen M-S, et al. (2019). The potential of the stem cells composite hydrogel wound dressings for promoting wound healing and skin regeneration: in vitro and in vivo evaluation. J Biomed Mater Res B Appl Biomater 107:278–85.
- Hu L, Wang J, Zhou X, et al. (2016). Exosomes derived from human adipose mesenchymal stem cells accelerates cutaneous wound healing via optimizing the characteristics of fibroblasts. Sci Rep 6:32993.
- Kaisang L, Siyu W, Lijun F, et al. (2017). Adipose-derived stem cells seeded in Pluronic F-127 hydrogel promotes diabetic wound healing. J Surg Res 217:63–74.
- Kasuya A, Tokura Y. (2014). Attempts to accelerate wound healing. J Dermatol Sci 76:169–72.
- Kazeminava F, Arsalani N, Akbari A. (2018). POSS nanocrosslinked poly (ethylene glycol) hydrogel as hybrid material support for silver nanocatalyst. Appl Organometal Chem 32:e4359.
- Khanmohammadi M, Sakai S, Taya M. (2017). Impact of immobilizing of low molecular weight hyaluronic acid within gelatin-based hydrogel through enzymatic reaction on behavior of enclosed endothelial cells. Int J Biol Macromol 97:308–16.
- Lai RC, Yeo RWY, Lim SK. (2015). Mesenchymal stem cell exosomes. Semin Cell Dev Biol 40:82–8.
- Leijten J, Seo J, Yue K, et al. (2017). Spatially and temporally controlled hydrogels for tissue engineering. Mater Sci Eng R Rep 119:1–35.
- Li D, Hu N, Yu Y, et al. (2017). Trajectories of multidimensional caregiver burden in Chinese informal caregivers for dementia: evidence from exploratory and confirmatory factor analysis of the Zarit Burden Interview. J Alzheimers Dis 59:1317–25.
- Li M, Liang Y, He J, et al. (2020). Two-pronged strategy of biomechanically active and biochemically multifunctional hydrogel wound dressing to accelerate wound closure and wound healing. Chem Mater 32:9937–53.
- Li M, Tao S-C, Ke Q-F, et al. (2016). Fabrication of hydroxyapatite/chitosan composite hydrogels loaded with exosomes derived from miR-126-3p overexpressed synovium mesenchymal stem cells for diabetic chronic wound healing. J Mater Chem B 4:6830–41.
- Li P, Kaslan M, Lee SH, et al. (2017). Progress in exosome isolation techniques. Theranostics 7:789–804.
- Liang X, Zhang L, Wang S, et al. (2016). Exosomes secreted by mesenchymal stem cells promote endothelial cell angiogenesis by transferring miR-125a. J Cell Sci 129:2182–9.
- Liang Y, Zhao X, Hu T, et al. (2019). Adhesive hemostatic conducting injectable composite hydrogels with sustained drug release and photothermal antibacterial activity to promote full-thickness skin regeneration during wound healing. Small 15:e1900046.
- Liu H, Wang C, Li C, et al. (2018). A functional chitosan-based hydrogel as a wound dressing and drug delivery system in the treatment of wound healing. RSC Adv 8:7533–49.
- Newberry CI, Thomas JR, Cerrati EW. (2018). Facial scar improvement procedures. Facial Plast Surg 34:448–57.
- Nooshabadi VT, Khanmohamadi M, Valipour E, et al. (2020). Impact of exosome-loaded chitosan hydrogel in wound repair and layered dermal reconstitution in mice animal model. J Biomed Mater Res A 108:2138–49.
- Qu J, Zhao X, Liang Y, et al. (2018). Antibacterial adhesive injectable hydrogels with rapid self-healing, extensibility and compressibility as wound dressing for joints skin wound healing. Biomaterials 183:185–99.
- Rani S, Ritter T. (2016). The exosome – a naturally secreted nanoparticle and its application to wound healing. Adv Mater 28:5542–52.
- Rice JJ, Martino MM, De Laporte L, et al. (2013). Engineering the regenerative microenvironment with biomaterials. Adv Healthc Mater 2:57–71.
- Rosca A, Tutuianu R, Titorencu I. (2018). Mesenchymal stromal cells derived exosomes as tools for chronic wound healing therapy. Roman J Morphol Embryol 59:655–662.
- Shabbir A, Cox A, Rodriguez-Menocal L, et al. (2015). Mesenchymal stem cell exosomes induce proliferation and migration of normal and chronic wound fibroblasts, and enhance angiogenesis in vitro. Stem Cells Dev 24:1635–47.
- Shafei S, Khanmohammadi M, Heidari R, et al. (2020). Exosome loaded alginate hydrogel promotes tissue regeneration in full-thickness skin wounds: an in vivo study. J Biomed Mater Res A 108:545–56.
- Shi Q, Qian Z, Liu D, et al. (2017). GMSC-derived exosomes combined with a chitosan/silk hydrogel sponge accelerates wound healing in a diabetic rat skin defect model. Front Physiol 8:904.
- Shi Y, Shu B, Yang R, et al. (2015). Wnt and Notch signaling pathway involved in wound healing by targeting c-Myc and Hes1 separately. Stem Cell Res Ther 6:120.
- Simorgh S, Alizadeh R, Eftekharzadeh M, et al. (2019). Olfactory mucosa stem cells: an available candidate for the treatment of the Parkinson's disease. J Cell Physiol 234:23763–73.
- Soleymani M, Akbari A, Mahdavinia G. (2019). Magnetic PVA/laponite RD hydrogel nanocomposites for adsorption of model protein BSA. Polym Bull 76:2321–40.
- Tao S-C, Guo S-C, Li M, et al. (2017). Chitosan wound dressings incorporating exosomes derived from microRNA-126-overexpressing synovium mesenchymal stem cells provide sustained release of exosomes and heal full-thickness skin defects in a diabetic rat model. Stem Cells Transl Med 6:736–47.
- Tricarico C, Clancy J, D'Souza-Schorey C. (2017). Biology and biogenesis of shed microvesicles. Small GTPases 8:220–32.
- Trkov S, Eng G, Di Liddo R, et al. (2010). Micropatterned three-dimensional hydrogel system to study human endothelial–mesenchymal stem cell interactions. J Tissue Eng Regen Med 4:205–15.
- Wang C, Liang C, Wang R, et al. (2019). The fabrication of a highly efficient self-healing hydrogel from natural biopolymers loaded with exosomes for the synergistic promotion of severe wound healing. Biomater Sci 8:313–24.
- Wang L, Gu Z, Zhao X, et al. (2016). Extracellular vesicles released from human umbilical cord-derived mesenchymal stromal cells prevent life-threatening acute graft-versus-host disease in a mouse model of allogeneic hematopoietic stem cell transplantation. Stem Cells Dev 25:1874–83.
- Wang C, Wang M, Xu T, et al. (2019). Engineering bioactive self-healing antibacterial exosomes hydrogel for promoting chronic diabetic wound healing and complete skin regeneration. Theranostics 9:65–76.
- Wang W, Li P, Li W, et al. (2017). Osteopontin activates mesenchymal stem cells to repair skin wound. PLoS One 12:e0185346.
- Xu H, Huang S, Wang J, et al. (2019). Enhanced cutaneous wound healing by functional injectable thermo-sensitive chitosan-based hydrogel encapsulated human umbilical cord-mesenchymal stem cells. Int J Biol Macromol 137:433–41.
- Xu Q, Sigen A, Gao Y, et al. (2018). A hybrid injectable hydrogel from hyperbranched PEG macromer as a stem cell delivery and retention platform for diabetic wound healing. Acta Biomater 75:63–74.
- Yim N, Ryu SW, Choi K, et al. (2016). Exosome engineering for efficient intracellular delivery of soluble proteins using optically reversible protein–protein interaction module. Nat Commun 7:12277.
- Zhang J, Guan J, Niu X, et al. (2015). Exosomes released from human induced pluripotent stem cells-derived MSCs facilitate cutaneous wound healing by promoting collagen synthesis and angiogenesis. J Transl Med 13:49.
- Zhang K, Zhao X, Chen X, et al. (2018). Enhanced therapeutic effects of mesenchymal stem cell-derived exosomes with an injectable hydrogel for hindlimb ischemia treatment. ACS Appl Mater Interfaces 10:30081–91.
- Zhang X, Li J, Ye P, et al. (2017). Coculture of mesenchymal stem cells and endothelial cells enhances host tissue integration and epidermis maturation through AKT activation in gelatin methacryloyl hydrogel-based skin model. Acta Biomater 59:317–26.
- Zhao X, Liang Y, Huang Y, et al. (2020). Physical double‐network hydrogel adhesives with rapid shape adaptability, fast self‐healing, antioxidant and NIR/pH stimulus‐responsiveness for multidrug‐resistant bacterial infection and removable wound dressing. Adv Funct Mater 30:1910748.
- Zhang Y, Chopp M, Meng Y, et al. (2015). Effect of exosomes derived from multipluripotent mesenchymal stromal cells on functional recovery and neurovascular plasticity in rats after traumatic brain injury. J Neurosurg 122:856–67.
- Zhou Y, Nie W, Zhao J, Yuan X. (2013). Rapidly in situ forming adhesive hydrogel based on a PEG-maleimide modified polypeptide through Michael addition. J Mater Sci Mater Med 24:2277–2286.