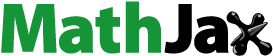
Abstract
Uncertainties about the size of fissile-material stockpiles are large; states routinely observe differences between measured physical inventories and “book inventories.” Nuclear archaeology can reduce these uncertainties. A new, integrated approach would make use of data-analysis techniques that take into account direct fissile-material measurements, a variety of forensic measurements for shut-down facilities and radioactive wastes, and fuel-cycle operational records with related simulations. This article describes that approach and includes a hypothetical case illustrating its potential to probe declared histories and detect falsifications. The article reviews past efforts to fully capture fissile-material stockpiles. Future scenarios in which methods of nuclear archaeology could be used or will be required are also discussed, including improving nuclear safety and security through better knowledge of fissile materials and radioactive waste. International exercises for transparency purposes could be carried out in either nuclear-weapon or non-nuclear-weapon states. During any disarmament process, baseline declarations of fissile materials will need to be verified to assess the potential for a warhead buildup during arsenal reductions and eventually to verify the disposal of all weapons-related fissile materials.
IntroductionFootnote1
Accounting for nuclear materials is notoriously difficult. For example, while the United States stated in 1996 that 1.1 tonsFootnote2 of plutonium entered the waste stream at the Hanford production site, a 2011 estimate assumes 4 tons.Footnote3 A particular nuclear-waste crib in Hanford may have contained 150 kilograms (kg) of plutonium instead of the previously assumed 27 kg, in concentrations high enough that water intrusion could have started a nuclear-criticality event.Footnote4 As of 2009, the total plutonium stock that the United States is managing is 2.4 tons less than what it should possess according to its records.Footnote5 This quantity theoretically corresponds to hundreds of weapon-equivalents. It is, however, unclear whether any of this missing material ever existed.
Similarly, around the time (2012) when the United States, Russia, and Kazakhstan completed 17 years of work to secure fissile material remaining at the Semipalatinsk nuclear test site in Kazakhstan, a Kazakh survey team discovered plutonium residue in five new areas at the site. In some cases, the amounts were sufficient for a nuclear weapon, and in concentrations high enough to pose a proliferation risk. Generally, establishing an accurate inventory of the Semipalatinsk plutonium appeared impossible; the situation is probably similar at other test sites.Footnote6 As long as physical-protection measures are in place—as they are at Semipalatinsk—the threat is presumably low. This article will, however, emphasize the difficulty of maintaining such measures over long periods of time.
These are only two examples of uncertainty and incompleteness of knowledge around fissile-material inventories, which pose challenges for nuclear security, safety, and arms control. These uncertainties can be expected in particular in states with significant past or present nuclear programs, especially military nuclear programs.
The examples above show that the quantification and characterization of fissile-material inventories cannot be based only on direct measurement. Unknown inventories may exist, and, in some cases, materials may be inaccessible to accurate measurement (especially in nuclear waste). A complementary method is to examine the history of the fissile materials. By studying records and other sources of knowledge detailing past production and removals, one may calculate the expected inventories, which can be compared with the fissile materials accounted for.Footnote7 While this is already done to some extent, new approaches should be developed to make such an undertaking as effective as possible while recognizing that knowledge gaps will always remain.
The field of reconstructing fissile-material history, known as “nuclear archaeology,” has been a topic of research since about 1990. This article begins by examining past instances of determining baseline inventories, identifying several shortcomings. Then it illustrates the potential for innovation in this area by means of a case study with relevance for future verification in North Korea. Lastly, the article provides an overview of the various areas that could benefit from further nuclear-archaeology research. They include nuclear security, nuclear safety, international transparency in military programs, and verification of nuclear disarmament.
Instances of determining baseline fissile-material inventories
This section will examine several cases in which fissile-material inventories were investigated, highlighting challenges that occurred and opportunities for improvement in the future.
IAEA safeguards
All states that have joined the 1968 Treaty on the Non-Proliferation of Nuclear Weapons (NPT) must conclude a comprehensive safeguards agreement (CSA) with the International Atomic Energy Agency (IAEA). Each must submit an initial declaration, which the IAEA then verifies for correctness and completeness. But, in practice, only when Additional Protocols are also in place is the IAEA able to conclude that all nuclear materials within a state are being used for peaceful purposes. To reach this “broader conclusion,” the IAEA evaluates all the available information it has about a state. This includes information and access to facilities that the examined state must provide. Facility designs, research activities, information from inspections, and any other available information may contribute to this endeavor.
To issue a broader conclusion, the IAEA must have addressed all identified anomalies, discrepancies, and inconsistencies.Footnote8 For states that had extensive fuel cycles before joining the NPT, confirming the correctness and completeness of initial declarations is a complex undertaking, requiring significant cooperation, including provision of information on past activities, from the inspected state.Footnote9 In the 1990s, for example, the list of states with prior nuclear programs that signed safeguards agreements included Argentina, Brazil, Kazakhstan, Lithuania, North Korea, South Africa, and Ukraine.
Indeed, according to the IAEA, “Transparency in nuclear activities in a State increases the IAEA’s understanding of the nuclear programme, facilitates analysis of its coherence and consistency, and ultimately increases confidence in the conclusions drawn by the IAEA for that State.”Footnote10 Despite the importance of information on the past, there is no legal obligation for states to provide details. The IAEA also acquires confidence over time as safeguards are being routinely applied, if no suspicions arise. A more systematic examination of the history could, however, identify inconsistencies in a more timely manner.
The IAEA in South Africa
Arguably the most extensive reconstruction of past nuclear activities occurred during the IAEA’s inspection visits in 1992–93 when South Africa joined the NPT. The country's history of fissile-material production was reconstructed in detail, as the South Africans gave the IAEA access to thousands of pages of documentation.Footnote11 This allowed the IAEA to assess the size of the inventory of highly enriched uranium (HEU) that South Africa would be expected to possess, which can be obtained by making calculations based on the known production history. If the declared inventory is smaller than this value, it could hint at the existence of undeclared materials.
One major issue in the South African case was that the state had limited knowledge about the uranium-235 (U-235) content of various wastes from enrichment. South African calculations showed that the uncertainty of the U-235 content in enrichment tails (the depleted material that has been separated from the uranium feed) translated into an uncertainty of 526 kg in the amount of U-235 in the HEU product.Footnote12 According to South Africa, the actual discrepancy between expected and measured inventory was less than one-fifth this value and therefore the declared inventory was, as the IAEA concluded in 1993, consistent with the operational history. On the basis of the available information, the IAEA could, however, not strictly exclude the possibility that South Africa had produced more HEU than declared.Footnote13
While the IAEA did measure the isotopic compositions for some of the tails during those early years,Footnote14 Thomas Cochran proposed that the uncertainty could be reduced if the tails were remeasured more accurately.Footnote15 It was only later that various forms of waste were fully measured, a process that took several years. After performing this remeasurement, the inspectors finally stated that the HEU was fully accounted for.Footnote16 Given the long duration of assessing completeness for the South African nuclear-weapons program, which was a relatively small one, it becomes clear that modern approaches are required to verify larger programs.
Non-weapon and weapon states’ own knowledge of fissile materials
Just as South Africa had retained a large amount of the documentation that its experts examined to prepare its initial declaration to the IAEA as an incoming member of the NPT, other countries that had significant nuclear programs prior to joining the treaty presumably also reviewed their programs’ histories for the same purpose. Beyond the context of international safeguards, all nuclear-armed states can be expected to have nuclear-material-accounting systems in place; the extent to which they have retained their operational records likely varies from state to state. At the same time, the extent of such efforts, the depth of knowledge, and the uncertainties involved will vary, probably widely.
A number of non-weapon states provide information about their civilian-plutonium holdings by regularly submitting voluntary updates according to the IAEA INFCIRC/549 guidelines.Footnote17 All nuclear-weapon states that are parties to the NPT provide such declarations, and, in recent years, the United Kingdom and France have also declared civilian HEU holdings.
To date, of the weapon states, only the United States and the United Kingdom have made public declarations about their military fissile-material inventories, which also include information on their production histories. The US Department of Energy issued its plutonium declaration in 1996Footnote18 and updated it in 2012;Footnote19 US HEU holdings were declared in 2006 and updated later the same year.Footnote20 The US declarations provide detailed information on annual production rates, broken down by sites, and include some information about isotopics (U-235 content in HEU and plutonium-239 [Pu-239] in plutonium).
The United Kingdom made its first declaration in 1998, essentially in a single sentence in a report by its Ministy of Defence: “Our current defence stocks are 7.6 tonnes of plutonium, 21.9 tonnes of highly enriched uranium and 15,000 tonnes of other forms of uranium.”Footnote21 It released somewhat more detailed declarations on historical plutonium and HEU accounting in 2000 and 2006.Footnote22
Some of these reports go into depth about the problems encountered in accounting for these materials. The United States, according to the 2012 Department of Energy report, could not resolve uncertainties “about how much plutonium was actually produced, processed, and discarded to waste, especially for the period from the mid-1940s to 1970 before advances in nuclear material measurement systems and computer-aided tools to assist in the analysis of nuclear material accounting data.”Footnote23 The report specifically addresses waste-characterization challenges. While in 1996 it was estimated that 3.4 tons of plutonium were lost to waste, more recent assessments indicate that the waste actually contained 12.7 tons of plutonium, over 10 percent of the overall amount produced.Footnote24
The authors of the 2012 report suspect that the discrepancy between the book assessment and the physical inventory is explained by the uncertainties of measurements in the past and today, such as the difficulty of measuring plant holdup,Footnote25 or uncertainties due to wide variations in the material forms, statistical uncertainties, and lack of adequate measurement technology. Other problems may include unmeasured material from accidental spills, as well as recording, reporting, and rounding errors. Furthermore, “[I]t can be seen that 68% of the inventory difference occurred during the period prior to the late 1960’s, when predictive reactor codes … and materials measurement technologies were less accurate than today.”Footnote26 Issues for HEU inventories are similar: the US inventory difference is about 3 tons.Footnote27 The United Kingdom has faced similar issues. The Ministry of Defence authors note that a considerable number of records from the early years of the program have been destroyed and that some records lack the precision now required. For example, some figures did not include mass units, or were rounded.Footnote28
An integrated approach to nuclear archaeology
The first major challenge in determining a state’s fissile-material inventory is the limitations of direct measurements of those materials, as the US plutonium report indicates. Not only do measurements involve uncertainties, but sometimes they cannot be carried out effectively at all. In the verification context, fissile materials may be unavailable for direct measurement, or deliberately withheld. These problems limit the reliability of basing inventory estimates only on direct measurements taken today.
Documentation issues in general are a second major challenge. The United States collected only limited nuclear-material-accounting data until 1970. In the United Kingdom, information was lost, as described above. In a hypothetical verification context, documentation may be deliberately falsified or left incomplete. Recent work has further developed approaches to critically examine such sources.Footnote29
To reconstruct production histories, nuclear-archaeology research has focused on forensic measurements at shut-down facilities and of stored waste. For example, a reactor’s cumulative plutonium production can in some cases be estimated by measuring samples from permanent structures within the reactor core (that is, anything that remains within the core even as fuel is exchanged, such as graphite moderators);Footnote30 methods to assess past uranium enrichment also exist to a limited extent, including measurement of enrichment tails.Footnote31 In general, only limited research on forensic measurements has been carried out. Most proposals need further validation.
Forensic measurements have important shortcomings. Without taking additional background information into account, uncertainties may be large.Footnote32 In some cases, these measurements may not even be possible. Inferring plutonium production from reactor-core samples, for example, will be infeasible if the permanent structure is insufficient for sampling. Production reactors may even have been dismantled when measurements are sought.
Because knowledge gaps in nuclear material inventories can result in grave consequences, nuclear-archaeology research should be expanded. New measurable forensic signatures that are complementary to existing ones should be identified. Perhaps even more importantly, a major goal should be to develop more effective data-analysis approaches to evaluate various sources of information in combination—toward integrated nuclear-archaeology approaches.Footnote33 These include fissile-material measurements today where possible, documentation of past fissile-material production and of removals (for example, material lost to waste or used in weapons tests), and an expanded set of forensic measurements.
An illustrative example
Different nuclear-archaeology applications and contexts require different technical approaches. The following example provides a verification scenario in which past reactor operations are to be reconstructed.
The goals are twofold. The first is to reduce the overall uncertainty of fissile-material assessments by combining information from various sources. Uncertainties will, however, always remain. Therefore, a second goal is equally important: to be able to reconstruct information missing from each of the separate data sources and to detect and resolve inconsistencies between them. This means that a declaration backed up by documentation can be probed more effectively.
Specifically, this article considers measuring reprocessing waste for this purpose in conjunction with a state’s hypothetical declaration.Footnote34 The waste could remain accessible for many years before it is permanently disposed of, and potentially after the fuel-cycle facilities that produced it have been dismantled. It contains a rich isotopic signature of past fuel-cycle activities.
A precedent for measuring this waste can be found in IAEA verification activities in North Korea in the early 1990s. After having signed a safeguards agreement with the IAEA, North Korea issued its initial declaration for the NPT in 1992. The declaration stated that Pyongyang had conducted only one reprocessing campaign of damaged spent-fuel rods from the gas-graphite reactor at Yongbyon, in 1990, containing 84 grams of plutonium, while the other fuel rods from the first load remained in the reactor. The separated plutonium was presented to IAEA inspectors, who found that it contained 2.4 percent plutonium-240 (Pu-240). Sampling the high-level reprocessing waste revealed, however, a Pu-240 fraction of about 2.25 percent. The IAEA concluded that North Korea had separated additional undeclared plutonium in kilogram quantities, either by discharging more spent fuel than declared from the gas-graphite reactor, by separating plutonium from a Soviet-supplied research reactor nearby, or both. The IAEA was not able to fully reconstruct the plutonium-production history.Footnote35
As the following—very simplified—hypothetical case study involving a plutonium-production program will show, an analysis of a larger number of isotopes from waste can, in principle, yield additional information regarding past nuclear operations. Let us assume that a state provides documentation on its past nuclear activities. It declares that it operated a test reactor only once and produced non-weapons-grade plutonium. In addition to providing information on operating records and reactor design, the state allows inspectors to take waste samples. The inspectors then examine whether the waste samples are consistent with the declared information. The state’s declaration is, however, incomplete. This case study assumes that fuel has been discharged and reprocessed not once, but twice, and that the other, undeclared campaign produced weapons-grade plutonium. The actual scenario this alludes to is a state hiding some past production of military plutonium within a declared civilian program.
The corresponding comprehensive waste composition that inspectors would measure has been calculated for a specific scenario: plutonium with a burnupFootnote36 of 0.8 megawatt-days per kilogram (MWd/kg) in a heavy-water reactor 30 years ago and with a burnup of 1.8 MWd/kg 10 years ago. Burnup indicates whether the plutonium from a specific irradiation campaign was weapons-grade or non-weapons-grade. Based on the expected measurement results for several isotopes, the Appendix shows that the production history (the two ages and two burnup values) can be correctly reconstructed and the two campaigns can be correctly distinguished.
The Appendix also presents an evaluation of the uncertainties in such assessments. While the previous results could have been obtained without any prior information from a declaration, the Appendix summarizes research that has shown that the uncertainty about burnup and age from an undeclared campaign can be reduced by prior information (for example, from provided documentation) about declared campaigns. This becomes possible by exploiting a statistical technique combined with the simulation of past fissile-material production using modern codes.Footnote37
These results are promising. Should inspectors obtain access to waste in North Korea in the future, it appears that they could use such a method to verify a new initial declaration.Footnote38 It could be part of a wider toolbox, also including forensic measurements at the Yongbyon gas-graphite reactor and information on reactor operations based on satellite imagery.
The roles of nuclear archaeology
The following sections review and summarize the relevance of nuclear-archaeology methods, including the proposed integrated approach.
Improving knowledge of fissile-material inventories for nuclear security and nuclear safety
While some states, notably the United States, have engaged in research into nuclear archaeology, its application is not mentioned in any fissile-material declarations or reports. Nuclear archaeology appears not to have been used to better understand states’ own production histories as a whole.
Both in weapon and non-weapon states, nuclear-archaeology methods can be used in the context of nuclear security. Nuclear-security discussions usually center on physically securing plutonium and HEU to reduce the risk of theft or sabotage, in addition to minimizing the use of those materials in civilian programs. However, physical protection will likely fail at some point in the future, as the National Academy of Sciences (NAS) in Washington found in a 2000 report. The study notes that “there is no convincing evidence that institutional controls and other stewardship measures are reliable over the long term. … [T]he likelihood that institutional management measures will fail at some point is relatively high.”Footnote39 An important part of a comprehensive risk assessment needs to be a detailed understanding of the nuclear materials that could be obtained should institutional controls fail. One issue is that nuclear materials contained in waste or in contaminated soil—as a result of weapons tests, for instance—can pose a concern: waste that is considered disposed of and therefore no longer included in accountancy systems can be recovered in some cases. The US standard for terminating domestic safeguards even includes provisions for returning materials that have been removed from safeguards to the active inventory.Footnote40
Large uncertainties can be expected in waste. The NAS study notes a lack of information about the contents of high-level-waste tanks, buried radioactive waste (such as the low-level-waste sites at Hanford), and environments contaminated by nuclear testing (such as the Nevada Test SiteFootnote41).
While the United States has engaged in remeasuring waste,Footnote42 other states may have less knowledge about their waste today. A large effort went into assessing remaining plutonium at the Semipalatinsk site, Footnote43 but it is not clear, for example, whether the Russians have engaged in a similar effort to characterize what remains at the Novaya Zemlya test site in the Russian Arctic.
Fully characterizing and accounting for nuclear materials by applying nuclear-archaeology methods would offer benefits in nuclear security and safety. Conducting nuclear archaeology to improve states’ own inventory assessments would also serve as a confidence-building measure, showing that they take seriously their commitments to nuclear security and safety. Ideally, the results would be made public. But even the provision of less specific information, such as descriptions of the activities being pursued, would be useful. States conducting such assessments, both with and without nuclear weapons, would then have a stronger case when demanding that others better assess and secure their fissile materials. Indeed, as a study by the Nuclear Threat Initiative on baseline declarations notes, preparing nuclear-material baselines could prompt “all states to undertake a process of fully characterizing and accounting for all weapons-usable materials, which, in turn, sharpens the focus on improving nuclear materials security.”Footnote44
While nuclear archaeology is primarily concerned with quantifying plutonium and HEU production, the full isotopic composition of waste is obtained from reactor simulations. By characterizing fission products and actinides that pose safety threats, this process would produce benefits for nuclear safety beyond fissile materials.
For instance, referring to high-level-waste tanks, the NAS writes,
Historical records are used to estimate the initial load of waste in the tank because an assay of the residue can be extremely difficult. Some of the residue is sludge or solids trapped in difficult-to-reach locations of the structures; some is caught on or in the corroded carbon steel surface of the tank interior. Thorough, direct sampling and characterization are not really possible in a practical sense.Footnote45
Fissile-material transparency in military programs
Against the backdrop of the Russo-Ukrainian War and the generally deep mistrust between competing nuclear powers, any new confidence-building measures are difficult to imagine in the short term. Nevertheless, past outcomes of the NPT review process should be taken seriously. For example, it was agreed at the 2010 NPT Review Conference that the nuclear-weapon states should agree on a possible format for declarations that include inventories of military fissile material, among other information.Footnote46 The Non-Proliferation and Disarmament Initiative, a cross-regional group of non-nuclear-weapon states, proposed a specific reporting format in anticipation of declarations of aggregate amounts of plutonium and HEU produced for national-security purposes.Footnote47
Unfortunately, apart from the existing detailed US and UK declarations, as well as INFCIRC/549 declarations of civilian stocks, nuclear-weapon states currently do not provide transparency with respect to their fissile-material inventories. Governments consider such information to be sensitive. There are, however, options for transparency and confidence building related to nuclear archaeology that would not require new declarations on fissile-material inventories.
International exercises
An initial step to advance the state of nuclear archaeology—and, at the same time, bridge the divide between weapon and non-weapon states—could be to hold an international exercise in which the methodology for reconstructing fissile-material inventories is discussed. Selected documentation from past operations could be used as a basis to discuss how to determine how much fissile material has been produced. Furthermore, the exercise could explore how forensic measurements taken today could hypothetically be used—or how such measurements could even be carried out. In such a setting, nuclear-weapon states could decide how much information to share about their processes for examining their inventories.
Such an exercise need not even be held in a nuclear-weapon state.Footnote48 Some civilian programs share technical characteristics with military programs, such as the use of spent-fuel reprocessing and of heavy-water reactors fueled with natural uranium. In fact, non-weapon states such as Germany, Canada, and Japan, which had civilian nuclear programs with various fuel-cycle facilities before entering the NPT, have relevant experience from preparing their initial declarations for the IAEA.
Non-weapon states that have less experience with preparing baseline declarations would gain an understanding of this process, which would help them to put into context declarations such as those of the United States and United Kingdom and the uncertainties that those two countries mention. Confidence in these types of declarations could be further enhanced if states that issued them also provided insights into their methodology and the nature of the documentation involved.
Inspection visits at select facilities
Moving from exercises to implementation, an inspection could be carried out to assess a specified part of an existing declaration, in particular in the United States or the United Kingdom. It could directly build confidence in the correctness of the declaration(s). In 1994, the United Kingdom allowed the United States to measure samples from one of its reactors,Footnote49 which could be seen as a precedent among weapon states. The same two countries undertook a similar exercise in 2022.Footnote50 The United States and Canada conducted a joint exercise in 2021.
A first inspection involving non-weapon states might be limited in scope, perhaps by including operational records of only one nuclear facility covering a period of one or two years (and no measurements). The United States has already released figures on the amount of plutonium produced per site per year in its military fissile-material-production complex, along with some additional information regarding the operation of facilities, such as the power levels of the reactors. The United Kingdom has declared annual information on plutonium transfers from the reprocessing facility in Sellafield to the weapons program in Aldermaston. Examining information for only one year or a few years would prevent inference of knowledge about the total inventory, which would be seen as too intrusive at this point.
Verified declarations for disarmament
While nuclear archaeology is helpful for confidence-building and transparency measures, reconstructing fissile-material histories is indispensable for verifying disarmament, as the South African case has demonstrated. Current research on disarmament verification focuses on warhead-related measures, in particular verifying warhead dismantlement.Footnote51 This work involves stringent requirements for protecting sensitive information, posing a great challenge for the development of corresponding verification technologies.
Due to this difficulty, an alternative and less intrusive approach has been developed that focuses on weapons-usable fissile materials instead of warheads: deferred verification.Footnote52 This concept requires a baseline declaration of all weapons-usable fissile materials. Some of these are placed in an “open segment” that may be verified; the rest can be placed in a “closed segment” without verification, including all fissile materials that are in weapons at the time and any that may still be required for national-security purposes. The disarmament process is then verified by measuring fissile materials as they leave the closed segment.
A detailed assessment of weapons-usable fissile materials has been published by Pavel Podvig and Ryan Snyder. Based on this assessment, there is a total of 1,200 tons of HEU and 530 tons of separated plutonium as of 2019 in the world’s military and civilian programs. Of this, about 15 percent of the HEU and 7 percent of the separated plutonium were in weapons. There were significant stocks of military materials not in weapons but available for that purpose. These HEU stocks amount to 749 tons, or about four times the stocks estimated to be in weapons. The separated plutonium stocks add up to 85 tons, or about twice the amount estimated to be in weapons. Additional stocks are in weapons no longer required for defense purposes.Footnote53
Assessing stability during disarmament
Nuclear archaeology can be used to assess stability during nuclear disarmament. Furthermore, it is indispensable to verifying that disarmament is not reversible, and it can even be used under specific circumstances to assess warhead baseline declarations.
Verified fissile-material declarations will at some stage also likely be required from a stability perspective: as long as nuclear-weapon states subscribe to deterrence theory, negotiators will be likely to seek to maintain strategic stability—however they would exactly define itFootnote54—as nuclear forces are reduced to lower levels.Footnote55 The potential to build new warheads must be taken into account during reductions to low warhead numbers. Such reductions would necessitate the verification of fissile-material declarations. Otherwise, a state could under-declare its available fissile-material inventory, allowing it to emerge later with a perceived military advantage.Footnote56 Since a moderately sophisticated nuclear device could contain as little as 3–4 kg of plutonium or 12–15 kg of HEU, speculation about fissile-material stockpiles unaccounted for, possibly equivalent to hundreds of nuclear weapons, could make deep cuts very hard to achieve.
In principle, fissile-material declarations could be verified by on-site inspections of all fissile materials available for weapons, including in the national-security sectors. This would be highly intrusive. At least in larger nuclear-weapon states, there are many locations (such as sites of deployed weapons or facilities for storage of warheads or pits), many of which are in active military use. There, it would be a significant challenge to design verification approaches to protect sensitive information. In such an environment, obtaining confidence in the accuracy of fissile-material declarations through on-site visits would even require extensive short-notice challenge inspections at sensitive sites, something that is very hard to imagine. In practice, therefore, nuclear archaeology would provide a much less intrusive way, not requiring direct access to the materials.
Verifying effective irreversibility of disarmament
Assessing inventories of weapons-usable fissile material will be necessary to verify that nuclear disarmament is irreversible. This article adopts the definition of irreversibility proposed by James Fuller and his colleagues: “making it physically difficult and costly to recover material for use in weapons—for example, by blending down HEU to [low-enriched uranium] or using plutonium in mixed oxide fuel (MOX) or immobilizing it.”Footnote57
Irreversible disarmament will therefore require the verified conversion to civilian purposes or disposition of fissile materials from weapons. After weapons have been dismantled and the recovered fissile material no longer has sensitive characteristics, it will become subject to safeguards. Assuming that all other fissile materials are safeguarded, including those in naval fuel, a state will move toward placing all fissile materials under safeguards as dismantlement proceeds.
Inspectors would compare the quantity of fissile materials under safeguards to the amount of fissile materials a state should possess according to nuclear archaeology. As nuclear disarmament progresses, one moves toward “closing the fissile material balance.”Footnote58 The IAEA inspections in South Africa show that the international community requires such an effort to be confident that a state has fully disarmed.
The link to warhead declarations
Apart from verifying fissile-material declarations, one of the most important unresolved issues is how to verify warhead declarations. Unfortunately, there is no reliable method for detection of undeclared warheads. But in small states with limited nuclear programs, such as North Korea, nuclear archaeology could be used to bound the problem. If confidence can be built in knowledge of the amount of material produced, as well as the amount of material removed, and if the size of fissile-materials stocks not used in warheads is also known (for example, from on-site inspections), then the amount of fissile material in or available for warheads can be known. If there are uncertainties in the removals or the existing stocks outside of warheads, an upper bound of fissile material in warheads can be obtained.
It may not be known how much fissile material is required per warhead for a specific design. One option is that a state provide warhead design information to be examined by weapon-state inspectors. For instance, South Africa made records available to the IAEA concerning the HEU components of its weapons. This allowed the IAEA, working with nuclear-material-accountancy records and other data, to build confidence that no further nuclear warheads existed.Footnote59 A similar scenario could work for North Korean denuclearization. It may even be possible that North Korea, if it decided to give up its nuclear program, could show one or several warheads to inspectors from weapon states as part of the process.
Even without detailed knowledge of warhead designs, plausible assumptions could be made, although they would involve some uncertainty. Since fission warheads require only a few kilograms of plutonium, or somewhat more HEU, and uncertainties in fissile-material estimates are inevitable, it will usually not be possible to detect with confidence that a single warhead or a few have not been declared. By way of illustration, a very rough fissile-material estimate for North Korea (35 kg of plutonium and 750 kg of HEU) and an upper-bound uncertainty estimate of 10 percent on plutonium and 20 percent on HEU would result in an uncertainty of around seven warheads.Footnote60 What is important is the ability to conclude that no militarily significant number of warheads is hidden.
Applying nuclear archaeology to disarmament
By the time nuclear archaeology would be required for continuing the disarmament process, the nuclear-weapon states should have reduced their inventory uncertainties by having conducted nuclear archaeology for their own production histories. Due to the vast amounts of fissile materials produced in these states, however, even uncertainties that are relatively small in terms of percentage will still be equivalent to a fairly large number of warheads. Once the United States has reduced its weapons arsenal to a triple-digit number, for instance, the uncertainties of fissile-material estimates may—in terms of warhead-equivalents—be in a similar range of warhead numbers. In other words, the fissile-material uncertainty could translate into an uncertainty of warhead inventories (or of the potential to build warheads) that is in the same range as the actual declared warhead inventory at that point.Footnote61As the South Africa case shows, some quantitative uncertainty can be acceptable if the underlying reasons are adequately explained and any inconsistencies are addressed cooperatively. This goal will nevertheless require a level of trust that must be built over years.
The integrated nuclear-archaeology approach, with its forensic measurements and data-analysis methods, offers benefits over the traditional approach of only comparing book inventories with physical inventories. Besides the ability to reduce uncertainties, its capability for checking consistency is particularly important. The use of forensic measurement techniques that examine complementary signatures is ideal. The goal is to create an interlocking web of techniques that would make it difficult to undertake a large-scale falsification of the fissile-material history that would be consistent with all forensic measurements.
In addition to nuclear-archaeology methods to assess fissile-material production, methods that can assess removals must be developed, as fissile materials in weapons can be under-declared by over-declaring lost fissile materials, even assuming a correct declaration on the production side. Extensive measurement campaigns at waste and former weapon-test sites would be helpful.
Conclusion
Among the scenarios in which nuclear archaeology would become important, some cases could occur in the short or medium term, particularly those relating to nuclear security and fissile-material transparency. At the same time, advancing the nuclear-archaeology toolbox will require extensive research. Based on past progress, it seems plausible that it will take at least another decade for the relevant methods to be fully developed and tested. Luckily, the available nuclear-security and transparency options do not require that these methods be developed to their full extent. Nuclear archaeology in its current state could be applied in certain situations. Such applications would help engineers, researchers, and facility operators gain experience with nuclear archaeology and could even help advance nuclear-archaeology research itself. In any case, it is important to pursue an ambitious research agenda now, to ensure that the methods are available when they are required.
Acknowledgments
This research has been generously supported by the Volkswagen Foundation. The case study described in the Appendix is based on previously published research, to which Antonio Figueroa and Martin Frank contributed.
Disclosure statement
No potential conflict of interest was reported by the author.
Additional information
Notes on contributors
Malte Göttsche
Malte Göttsche is a junior professor at RWTH Aachen University, where he leads the Nuclear Verification and Disarmament Group. Previously, he was a postdoctoral research associate in Princeton University’s Program on Science and Global Security, after having been awarded a doctoral degree in physics by the University of Hamburg. He develops concepts and scientific techniques to verify nuclear nonproliferation and disarmament.
Notes
1 This paper was written and reviewed in 2020–21 and has been only slightly revised in 2023 for final publication.
2 Here and throughout this article, “tons” means “metric tons.”
3 Robert Alvarez, “Plutonium Wastes from the U.S. Nuclear Weapons Complex,” Science & Global Security, Vol. 19, No. 1 (2011), p. 21
4 Alvarez, “Plutonium Wastes,” p. 21.
5 US Department of Energy, “The United States Plutonium Balance, 1944–2009,” 2012, p. 2, <https://fissilematerials.org/library/doe12.pdf>.
6 Eben Harrell and David E. Hoffman, “Plutonium Mountain: Inside the 17-Year Mission to Secure a Dangerous Legacy of Soviet Nuclear Testing,” Belfer Center for Science and International Affairs, Harvard University, August 2013, <https://www.belfercenter.org/sites/default/files/legacy/files/Plutonium%20Mountain-Web.pdf>.
7 Alexander Glaser and Malte Göttsche, “Fissile Material Stockpile Declarations and Cooperative Nuclear Archaeology,” in Verifiable Declarations of Fissile Material Stocks: Challenges and Solutions, FM(C)T Meeting Series, United Nations Institute for Disarmament Research, 2017, <https://unidir.org/sites/default/files/publication/pdfs//fm-c-t-meeting-series-verifiable-declarations-of-fissile-material-stocks-challenges-and-solutions-en-671.pdf>.
8 IAEA, “Safeguards Statement for 2018,” 2019, <https://www.iaea.org/sites/default/files/19/06/statement-sir-2018.pdf>.
9 Olli Heinonen, “Verification of the Correctness and Completeness of Initial Declarations,” in Symposium on International Safeguards: Verification and Nuclear Material Security (proceedings of the conference held October 29 to November 2, 2001), Vienna, 2001, <https://www-pub.iaea.org/MTCD/Publications/PDF/SS-2001/Start.pdf>.
10 IAEA, “Guidance for States Implementing Comprehensive Safeguards Agreements and Additional Protocols,” IAEA Services Series 21, May 2016, <https://www-pub.iaea.org/MTCD/Publications/PDF/SVS-21_web.pdf>.
11 IAEA, “The Denuclearization of Africa,” GC(XXXVII)/1075, September 9, 1993, <https://www.iaea.org/sites/default/files/gc/gc37-1075_en.pdf>.
12 This uncertainty value corresponds to two standard deviations (that is, the true value lies within plus or minus 526 kg with 95 percent probability). Thomas Cochran, “Highly Enriched Uranium Production for South African Nuclear Weapons,” Science & Global Security, Vol. 4, No. 2 (1994), pp. 161–76.
13 Cochran, “Highly Enriched Uranium Production”; Nathan E. Busch and Joseph F. Pilat, The Politics of Weapons Inspections: Assessing WMD Monitoring and Verification Regimes (Stanford, CA: Stanford University Press, 2017).
14 IAEA, “South Africa’s Nuclear Capabilities,” GC(XXXVI)/1015, September 4, 1992, <https://www.iaea.org/sites/default/files/gc/gc36-1015_en.pdf>.
15 Cochran, “Highly Enriched Uranium Production.”
16 Jodi Lieberman, “Dismantling the South African Nuclear Weapons Program: Lessons Learned and Questions Unresolved,” in Henry D. Sokolski, ed., Nuclear Weapons Materials Gone Missing: What Does History Teach? (Carlisle Barracks, PA: United States Army War College Press, 2014), p. 142; Olli Heinonen, “Verifying the Dismantlement of South Africa’s Nuclear Weapons Program,” in Henry D. Sokolski, ed., Nuclear Weapons Materials Gone Missing: What Does History Teach? (Carlisle Barracks, PA: United States Army War College Press, 2014).
17 IAEA, “Communication Received from Certain Member States Concerning Their Policies Regarding the Management of Plutonium,” INFCIRC/549, Vienna, March 16, 1998, <https://www.iaea.org/publications/documents/infcircs/communication-received-certain-member-states-concerning-their-policies-regarding-management-plutonium>.
18 US Department of Energy, “Plutonium: The First 50 Years,” DOE/DP-0137, February 1996, <www.ipfmlibrary.org/doe96.pdf>.
19 US Department of Energy, “The United States Plutonium Balance, 1944–2009,” June 2012, <www.ipfmlibrary.org/doe12.pdf>.
20 US Department of Energy, “Highly Enriched Uranium: Striking a Balance (Revision 1),” 2001 (publicly released in 2006 under the Freedom of Information Act), <www.ipfmlibrary.org/doe01.pdf>; US Department of Energy, “Highly Enriched Uranium Inventory: Amounts of Highly Enriched Uranium in the United States,” 2006, <www.ipfmlibrary.org/doe06f.pdf>.
21 UK Ministry of Defence, “Strategic Defence Review: Modern Forces for the Modern World,” 1998, <www.ipfmlibrary.org/mod98.pdf>. (A “tonne” is a metric ton.)
22 UK Ministry of Defence, “Plutonium and Aldermaston—An Historical Account,” 2000, <fissilematerials.org/library/mod00.pdf>; UK Ministry of Defence, “Historical Accounting for UK Defence Highly Enriched Uranium,” 2006, <www.ipfmlibrary.org/mod06.pdf>.
23 US Department of Energy, “The United States Plutonium Balance.”
24 This includes 3.7 tons that were originally known, but had not been classified as waste. Alvarez, “Plutonium Wastes.”
25 “Plant holdup” refers to materials remaining in tanks, piping, and other locations—for instance in reprocessing plants.
26 US Department of Energy, “Plutonium.”
27 International Panel on Fissile Materials, “Global Fissile Material Report 2010,” 2010, <https://fissilematerials.org/library/gfmr10.pdf>.
28 UK Ministry of Defence, “Historical Accounting for UK Defence Highly Enriched Uranium.”
29 Ole Reistad, Alex Glaser, Rebecca D. Frank, and Sindre H. Kaald, "Document-Based Nuclear Archaeology," Science & Global Security, Vol. 30, No. 2 (2022), pp. 95–107; Sophie Kretzschmar and Malte Göttsche, “Reconstructing Nuclear Histories—A Field Study,” paper delivered at the Joint INMM/ESARDA Meeting, Vienna, May 22–26, 2023.
30 Alex Gasner and Alexander Glaser, “Nuclear Archaeology for Heavy-Water-Moderated Plutonium Production Reactors,” Science & Global Security, Vol. 19, No. 3 (2011), pp. 223–33; Christopher J. Gesh, “A Graphite Isotope Ratio Method Primer—A Method for Estimating Plutonium Production in Graphite Moderated Reactors,” Pacific Northwest National Laboratory, Richland, WA, 2004; Julien de Troullioud de Lanversin, Malte Göttsche, and Alexander Glaser, “Nuclear Archaeology to Distinguish Plutonium and Tritium Production Modes in Heavy Water Reactors,” Science & Global Security, Vol. 26, Nos. 2–3 (2018), pp. 70–90; Benjamin Jung and Malte Göttsche, “Assessing Uncertainty in Plutonium Production Estimates Based on the Isotope Ratio Method,” Science & Global Security, Vol. 30, No. 1 (2022), pp. 3–21.
31 Matthew Sharp, “Applications and Limitations of Nuclear Archaeology in Uranium Enrichment Plants,” Science & Global Security, Vol. 21, No. 1 (2013), pp. 70–92; Sébastien Philippe and Alexander Glaser, “Nuclear Archaeology for Gaseous Diffusion Enrichment Plants,” Science & Global Security, Vol. 22, No. 1 (2014), pp. 27–49.
32 Jung and Göttsche, “Uncertainty in Plutonium Production Estimates”; Moritz Kütt, Julien de Troullioud de Lanversin and Malte Göttsche, “Understanding Uncertainties in Nuclear Archaeology,” paper delivered at the 59th Annual INMM Meeting, Baltimore, MD, July 22–26, 2018.
33 A possible platform for some nuclear-archaeology scenarios can be provided by fuel-cycle simulation frameworks. See Max Schalz, Lewin Bormann, and Malte Göttsche, “Bicyclus: A Statistical Fuel Cycle Simulation Framework for Nuclear Verification,” Annals of Nuclear Energy, Vol. 196, No. 110221 (2024).
34 This new forensic approach is further discussed in Antonio Figueroa and Malte Göttsche, “Nuclear Archaeology: Reconstructing Reactor Histories,” ESARDA Bulletin, Vol. 59 (December 2019), pp. 39–46; Antonio Figueroa and Malte Göttsche, “Nuclear Archaeology Based on Measurements of Reprocessing Waste: First Experimental Results,” paper delivered at the Joint INMM/ESARDA Meeting, online, August 23 to September 1, 2021; Benjamin Jung, Antonio Figueroa, and Malte Göttsche, “Towards Inferring Reactor Operations from High-Level Waste,” Nuclear Engineering and Technology (2024), https://doi.org/10.1016/j.net.2024.02.031 (corrected proof).
35 This paragraph is based on David Albright and Kevin O'Neill, Solving the North Korean Nuclear Puzzle (Washington, DC: ISIS Press, 2000), pp. 83–126.
36 “Burnup” refers to the fission energy extracted from fuel until its discharge from a reactor. For constant power, it is proportional to the irradiation time. Low burnup is ideal for producing military plutonium because the resulting plutonium has a low Pu-240 content. While plutonium with a higher Pu-240 content (corresponding to higher burnup) is also usable for weapons, it is less suitable.
37 Malte Göttsche, Antonio Figueroa, and Martin Frank, “An Integrated Approach to Nuclear Archaeology,” paper delivered at the 60th Annual INMM Meeting, Palm Desert, CA, July 14–18, 2019.
38 See also Malte Göttsche, Katja Ermert, Benjamin Jung, and Lukas Rademacher, “Verifying Initial Declarations Using Nuclear Archaeology Methods,“ paper delivered at the IAEA Safeguards Symposium, Vienna, October 31 to November 4, 2022.
39 National Research Council, “Long-Term Institutional Management of U.S. Department of Energy Legacy Waste Sites,” National Academies Press, Washington, DC, 2000, p. 3, <https://nap.nationalacademies.org/catalog/9949/long-term-institutional-management-of-us-department-of-energy-legacy-waste-sites>.
40 Pavel Podvig, “Verifiable Declarations of Fissile Material Stocks: Challenges and Solutions,” in Verifiable Declarations of Fissile Material Stocks: Challenges and Solutions, FM(C)T Meeting Series, United Nations Institute for Disarmament Research, 2017.
41 Since 2010, the site has been officially known as the Nevada National Security Site.
42 Alvarez, “Plutonium Wastes.”
43 Harrell and Hoffman, “Plutonium Mountain.”
44 John Carlson, James Fuller, Kelsey Hartigan, et al., “New Tools and New Actors to Reduce Nuclear Risks: Verifying Baseline Declarations of Nuclear Materials and Warheads,” Nuclear Threat Initiative, July 2014, p. 16, <https://www.nti.org/analysis/articles/innovating-verification-verifying-baseline-declarations-nuclear-warheads-and-materials/>.
45 National Research Council, “Long-Term Institutional Management.” This difficulty of measurements is specific to the United States. Other states—for example, in Europe—keep high-level waste in a different chemical form, making measurements easier.
46 2010 Review Conference of the Parties to the Treaty on the Non-Proliferation of Nuclear Weapons, Final Document, Vol. I, NPT/CONF.2010/50, New York, 2010, <https://documents-dds-ny.un.org/doc/UNDOC/GEN/N10/390/21/PDF/N1039021.pdf?OpenElement>.
47 Preparatory Committee for the 2020 Review Conference of the Parties to the Treaty on the Non-Proliferation of Nuclear Weapons, “Proposal by the Non-Proliferation and Disarmament Initiative to Enhance Transparency for Strengthening the Review Process for the Treaty on the Non-Proliferation of Nuclear Weapons,” NPT/CONF.2020/PC.II/WP.26, April 11, 2018, <https://documents-dds-ny.un.org/doc/UNDOC/GEN/N18/103/07/PDF/N1810307.pdf?OpenElement>. Members of the initiative are Australia, Canada, Chile, Germany, Japan, Mexico, the Netherlands, Nigeria, the Philippines, Poland, Turkey, and the United Arab Emirates.
48 Glaser and Göttsche, “Fissile Material Stockpile Declarations.”
49 Bruce D. Reid, David C. Gerlach, Patrick G. Heasler, and J. Livingston, “Trawsfynydd Plutonium Estimate,” PNNL-13528, Pacific Northwest National Laboratory, Richland, WA, 2009. The reactor was used for power production, but some of its reprocessed plutonium may have gone into the weapons program.
50 B. Bowerman, R. Boyd, C. Finrock, C. Gesh, and R. Pires, “Trepanning Graphite Samples at a Shutdown Magnox Reactor: A US–UK Joint Exercise for Nuclear Verification,” paper delivered at the Joint INMM/ESARDA Meeting, Vienna, May 22–26, 2023; National Nuclear Security Administration, “NNSA and Canadian Nuclear Laboratories Conduct Joint Exercise to Improve Future Nuclear Verification Work,” December 20, 2021, <https://www.energy.gov/nnsa/articles/nnsa-and-canadian-nuclear-laboratories-conduct-joint-exercise-improve-future-nuclear>.
51 This is the focus of, for instance, the International Partnership for Nuclear Disarmament Verification. See <www.ipndv.org>.
52 Pavel Podvig and Joseph Rodgers, “Deferred Verification: Verifiable Declarations of Fissile-Material Stocks for Disarmament Purposes,” Nonproliferation Review, Vol. 26, Nos. 3–4 (2019), pp. 209–17.
53 Pavel Podvig and Ryan Snyder, “Watch Them Go: Simplifying the Elimination of Fissile Materials and Nuclear Weapons,” United Nations Institute for Disarmament Research, August 15, 2019, <https://doi.org/10.37559/WMD/19/NuclearVer01>.
54 Criticial views of the term can be found in James M. Acton, “Reclaiming Strategic Stability,” in Elbridge A. Colby and Michael S. Gerson, eds., Strategic Stability: Contending Interpretations (Carlisle, PA: Strategic Studies Institute and US Army War College Press, 2013), <https://publications.armywarcollege.edu/pubs/2216.pdf>; Pavel Podvig, “The Myth of Strategic Stability,” Bulletin of the Atomic Scientists, October 31, 2012, <https://thebulletin.org/2012/10/the-myth-of-strategic-stability/>.
55 David Holloway, Steps toward a World Free of Nuclear Weapons (Stanford, CA: Stanford University Press, 2011), pp. 347–58.
56 Tamara Patton and Alexander Glaser, “Deferred Verification: The Role of New Verification Technologies,” Nonproliferation Review, Vol. 26, Nos. 3–4 (2019), pp. 219–30.
57 Fuller et al., “New Tools and New Actors,” p. 84.
58 Podvig and Rodgers, “Deferred Verification.”
59 IAEA, “The Denuclearization of Africa.”
60 In an interview in 2021, Siegfried Hecker assumes that North Korea had available 25–48 kg of plutonium and 600–950 kg of HEU and that it could build warheads with 5 kg of plutonium or 25 kg of HEU. 38 North, “Estimating North Korea’s Nuclear Stockpiles: An Interview with Siegfried Hecker,” April 30, 2021, <https://www.38north.org/2021/04/estimating-north-koreas-nuclear-stockpiles-an-interview-with-siegfried-hecker/>.
61 For example, if there are 300 weapons, the fissile material estimates may be such that there is the potential to build 1,000 more, plus or minus 300.
1 Antonio Figueroa and Malte Göttsche, “Nuclear Archaeology: Reconstructing Reactor Histories,” ESARDA Bulletin, Vol. 59, (December 2019), pp. 39–46; Malte Göttsche, Antonio Figueroa, and Martin Frank, “An Integrated Approach to Nuclear Archaeology,” paper delivered at the 60th INMM Annual Meeting, Palm Desert, CA, 14–18 July 2019.
2 Jaakko Leppänen, Maria Pusa, Tuomas Viitanen, Ville Valtavirta, and Toni Kaltiaisenaho, “The Serpent Monte Carlo Code: Status, Development and Applications in 2013,” Annals of Nuclear Energy, Vol. 82 (August 2015), pp. 142–50.
3 John Salvatier, Thomas V. Wiecki, and C. Fonnesbeck, “Probabilistic Programming in Python Using PyMC3,” PeerJ Computer Science, 2:e55 (April 2016), <https://doi.org/10.7717/peerj-cs.55>; Figueroa and Göttsche, “Nuclear Archaeology.”
Appendix
This appendix provides scientific details relating to the case study of the integrated approach presented in the article. It gives a concrete example of how information from a state’s declaration can be taken into account jointly with forensic measurements to reconstruct histories of fissile-material production. Specifically, an incomplete declaration of a past plutonium-production program is assumed. The case study assumes that a country has conducted an undeclared campaign that has produced plutonium that is 96 percent Pu-239 and that the country’s one declared campaign has produced plutonium with a somewhat lower Pu-239 content. (The higher the Pu-239 content, the better suited the material is for weapons use.)
Data on the declared reactor operation are taken into account, together with hypothetically measured isotopic concentrations of the high-level waste that is produced during plutonium separation. This information is used to reconstruct the complete operational history, including the undeclared operation. Furthermore, it is shown how the incorporation of declared information is valuable in reducing the uncertainty of parameters related to the undeclared activity. The appendix is based on previously published research.Footnote1
The problem
Scientifically speaking, reconstructing the operational history from measuring high-level waste is an inverse problem. The measured waste’s isotopic composition () could also be calculated using reactor simulations, assuming that the complete and true record of reactor operations leading to the waste was known. The record is the input to those simulations, codified as a set of parameters (
). In our case, it is precisely those parameters that we seek. The reactor simulations can then be thought of as a model that computes
, and we inversely know
, and seek
.
Besides the reactor and fuel geometry, which we assume to be known, includes operational parameters such as fuel burnup. Burnup is the energy extracted per fuel mass. With increasing burnup, the amount of produced plutonium increases, but the Pu-239 fraction decreases as more Pu-240 is being produced. By the time the waste is measured, many isotopes that were produced in the reactor have undergone radioactive decay. Therefore, the age of the waste, which we define as the time passed between production and measurement, must also be considered. It is these parameters, burnup and age, that we seek to reconstruct by measuring the waste.
The solution approach
We solve the inverse problem by applying Bayes’ theorem, which states that the probability that a specific set of parameters (burnup, etc.) has “caused” the measured (“observed”) waste composition is proportional to the probability of measuring a specific waste composition as the result of a specific set of parameters. The former (called “posterior”) are the parameters that we seek (that is, we seek those combinations of parameters with high probability) and are obtained by many repeated calculations of the latter (called “likelihood”). This approach is called “Markov chain Monte Carlo.” Roughly speaking, for many different combinations of input parameters, we calculate the resulting isotopic composition, which may be very different from those that were measured. After a number of calculations, we would find those input parameters that result in waste compositions similar to those that were measured.
With only a few measured isotopes—as the IAEA sampled in North Korea in the early 1990s—only limited information can be reconstructed. With more isotopes, more information can be reconstructed.
In this Bayesian framework, existing information (such as from a state’s declaration) can be included. In our case study, this information consists of the burnup and age parameters from the declared production campaign. Those can also be assigned an uncertainty, as the declared information may be uncertain or false. This is the so-called “prior” .
The prior and the likelihood are jointly taken into account when calculating the posterior: it is proportional to their product, . Note that the Bayesian approach could be applied very broadly for the integrated nuclear-archaeology approach, beyond this example based on waste measurements.
Implementation
To calculate , we created a model constructed using the reactor-simulation code SERPENT 2.Footnote2 As a reactor in this case study, we use a model of the Savannah River Site’s K Reactor’s inner core with a specific fuel design (called Mark 15, 1.1 percent uranium enrichment) using publicly available data (see ). The Savannah River reactors and the Hanford reactors produced the United States’ military plutonium.
Figure A1. Two-dimensional implementation in SERPENT 2 of Savannah River Site’s K Reactor’s inner core with the Mark 15 fuel design (left). Detail of the fuel element (right).
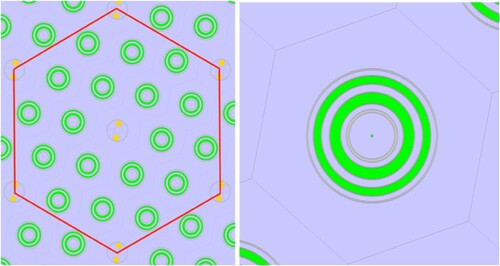
To calculate the posterior, we used the software package PyMC3.Footnote3 The isotopes to be measured in waste are cesium-137, europium-154, molybdenum-95, neodymium-142, and strontium-90, as they are sensitive to burnup and age. (This is a feasibility study. In an actual application, one would study ratios of isotopes as opposed to individual concentrations, and the list could be larger.) If this proof of concept can be demonstrated with individual isotopic concentrations, it should be equally feasible with isotopic ratios, as the underlying mathematical principles would remain the same.
The following scenario is simulated (see ). The declared reactor operation (“a”) 10 years ago has produced fuel with a burnup of 1.8 MWd/kg, corresponding to a Pu-239 content of 92 percent. The undeclared operation (“b”) occurred 30 years ago, and its burnup was 0.8 MWd/kg, corresponding to 96 percent Pu-239. Note that by reconstructing these two operations, we aim to distinguish two rather similar burnup values. The waste of these two operations has been mixed in the same waste tank, as illustrated in . As can be seen, an average isotopic composition is measured:
, where
is the mass ratio of both batches and is also reconstructed. The true value in our scenario is
= 40 percent. For each measured isotope in
, we assign an uncertainty of 5 percent that is considered in the calculation of the likelihood.
Figure A2. Illustration (in the form of vials) of mixing the waste from two different plutonium-production campaigns “A” and “B” in one waste tank. “Cooling time” refers to the age. Courtesy of Antonio Figueroa.
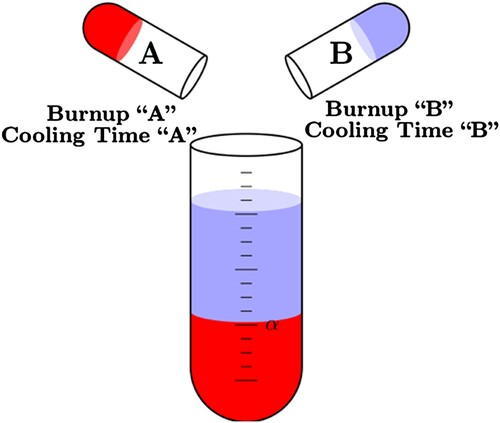
Table A1. Implemented priors used to reconstruct the plutonium-production history (whose true parameter values are also shown here) using Bayes’ theorem
In a first implementation, we assume no provided information. We implement this assumption by choosing a prior where all burnup values between 0 and 3 MWd/kg have the same probability (hence a larger range corresponding to no or limited knowledge), as do the age values between 0 and 50 years. In a second implementation, we assume the state’s declaration on the more recent campaign is correct. This is implemented as a prior describing the declared campaign where burnup values between 1.7 and 1.9 MWd/kg are equally likely, and production occurred 9 to 11 years ago. For each of the two implementations, we have used 40,000 repeated calculations.
Results
In all implementations, we can successfully reconstruct the burnup values, the ages, and the mass ratio, including those from the undeclared campaign. Interestingly, the uncertainties of the reconstructed values are lower when prior information is taken into account. shows the relative uncertainties of the undeclared campaign, when prior information is not included, compared with considering information on the declared campaign. By incorporating prior information, an uncertainty reduction of roughly 30–50 percent was achieved. shows the calculated posteriors for the implementation with a prior.
Figure A3. Posterior distributions for the declared campaign (“a”) and the undeclared campaign (“b”), as well as the mass ratio . Courtesy of Antonio Figueroa.
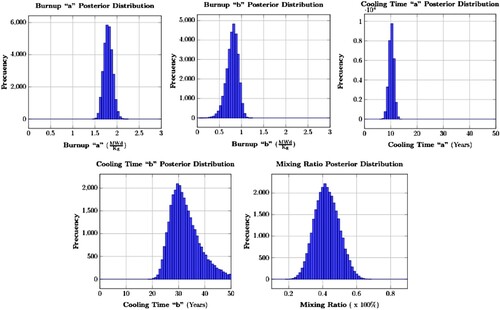
Table A2. Posterior uncertainties on the undeclared campaign
These results show the benefits of using several isotopes (isotopic ratios) and taking forensic information jointly with information from declarations into account. The five parameters could not be found by measuring just a few isotopes (as the IAEA sampled in North Korea in the early 1990s). While this is only an initial feasibility study, it is likely that more information can be reconstructed, and uncertainties be further reduced, by taking into account additional isotopes (isotopic ratios). However, further study is required to assess the potential and the limitations of this method, especially when applied to realistic (that is, more complex) programs, and to arrive at a general understanding of expected uncertainties beyond specific cases.