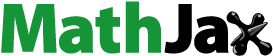
ABSTRACT
The propolis (PP) and linseed oil (LO) were encapsulated into the gum Arabic (GA) matrix along with whey protein concentrate (WPC) using the freeze-drying technique to form a hydrophilic matrix for production of functional pineapple snacks. The concentrations of the components in the matrix were optimized using response surface methodology (RSM) to maximize the protein content and encapsulation efficiency (EE). The encapsulation efficiency, protein content, color parameters (L*, a*, b* values), microstructure and texture properties of the snack containing optimum component concentrations were investigated. The glass transition temperature for the snack prepared with optimized ingredient concentration and components, oxidation temperature for linseed oil were estimated using a differential scanning calorimeter (DSC). The results revealed an encapsulation efficiency of 83.15% and a protein ratio of 31.53% for the developed matrix. Gum Arabic did not increase the encapsulation efficiency. The use of WPC has increased the encapsulation efficiency and protein content. Linolenic acid (50.804%), oleic acid (22.443%), and linoleic acid (14.012%) were the major fatty acids in the snack, owing to the highly encapsulated linseed oil and propolis. These result data support the freeze-dried pineapple snacks, which may be optimized for their component ratios and then used as a functional product suitable for consumption owing to its high nutritional value.
Introduction
Daily consumption of nutrients in adequate quantities is essential for both physiological and mental health. Fruits and vegetables are important components of a healthy nutrition program as these provide adequate levels of dietary fiber, vitamins, minerals, and antioxidant components for the human body. The World Health Organization recommends consuming a minimum of 400 g or five meals of fruit and vegetables each day[Citation1]. In addition to the consumption of fresh fruits and vegetables, these may also be consumed in the form of dried and frozen products, juice, jam, or marmalade.[Citation2] Such products allow for consuming fruits and vegetables even in the seasons when these are not available. Several fast-food products have associated health risks due to their high glycemic index and unhealthy fat sources. Recent reports have linked several diseases, such as high insulin resistance, obesity, blurred brain, and diabetes, to unhealthy and dietary fiber-poor diet regimes.[Citation3] On the other hand, increased understanding of nutrition, awareness regarding healthy growth and development, and anti-aging efforts have led to the emergence of healthy, fruit-based food alternatives that are rich in dietary fiber and functional properties.[Citation4] The improvement in healthy fruit-based snacks is becoming part of vital change, such as health management and weight control. Products containing high protein, fiber and low energy have started to attract attention in fast living conditions with formulations that can replace meals. These meal alternatives are gaining increasing attention among consumers who follow special diets such as blood glucose level control.[Citation5]
Potential health effects such as vitamin C and antioxidant biocomponents contained in fruits provides the opportunity to enrich the healthy snacks produced with appropriate formulations.[Citation6,Citation7] Production of fruit by-products other than raw fruit in the form of bars or snacks with a rich nutritional content by using gelling agents or hydrocolloids also offers the opportunity to develop sustainable innovative products. These products, produced in enriched form, provide a high level of microbiological safety without the use of any chemicals.[Citation8] The fruit products or byproducts in gel or foam structure can be consumed with enrich nutritional value which can become a part of the basic meal in the form of a snack or bar.[Citation9,Citation10]
Pineapple (Ananas comosus L.) is the most popular tropical fruit from the family Bromeliaceae.[Citation11,Citation12] This fruit is rich in minerals (magnesium, calcium, phosphorus, and iron) and fiber and is a great source of antioxidants, including phenolics, flavonoids, and ascorbic acid.[Citation13,Citation14] Pineapple is among the most preferred fruits in the world owing to its sensory characteristics and structural features along with a good sugar – acid balance.[Citation15]
Propolis is a natural antioxidant and antibacterial source collected from plant sources by honey bees.[Citation16] Recently, propolis has attracted attention as a functional food ingredient because it is rich in phenolic components, contains essential fatty acids, has anti-microbial, antioxidant properties and is seen as a natural preservative. Its anti-carcinogenic,[Citation17] anti-inflammatory,[Citation18] anti-aging[Citation19] effects encourage its addition to foods to provide functional gain, which is important for health. However, its use as a food additive is limited due to its solubility, thermal and oxidative stability. Many techniques have been used for use in foods to improve their functional properties by masking their negative sensory properties. Thermal encapsulation methods, which are among these techniques, include methods such as vacuum, freeze drying and spray drying.[Citation20] Each of these processes aims to minimize microbial growth and enzymatic change by ensuring a controlled removal of free moisture from the matrix,[Citation21,Citation22] which also simplifies the storage and transport of the products due to weight reduction.[Citation23,Citation24] Encapsulated form supported by biopolymers is used for enrichment in freeze dried food production in terms of fatty acids and transition elements.[Citation25]
Freeze-drying technology is applied for the removal of water contained in frozen food through sublimation. This drying method removes moisture by creating collapses in the intercellular spaces, while the size of the food product remains unchanged,[Citation26,Citation27] which is why shrinkage or volumetric reduction is less common when using this technique compared to other techniques.[Citation28] The main advantage of this technique, despite its requirement for a long processing duration, is that this method leads to no adverse impact on the appearance of the fruit and its nutrient components during the moisture removal process under a strong vacuum.[Citation29] The new matrix formed after the process creates a fracturable, lighter-colored product structure compared to the product prior to drying. Texture properties, such as fracturability, gumminess, and hardness, have been reported in freeze dried fruits.[Citation30–33]
In the drying process, collapses occur at temperatures just above the glass transition (Tg) temperature, and this is when the product structure is shaped. The amorphous system transforms from a glassy to a rubbery state at the glass transition point (Tg), which is defined as the temperature at which deterioration occurs under heat treatment conditions.[Citation34] In order to increase the rehydration ability in terms of functionality and to eliminate the defects in the texture of the fruit-based products, it is important to shape these products into desired structures. The products with the desired form may be prepared by incorporating high-molecular-weight polymers in the freeze-dried fruit-based products. Gum Arabic (E 414) is among the high-molecular-weight carbohydrates used as stabilizer, and emulsifier with the effect of reducing hygroscopicity, viscosity and anti-caking. Furthermore, it contributes significantly to storage stability by increasing the glass transition temperature. The use of biopolymers in high concentrations reportedly increases the gumminess as well as the cost of freeze-dried products.[Citation35] Moreover, the encapsulated matrix formed by the proteins with hydrophobic characters is in the form of a covering similar to a film, which confers it with a rehydration ability that exerts the positive effects of good texture and sensory acceptability.[Citation36] Better texture properties are reportedly obtained using amphiphilic protein additives in appropriate proportions.
This present study explored the possibility of formulating a pineapple based fruit snack rich in protein, essential oil. Appropriate concentrations of different biopolymer combinations were optimized using RSM (response surface methodology). The effects of the biopolymer support used on the physicochemical, texture, microstructure, and thermal properties of the freeze-dried snacks prepared using the pineapple fruit were determined.
Materials and methodology
Materials and chemicals
Fresh pineapple (Ananas comosus L) fruits obtained from the local market (Antalya in Turkey) were used in the present study. The whey protein concentrate (WPC, Arla foods, Denmark), gum Arabic (GA, Sigma-Aldrich Chemie GmbH, Eschenstr. 5, Germany), propolis (PP, from the local market), and linseed oil (LO, from the local market) were used as the encapsulation agents. All chemicals used (Merck KGaA, Darmstadt, Germany) in the present study were of analytical grade.
Sample preparation and the drying process
In order to prepare the desired pineapple snacks, the pineapple fruits were first washed, followed by peeling with a stainless steel knife and then preparing the pineapple puree using a food processor (Arçelik, K 1261 RHB, Turkey). The gum Arabic (as biopolymer), propolis, linseed oil (as encapsulation agents), and whey protein concentrate (WPC; as the conditioner) were mixed with the pineapple puree in proportions according to 27 different recipes (). The lower GA and higher WPC ratios were distinguished than the amounts investigated in previous studies in order to minimize the aforementioned adverse effects.[Citation37] The mixtures were prepared according to the recipes and then frozen using cubic silicone molds (1 × 1 cm) at −20 ± 2°C for 24 h. The frozen snack cubes were freeze-dried at −43°C for condenser temperature, at + 46°C for the final drying at a flow rate of 6 CFM and a pressure of 0.44–2.8 MPa using a freeze dryer (Harvest Right, LLC. China). The freeze drying parameters were optimized in the preliminary studies (Data not shown). Physico-chemical analyzes of all prepared mixtures before the freeze-dry process are listed in . The freeze-dried pineapple snacks were stored in oxygen and water-proof kraft aluminum ziplock doypack packages at 4 ± 2°C until to be used for laboratory tests.
Table 1. Initial moisture content and color properties of the pre-dried pineapple snacks.
Experimental design and data analysis
The mathematical relationship between the process input parameters and the measured performance characteristics of the product was modeled based on the regression equation, and the model was optimized to determine the factor values that would produce the desired outputs. The response surface method (RSM) was adopted for optimization. Two response variables – the protein amount and the encapsulation percentage – were measured, and the regression equation for these responses was established (EquationEquation 1(1)
(1) ):
In the above equation, X1, X2, X3, and X4 denote the amounts of whey protein concentrate, gum Arabic, propolis, and linseed oil, respectively (); β0, β1, β2, β3, and β4 are the constants of the regression coefficients from the linear, quadratic, and interaction effects, respectively; Y represents the response estimated from the model.
Where the predicted model was subjected to the analysis of variance (ANOVA) using P < .05 as the significance threshold in Minitab® statistical software (Pennsylvania, USA). The fitting of the model was determined based on the P-values of the lack of fit and the regression coefficient (R2). The optimal condition for each response surface was determined using 3D plots. The optimal condition was validated for the maximization of the protein amount, encapsulation percentage, drying efficiency, and the minimization of the moisture content and shrinkage. The model validation was performed by comparing the response surface data obtained from model prediction with the experimental data obtained for the sample under optimized conditions.
Moisture content
The moisture change between the pre-dried and freeze-dried samples was determined. The mixture samples (weighing approximately 3 grams) were placed in meter cups and then maintained inside a vacuum oven (Nüve EV018) at 70°C and 600 mm-Hg vacuum until a constant weight described by ICC No: 110/1[Citation38] was reached. The following equation was used for calculating the dry basis moisture content (MCd) (EquationEquation 2(2)
(2) ).
Where, Ww denotes the weight of the water; Wd denotes the weight of the dry matter.
Volume reduction rate
When determining the shrinkage value, the methods based on liquid volume displacement did not produce a reliable measurement due to the microstructural properties of the pre-dried and freeze-dried cubes. Therefore, the volume reduction rate (VR) of the pre-dried and freeze-dried snacks with a uniform geometric structure (cubic) was calculated mathematically (EquationEquation 3(3)
(3) ). The three dimensions (cm) of width (a), length (b), and height (c) were measured for all samples. The volume was calculated in cm3 using EquationEquation 3.
(3)
(3) The percent volume reduction was calculated using EquationEquation 4.
(4)
(4)
Where, V1 and V2 denote the volume of the frozen snack and the freeze-dried snack, respectively.
Apparent density, true density and porosity
True and apparent density, porosity of pre-dried and freeze-dried cubes were determined in order to clarify microstructural properties. The true density of powdered snacks was determined using a stereopycnometer (MVP-1 multipycnometer, Quantachrome, Boynton Beach, FL), with helium at room temperature. An electronic balance with accuracy of 10−4g was used to measure of total mass repeated three times. The apparent density was calculated using ratio of total mass to volume from collected data for (shrinkage) volume reduction rate (EquationEquation 5(5)
(5) ).[Citation39]
EquationEquations (5)(5)
(5) , (Equation6
(6)
(6) ) were used for calculating the remaining physicochemical properties; m denotes the mass, V denotes the total volume of the freeze-dried snacks (cm3),
denotes the porosity,
denotes the true density (g/cm3),
denotes to apparent dencity respectively, of the freeze-dried pineapple snacks.[Citation39]
Protein content
The protein content (PR) of the freeze-dried samples was analyzed as described by Nielsen using nitrogen-protein analyzer (NDA 701, VELP China Co. Ltd.).[Citation40] First, the instrument was calibrated for the mass of a particular nitrogen content value. Afterward, 0.5 g of the freeze-dried sample was combusted at 800°C and 900°C under oxidative conditions. The released gases (carbon dioxide, water vapor, and nitrogen) were passed through the columns of potassium hydroxide. The residual nitrogen was measured by separating nitrogen from water and carbon dioxide using a thermal conductivity detector, and the value obtained was considered the nitrogen content. The crude protein content was calculated using conversion factors that relied on the measured nitrogen content.
Encapsulation efficiency
The modified method was used to evaluate the combined encapsulation efficiency (EE) of propolis and linseed oil.[Citation41] Firstly, 1.5 g of the homogenized freeze-dried snack sample was placed in a cup, to which 15 mL of hexane was subsequently added. The mixture was vortexed for 2 min and then filtered using coarse filter paper. The retentate was washed three times with 20 mL of n-hexane to dissolve the residual oil. The solvent was evaporated in a rotary evaporator at 90°C (LabFirst Scientific Instruments, Shanghai, China) and oil phase was weighed. The surface oil (SO) was determined by the difference between the initial weight of the free flask and the weight of the flask with oil phase. The oil initially added in to the snack formulation were considered as total oil (TO). EE% was calculated by the following equation:
Texture and microstructure
In order to determine the texture properties of the freeze-dried snack samples, the maximum tolerable force was measured as fracturability. The force peaks were obtained using a texture analyzer (TA-XT, Texture Technologies Corp.; UK), and the first compression was considered fracturability. Owing to the fragile structure, the process for the second compression could not be performed.
The microstructure properties of the freeze-dried pineapple snacks were examined using a scanning electron microscope (SEM) (Quanta, 250 FEG FEI co.; Netherlands). In order to obtain sharp SEM images, the sample surfaces (thickness 0.1 cm) were coated with an extremely thin layer of gold.[Citation42]
Color
The color measurements were performed for the pre-dried and freeze-dried snack samples using the colorimeter device (LC100, Lovibond, Maharashtra, India). The color parameters evaluated included lightness (L* value), redness (a* value), and yellowness (b* value). The white tile was used for calibrating the instrument as instructed by the manufacturer.[Citation43] The other color attributes, such as chroma (saturation) and Hue0 angle, were evaluated using the following equations:
Thermal aspects
Thermal characteristics of freeze-dried snack with optimized composition and components of mixture (LO, WPC, GA, PP, pineapple) were estimated using a differential scanning calorimeter (DSC) (Hitachi High-Tech Sciences DSC7020, Japan). Firstly, the DSC was calibrated using indium (Tonset = 155.74°C and ΔH = 28.69 J/g). Approximately 5 mg of the sealed sample was placed, in triplicate, in the DSC pans, hermetically, while an empty pan was used as the reference. First step was cooling sample at the rate of 10°C/min to bring down the sample temperature to −60°C from the temperature 25°C. And them at the same rate of 10°C/min the sample was heating to 250°C in nitrogen atmosphere. Sample of 5–10 mg was measured in 40 μL aluminum crucibles without a lid in order to estimate of the oxidation temperature (OOT). The OOT measurements were performed by heating the samples from 30 to 250°C at 5°C/min to determine for Ton (onset temperature), Tpeak (middle of the oxidation), Tend (end of oxidation). Firstly, samples by heated 30 to 35°C using nitrogen gas. Then, 35 to 250°C at 5°C/min using oxygen as purge gas.
Fatty acids
The fatty acids were identified using the Agilent 7890 A series gas chromatograph (Gerstel GmbH & Co. KG, USA) with a silica capillary column (DB Wax 50 mm x 0.20 mm; i.d. 0.20 µm; Agilent Technologiepoliuns, Inc.; USA) and a flame ionization detector (FID).[Citation44] An isothermal column temperature of 240°C was used, while the temperature of the injector and the detector was 240°C. The volume injected was 1 µL. The fatty acid components were analyzed and computed as a percentage using the MSDCHEM software.
Sensorial aspects
The sensorial aspects of the produced pineapple snacks were evaluated in the form of with trained panelists (n = 100, aged 18–57, 98 male), describing to the quantitative descriptive analysis (QDA) method and ISO 13,299:2016 standard.[Citation45] Profile was characterized by taste (fruity, milky, oily, sour, metallic, lasting, rancidity), texture (brittleness, slickness, smoothness, chewiness, stickiness, oiliness, stringiness, sandiness, intraoral spread) and general appreciation. The intensity of those qualifications were rated on a hedonic scale from “0 = imperceptible” to “5 = very intense.” The samples were coded (three-digit) by labeling randomly. The samples were randomly presented to the panelists according to the experimental design established by applying the randomized block design (RCBD).
Results and discussion
Fitting of the model
presents a few physicochemical and functional properties of the pre-freeze pineapple snacks obtained using 27 different recipes with different component concentrations. The effects of the independent variables on the encapsulation efficiency (EE) and protein ratio are presented in . The interaction between dependent and independent variables was modeled using the quadratic polynomial equation (EquationEquation 1(1)
(1) ). The data revealed that the fitted model could be used at a 95% confidence level to predict the response surfaces of the three independent variables (). The quadratic polynomial equation was used for modeling the interaction between the dependent variables WPC, GA, PP, and LO and the independent variables EE and PR. In addition, the fitted model could be used at a 95% confidence level to predict the response surfaces for the two independent variables. The values obtained using the analysis of variance (ANOVA) of the polynomial functions, the regression equations, and the regression coefficients between the response variables and the independent variables are presented in . The coefficients of determination (R2) obtained using the model equations for all the response variables were in the range of 0.9395–0.9950 (considerably close to 1). Therefore, the equations could successfully represent the experimental data.
Table 2. The physicochemical, color, and functional properties of freeze-dried pineapple snacks.
Table 3. Adequacy of the fitted models for the pineapple snacks.
Table 4. The P-values and the regression coefficients of the main, quadratic, and interaction effects of different variables in the final reduced models fitted for pineapple snacks.
The P-values of the quadratic equations defined for all the response variables were significant (P < .05), which implied that the model equations could suitably represent the experimental data. In the absence of the fitting test, which would otherwise have indicated the effect on the success of the data that were in the experimental dataset and not in the regression dataset, the P-value was expected to be insignificant (P > .05).
The real systems were adopted to determine the adequacy of approximation of the fitted models for protein ratio and encapsulation efficiency. The adequacy of the models was determined using residuals from the fit of least squares. The normal probabilities were plotted using the residuals against the normal present probability for the protein ratio and encapsulation efficiency of the freeze-dried pineapple snacks (). The residuals from the responses formed a linear line in the plots ().
Encapsulation efficiency and the physicochemical properties
depicts the chromatograms, and presents the retention times of fatty acids in the pineapple snacks containing optimum component concentrations (). Since freeze drying is used as one of the encapsulation methods, it is desirable to have a high encapsulation efficiency. In the present study, the encapsulation percentages of the freeze-dried pineapple snacks varied between 35.18% and 83.15% (). The optimization of the drying conditions is essential in the field of food-drying technology. The relationship of the percentage of encapsulation with the amount of protein, gum, propolis, and oil amounts was investigated in the present study using the Minitab® statistical software (Pennsylvania, USA). The result data revealed a weak positive correlation between the protein content and the encapsulation efficiency (EE). In addition, the encapsulation efficiency exhibited a weak negative correlation with the gum content and a weak positive correlation with the propolis ratio. A slightly strong and positive correlation between the encapsulation efficiency and the oil content was observed (). A strong and positive correlation between the encapsulation efficiency and the WPC content was observed (p < .01). The predicted protein and encapsulation values were 37.64% and 83.39% for the sample with optimum component concentrations (), while the corresponding experimental data values were 33.44% and 68.68%, respectively (). Moisture content is a factor that effectively determines the drying efficiency and the shelf life of dried products. The main principle exploited in the drying processes is to extract the maximum amount of water from the food.
Figure 3. The chromatograms from the GC-MS analysis of the freeze-dried pineapple snacks containing optimum component concentrations.

Table 5. The retention times of fatty acids in freeze-dried pineapple snacks.
Table 6. Optimum encapsulation conditions and responses of the freeze-dried pineapple snacks.
Table 7. The effects of the freeze drying (FD) method on the physicochemical, color, and functional properties of the pineapple snacks prepared under optimum conditions.
Pineapple puree 12 °Brix was used to obtain freeze-dried pineapple snack. The initial moisture content of GA, PP and WPC were 6.83, 4.62, 7.76% respectively. The moisture contents of the prepared mixtures and the dried products are listed in , respectively. The MCd of the 27 mixtures (27 different recipes used) were compared statistically using the Minitab® statistical software (Pennsylvania, USA). Significant decreases (p < .05) were observed in the moisture contents of all evaluated mixtures. The essential fatty acid amounts, color properties and some physicochemical properties of the freeze-dried pineapple snack containing optimum component concentration prior to and after the drying process modeled to maximize the encapsulation efficiency with protein ratio are presented in .
The volume reduction rate of the freeze-dried snacks varied between 6.22% and 13.03% (). The experimental value obtained at the optimum conditions using the model approach was 8.2% (). The 3D plots depicting the optimum values for the protein content and the encapsulation efficiency of the freeze-dried pineapple snacks are presented in , respectively.
Figure 4. The surface plots for the protein content of the freeze-dried pineapple snacks under the effect of the drying conditions of WPC and GA (a), WPC and PP (b), WPC and LO (c), GA and PP (d), GA and LO (e), and PP and LO (f).

Figure 5. The surface plots for the encapsulation efficiency of the freeze-dried pineapple snacks under the effects of the freeze-drying conditions of WPC and GA (A), WPC and PP (b), WPC and LO (c), GA and PP (d), GA and LO (e), and PP and LO (f).

Freeze drying is a safe encapsulation technique for unstable aqueous solutions and heat-sensitive biocomponents.[Citation46] Among the freezing, sublimation, and drying methods used for encapsulation, freeze-drying is quite effective in terms of the appearance properties, such as shape and color, while also resulting in positive sensory effects in terms of flavor, aroma, and texture properties.[Citation47] Gum Arabic, whey hydrolyzate, starch, and maltodextrin are used widely in the formation of the structure as an amorphous matrix. In the freeze-drying process, the amorphous structure with high porosity forms a barrier with the active component,[Citation48] which explains the higher storage stability of freeze-dried products.
Encapsulation efficiency is an important parameter that affects the ability of active ingredients to be preserved and actively used.[Citation49,Citation50] presents the effects of the GA, PP, and LO proportions on the encapsulation efficiency of the protein in the freeze-dried WPC molecules. The results did not reveal a significant change in the encapsulation efficiency with the increase in the concentration of WPC, GA, PP, or LO proportions. Although GA is known as a stabilizer that increases emulsion stability. However, no significant effect was observed since lower GA concentrations used for content optimization could not create the desired synergy with drying stress at low moisture content. For all that, owing to the synergy among the concentrations of WPC, GA, PP, and LO, the highest encapsulation rate of 83.15% was achieved for the sample containing 16% of WPC, 0.15% of GA, 0.12% of PP, and 15% of LO (P < .01). WPC has been used in order to reduce the fracture resistance by improving the texture properties and to create an amorphous structure that will allow the improvement of the texture properties together with Gum Arabic in addition to increasing the protein content. The increase in WPC concentration had a significant effect (P < .001) on the protein ratio compared to the concentration of the other components (). In particular, the increase in LO concentration had an increasing effect on the encapsulation efficiency.
The main reason for using linseed oil with high encapsulation efficiency in the present study was the objective of producing snacks rich in essential polyunsaturated fatty acids, particularly linolenic, linoleic, and oleic acids. It was evident that the freeze-dried pineapple snacks containing an optimized amount of linseed oil presented a considerably higher concentration of linolenic acid compared to the other unsaturated fatty acids (). The content of saturated fatty acids was determined to be quite low compared to the unsaturated fatty acids (). The reported linolenic acid content in linseed oil is in the range of 39.90%–60.42%.[Citation50–52] The contents of linolenic, linoleic, and oleic acids in the pineapples at different levels of maturity were reported to be in the ranges of 1027.9–1299.9 µg/g, 0.409047–1.4893 µg/g, and 2.3335–5.3592 µg/g, respectively.[Citation53] The freeze-dried snacks produced with a high encapsulation efficiency under the specified optimization conditions could, therefore, be used as a functional food alternative with good content of essential fatty acids ().
In comparison to the other drying methods, freeze-drying is characterized by a matrix that develops with lower shrinkage or volume reduction and has further visible pore formation. The volume reduction rate, also referred to as shrinkage, in the freeze-dried pineapple snacks prepared in the present study (moisture content range: 0.7% − 2.4%) was determined to be 6.22%–13.03%. It is reported that sorption isotherms, which develop due to changes in the water activity, are effective in reflecting the matrix shrinkage properties of freeze-dried mixed vegetable snacks prepared using a hydrocolloids.[Citation54] In addition, the polymer supports reportedly cause remarkable changes in the sorption properties of freeze-dried mix-fruit products. Moreover, using a hydrocolloid matrix in the production of fruit bars or snacks would lead to better results due to the formation of a porous skeleton that would serve as a carrier structure for other food ingredients. However, it is reported that the shrinkage or decrease in the volume is greater upon freeze-drying due to the high sugar content of the fruit products.[Citation55]
Color attributes
The color data measured for the undried and dried mixtures are provided in , respectively. The differences between the L* (lightness) and b* (yellowness) values were statistically significant (P < .05). The L* and b* values of all 27 mixtures were observed to increase after freeze-drying. The L* (lightness) value decreased as the WPC proportion increased in the composition of pre-freeze snacks (). However, the different proportions of hydrocolloid and amorphous substances in the mixture altered the L* value together. While the L* value increased in the samples produced without using GA alone, the L* value increased significantly when GA was not used together with PP. Significant decreases occurred in the L* value when GA and WPC were not used together. It was evident that the change in the L* value was affected by the use of WPC. On the other hand, when LO was not used in the samples, the L* value decreased when GA was not used and increased when it was used. It was determined that freeze drying has an effect of increasing the L* value of the product (). Increased brightness has often been expressed in freeze dried fruit- or vegetable-based products [5,7,8,24,28]. In the freeze-drying process, the surface porosity and microbubbles just below the particle surface with the brighter reflection lead to a lighter appearance of the product.[Citation56] In the present study, a* value (greenness) was within the limits of the redness value prior to the drying process, it gradually increased after the freeze-drying process (). This change, which occurred due to the sublimation effect caused by the pressure (vacuum), manifested as the transition from the amorphous structure to the glassy structure formed by the different hydrocolloid and protein structures together.[Citation57] Ciurzyńska et al. (2022) have been reported brightness of freeze-dried fruit bars designed fruit gels consist of apple pulp, apple juice, water, sodium alginate and chokeberry pomace were decreased due to the increase in pomace concentration.[Citation54]
Textural and microstructure characteristics
The fracturability of all the freeze-dried pineapple snack prepared using optimum component concentrations was analyzed. depicts the texture curves for the measurements performed in triplicate. The measurements for springiness, resilience, chewiness, cohesiveness, and adhesiveness could not be performed due to the fracturable structure of the material. But hardness measured as 33 ± 2.05 N. The expected fracture strength could not be obtained at the used concentrations of the amorphous components.
Figure 6. An example of the force – time curves of freeze-dried pineapple snacks (evaluated in triplicate).

The force-time curves indicate the increase in force as a measure of the deformability of the samples. The excess of the curve slope indicates the high deformability. The first peak is the point where deformation continues as the fracture or yield point. After this point, while the fracture continues on the inner surface of the sample, there is no increase in force as long as the fractured sample was compressed. A noticeable increase in force was observed at the fracture point. Polymer supports are added to reduce fracture behavior at low porosity. Egas-Astudillo[Citation58] reported that the polymer supports allowed to obtain freeze-dried fruit products with low deformation properties in the glassy state and more resistant to breakage.
The application of the drying method to fruits affects the existing porosity of the product structure as well. According to the drying method used, the water forms “collapses” while leaving the fruit matrix or causes tissue damage due to the deformation of the cell membrane. Therefore, the porosity is also affected during drying. In the production of functional snack by freeze drying, firstly the freezing process and then sublimation affected the microstructure properties and especially porosity. Increasing GA and WPC concentrations showed a decreasing effect on porosity while ratio of PP were constant. On the other hand, decreasing concentrations of PP affected the porosity linearly (P < .05) ().
In addition to the direct effect of the drying method, the porosity of the freeze-dried fruits is also affected by the amorphous structures formed in the dried fruit products. It is reported that the use of drying agents causes high porosity by trapping the air between molecules as a result of intermolecular interaction.[Citation59] However, the use of gum Arabic was characterized by lower porosity as well as higher tapped density in the production of freeze-dried lemon beverage powder.[Citation60] The porosity values expressed showed lower porosity values than the pineapple snack product obtained in our research due to the powder particle structure of the product.
The SEM images of the pineapple snacks containing optimum component proportions are depicted in . The different-sized SEM images display polygonal cellular spaces (), and no evident collapse formations (). The porosity of the spongy texture observed in the freeze-dried products was evident. Amorphous structures with prominent glassy structures were distinctly visible.
Figure 7. Scanning electron microscopy (SEM) images of the freeze-dried pineapple snack containing optimum component concentrations a) 10 µm, b) 50 µm, c) 200 µm, d) 300 µm, e) 200 µm (another field of view), and F) 300 µm (another field of view).

In most studies on freeze-dried ready-to-eat foods, the energy requirements for the crushing and grinding processes are investigated.[Citation61,Citation62] This is necessary for determining the possibilities of processing the raw material into different products and also for evaluating storage safety. The product texture is evaluated to confirm efficient grinding in the case of powdered freeze-dried products,[Citation63] while hardness or fracturability is evaluated in sliced or biopolymer-supported snacks.[Citation64] The structural properties of the biopolymer support used in the pineapple snacks, i.e.; the optimum encapsulation and the protein ratio, were measured using the maximum force method and characterized based on repetitive force-distance curves. The fracturable matrix of the pineapple snacks did not allow the application of further force. Similarly, the mechanical behavior of the freeze-dried orange snacks with different biopolymer supports was investigated, and greater fracturabilty was reported at low water activity levels.[Citation65] It is reported that porosity leads to increased fracturability. In the present study, although large pores were rarer, small pores and prominent cavities were identified in several places ().
In the process of freeze-drying pureed fruit products, the structure becomes highly porous due to the dispersed gas bubbles leaving their places in the cavities. In the ice formation stage, the pores become increasingly larger due to sublimation. Consequently, two different pore structures are formed, leading to a spongy texture. Traces of the spongy tissue were visible despite the changes in the amorphous structure of the freeze-dried pineapple snacks [the condenser temperature of −43°C used for freezing] containing 0.0 g of gum Arabic, 16 g of WPC, 0.24 g of PP, and 8.78 g of LO (). The spongy structure had slight fractures. In the drying process, the molecular structure changes due to the formation of new components or changes in the structures of the existing components. The variation differs greatly between amorphous and crystalline structures. Molecular differences in hygroscopicity, solubility, and moisture absorption behavior lead to the characterization of texture formation. Amorphous polymers are preferred as encapsulating agents for perfect modulation of the viscosity of dried foods. The loss of the volatile and aroma components may be prevented through the formation of the glassy structure. Crystal structures formed in this manner remain stable without being affected by relative humidity and temperature. While caking is not observed at relative humidity values until the melting point, partial recrystallization is observed.[Citation66] Unlike crystalline structures, amorphous structures are used to changes in viscosity with a gradual increase in temperature. On the other hand, crystalline structures transition from solid to liquid via energy transfer at a constant temperature.
Amorphous materials with their specific surface area may get greater moisture as the relative humidity increases. Unlike highly polarized amorphous structures, crystalline structures absorb negligible amounts of water except for their own crystalline water. The transition of moisture into a crystalline structure occurs layer by layer,[Citation67,Citation68] which increases the stability of the crystal structures during applications. Since amorphous structures retain moisture inside, partially porous structures cause them to gain a larger volume puffing during the transition from high pressure to vacuum during freeze drying.[Citation69] The volume increase is realized based on these pressure changes in the desired texture. The formation of visible collapses in freeze-dried pineapple products has not been reported previously in any of the studies.[Citation70,Citation71]
Thermal characteristics
The thermal characteristics of the components and the pineapple snack containing optimum component concentrations during heating at the rate of 10°C/min are depicted in . presents melting points (Tmon, Tmpeak, Tmend) for LO, PP, GA and the sample with optimum component concentrations. The glass transition temperature (Tg) of pineapple snack had the optimum concentration of ingredients, denaturation temperature for WPC and oxidation onset for LO have been also represented in . For LO, the exothermic peak in the range of 160°C to 250°C related to auto-oxidation process of unsaturated fatty acids was observed. According to DSC curve, onset temperature at which the auto-oxidation process begins was determined. The onset temperature is usually taken as a parameter characterizing the oxidative susceptibility of oils. For GA, the endothermic peak means crystallites melting during the heating. Endothermic peak range is 36.1°C − 216.9°C. For WPC, endothermic transition peak on heating shows that denaturation endotherm. Denaturation peak center of WPC was 88.4°C. For PP, first endothermic peak defines as characteristic of the wax (present in propolis) and second endothermic peak defines as decompositon of propolis substances. Melting point (1st peak) of propolis wax contained in propolis, Tm range was 27.3°C- 71.6°C. Decomposition temperature (Td) was 110.2°C ().
Figure 8. DSC thermograms during heating at the rate of 10°C/min of the a) LO, b) the pineapple snack containing optimum component concentrations, C) pineapple.

Figure 9. Radar diagrams of the a) texture profile b) general geometric acceptability characteristics, c) taste profile of olive snacks (n = 100).

Table 8. The thermal characteristics as Tmon: melting onset temperature, Tmpeak: melting peak temperature, Tmend: end of melting peak temperature, Tg: glass transition temperature of the evaluated factors and the pineapple snack containing optimum component concentrations during heating at the rate of 10°C/min.
The glass transition temperature (Tg) is a state transition that develops over a certain temperature range, characterized by the glass transition onset (Tgo), midpoint (Tgm), and endpoint (Tge). In most studies, Tgm is considered equivalent to the Tg point. However, the physical state change related to the transition may not start with the beginning of the transition and continue until the amorphous matrix acquires a rubbery structure at the end of Tg. Therefore, Tgo and Tge do not offer the same physical properties in every product.[Citation72] While the sample with optimum component concentration is in full glassy state at temperatures below −22.4°C, it will have a rubbery at temperatures above + 1.4°C. Such an expected effect from the use of WPC at low concentrations in lower moisture content (1.90 g/100 g). In the glassy state, the product is more brittle and shows a higher stability. The rubbery state means that the rate of deterioration reactions increases and the crispness disappears. However, it is thought that PP and LO, albeit weakly, contribute to this change in using concentrations (). A higher than expected melting point was determined at the optimum ingredient concentration of the freeze dry pineapple snack. This situation, which affects the stability of the solid product, is a factor that may pose a risk in terms of moisture absorption, especially due to the difference between the glassy transition. The glass transition temperature provides information regarding the highest temperature at which drying would occur during freeze-drying. The microstructure and the functional properties of freeze-dried foods are a result of the initial component concentrations and the glass transition (Tg).[Citation73] The softening of the polymer structures in the dried products is characterized by the glass transition (Tg). The glass transition temperature decreases as the humidity increases.[Citation74] With the increase in the moisture content in the freeze-dried fruit and vegetable products with biopolymers, the glass transition point decreased due to the plasticizing effect of moisture.[Citation75] The melting temperature decreased with the drying effect was reportedly caused due to freeze-stress.[Citation76]
Sensorial aspects
In the study, two focuses, namely taste and texture, which determine the sensory characteristics, were emphasized (). As expected, the fruity was dominant among the taste properties. However, rancidity, another focus of the study, was evident in some samples. Positive and weak correlation between rancidity and WPC, GA, PP was determined (p > .05). A positive and moderate correlation was determined between linseed oil and rancidity. Due to the oxidation sensitivity of the flaxseed oil and propolis additive, the change in taste showed a decreasing effect on the general appreciation. The contribution of WPC and GA increased the lasting taste and general appreciation. WPC additive has a positive effect on slickness, smoothness, chewiness and intraoral spread (P < .05). On the other hand, it had a negative (P > .05) effect on brittleness, stickiness, oiliness, sandiness and stringiness. The use of gum Arabic showed a weak positive effect in terms of brittleness and oiliness (P > .05). Especially negative correlation in terms of sandiness is evaluated positively in terms of brittleness in freeze dry products. The weak effect is thought to be due to the low doses used in gum Arabic. Valentina et al.,(2016) have been reported that flavor and aroma properties of different food groups can be perceived without loss and intense after freeze drying, and negative aroma development develops depending on the components.[Citation77]
Conclusion
In order to produce a healthy and easy-to-consume fruit snack, a pineapple-based food product was prepared using freeze-drying, and the structural, physicochemical, and thermal properties of matrix, which was formed using gum Arabic and whey protein concentrate, were investigated. Linseed oil, a well-recognized source of healthy fat, was encapsulated in the product to provide linolenic and linoleic acids, which are essential polyunsaturated fatty acids, in the final product. The matrix was formed using whey protein concentrate and propolis to increase the encapsulation efficiency. In addition to calculating the encapsulation efficiency, it was demonstrated using the fatty acid analysis that the polyunsaturated fatty acids from the incorporated linseed oil and propolis were entrapped. Microstructural features were visualized by the forms of encapsulated oil forms in the matrix. In addition to determining the functional properties of the whey protein concentrate, linseed oil and propolis used, the optimum component concentrations were estimated using RSM for the maximization of these components. Moreover, the experimental data were compared with the estimated data in terms of the healthy components of the final product produced. The snacks produced in the present study were also observed to have a tendency to high porosity and shrinkage at low moisture levels. In regard to the changes in the thermal properties with the moisture content in the structure, it was determined that the pineapple snacks had a lower glassy transition point compared to the components. The protein content and the encapsulation efficiency of the prepared pineapple snacks could be maximized using 16 g of WPC, 0 g of GA, 0.24 g of PP, and 8.78 g of LO. The concentrations of gum Arabic used in that study wasn’t effective in terms of encapsulation efficiency. However, it was determined that the contents of the other components at optimum conditions increased the encapsulation efficiency and the protein content of the pineapple snack.
Author contributions
formal analysis, investigation, E.S.; methodology, E.S.; resources, E.S.; software, M.M.; supervision, E.S.; validation, E.S.; visualization, E.S.; writing – original draft, writing – review and editing, E.S.; funding acquisition, E.S. All authors have read and agreed to the published version of the manuscript.
Acknowledgments
The authors thank to Savaşlar Soğutma Ltd. Sti. for the technical support.
Disclosure statement
No potential conflict of interest was reported by the author(s).
Data availability statement
Data is contained within the article.
Additional information
Funding
References
- Geneva: World Health Organization (WHO). Global nutrition policy review 2016–2017: country progress in creating enabling policy environments for promoting healthy diets and nutrition. 2020; pp 797.
- Featherstone, S. Jams, jellies, and related products, S. Featherstone (Editor), A Complete Course in Canning and Related Processes (Fourteenth Edition), Elsevier, 2016; pp 313–349.
- Zhang, Q.; Jin, K.; Chen, B.; Liu, R.; Cheng, S.; Zhang, Y.; Lu, J. Overnutrition Induced Cognitive Impairment: Insulin Resistance, Gut-Brain Axis, and Neuroinflammation. Front. Neurosci. 2022, 6, 884579. DOI: 10.3389/fnins.2022.884579.
- Dominguez, L. J.; Veronese, N.; Baiamonte, E.; Guarrera, M.; Parisi, A.; Ruffolo, C.; Tagliaferri, F.; Barbagallo, M. Healthy Aging and Dietary Patterns. Nutrients. 2022, 14(4), 889–911. DOI: 10.3390/nu14040889.
- Chen, K.; Zhang, M.; Bhandari, B.; Sun, J.; Chen, J. Novel Freeze Drying Based Technologies for Production and Development of Healthy Snacks and Meal Replacement Products with Special Nutrition and Function: A Review, Dry. Technol. 2022, 40(8), 1582–1597. DOI: 10.1080/07373937.2021.1967375.
- Donno, D.; Mellano, M. G.; Riondato, I.; De Biaggi, M.; Andriamaniraka, H.; Gamba, G.; Beccaro, G. L. Traditional and Unconventional Dried Fruit Snacks as a Source of Health-Promoting Compounds. Antioxidants. 2019, 8(9), 396–411. DOI: 10.3390/antiox8090396.
- Tylewicz, U.; Nowacka, M.; Rybak, K.; Drozdzal, K.; Dalla Rosa, M.; Mozzon, M. Design of Healthy Snack Based on Kiwifruit. Molecules. 2020, 25(14), 3309–3321. DOI: 10.3390/molecules25143309.
- Ciurzyńska, A.; Cieśluk, P.; Barwińska, M.; Marczak, W.; Ordyniak, A.; Lenart, A.; Janowicz, M. Eating Habits and Sustainable Food Production in the Development of Innovative “Healthy” Snacks. Sustainability. 2019, 11(10), 2800. DOI: 10.3390/su11102800.
- Grasso, S. Extruded Snacks from Industrial By-Products: A Review. Trends Food Sci. Technol. 2020, 99, 284–294. DOI: 10.1016/j.tifs.2020.03.012.
- Karwacka, M.; Ciurzyńska, A.; Galus, S.; Janowicz, M. Freeze-Dried Snacks Obtained from Frozen Vegetable By-Products and Apple Pomace – Selected Properties, Energy Consumption and Carbon Footprint. Innov. Food Sci. Emerg. Technol. 2022, 77, 102949. DOI: 10.1016/j.ifset.2022.102949.
- Izli, N.; Izli, G.; Taskin, O. Impact of Different Drying Methods on the Drying Kinetics, Color, Total Phenolic Content and Antioxidant Capacity of Pineapple. CYTA J. Food. 2018, 16(1), 213–221. DOI: 10.1080/19476337.2017.1381174.
- Lu, X. H.; Sun, D. Q.; Wu, Q. S.; Liu, S. H.; Sun, G. M. Physico-Chemical Properties, Antioxidant Activity and Mineral Contents of Pineapple Genotypes Grown in China. Molecules. 2014, 19(6), 8518–8532. DOI: 10.3390/molecules19068518.
- Rodríguez, Ó.; Gomes, W.; Rodrigues, S.; Fernandes, F. A. N. Effect of Acoustically Assisted Treatments on Vitamins, Antioxidant Activity, Organic Acids and Drying Kinetics of Pineapple. Ultrason. Sonochem. 2017, 35(Pt A), 92–102. DOI: 10.1016/j.ultsonch.2016.09.006.
- Mhatre, M.; Tilak-Jain, J.; De, S.; Devasagayam, T. P. A. Evaluation of the Antioxidant Activity of Non-Transformed and Transformed Pineapple: A Comparative Study. Food. Chem. Toxicol. 2009, 47(11), 2696–2702. DOI: 10.1016/j.fct.2009.06.031.
- Yuris, A.; Siow, L. F. A Comparative Study of the Antioxidant Properties of Three Pineapple (Ananas Comosus L.) Varieties. J. Food Stud. 2014, 3(1), 40–56. DOI: 10.5296/jfs.v3i1.4995.
- Ucak, I.; Khalily, R.; Carrillo, C.; Tomasevic, I.; Barba, F. J. Potential of Propolis Extract as a Natural Antioxidant and Antimicrobial in Gelatin Films Applied to Rainbow Trout (Oncorhynchus Mykiss) Fillets. Foods. 2020, 9, 1584. DOI: 10.3390/foods9111584.
- Forma, E.; Bryś, M. Anticancer Activity of Propolis and Its Compounds. Nutrients. 2021, 13(8), 2594–2615. DOI: 10.3390/nu13082594.
- Boufadi, M. Y.; Soubhye, J.; Van Antwerpen, P. Anti-Inflammatory, Antioxidant Effects, and Bioaccessibility of Tigzirt Propolis. J. Food Biochem. 2021, 45(4), e13663. DOI: 10.1111/jfbc.13663.
- An, J. Y.; Kim, C.; Park, N. R.; Jung, H. S.; Koo, T. S.; Yuk, S. H.; Lee, E. H.; Cho, S. H. Clinical Anti-Aging Efficacy of Propolis Polymeric Nanoparticles Prepared by a Temperature-Induced Phase Transition Method. J. Cosmet. Dermatol. 2022, 21(9), 4060–4071. DOI: 10.1111/jocd.14740.
- Pant, K.; Thakur, M.; Chopra, H. K.; Nanda, V. Encapsulated Bee Propolis Powder: Drying Process Optimization and Physicochemical Characterization. LWT - Food Sci. Technol. 2022, 155, 112956. DOI: 10.1016/j.lwt.2021.112956.
- Sagar, V. R.; Suresh Kumar, P. Recent Advances in Drying and Dehydration of Fruits and Vegetables: A Review. J. Food Sci. Technol. 2010, 47(1), 15–26. DOI: 10.1007/s13197-010-0010-8.
- Bourdoux, S.; Li, D.; Rajkovic, A.; Devlieghere, F.; Uyttendaele, M. Performance of Drying Technologies to Ensure Microbial Safety of Dried Fruits and Vegetables. Compr. Rev. Food Sci. Food Saf. 2016, 15(6), 1056–1066. DOI: 10.1111/1541-4337.12224.
- Belice, T.; Yüksel, A.; Akçiçek, S. F. Importance of Dried Fruits and Vegetables in the Older Adults. Eur. J. Geriatr. Gerontol. 2022, 2(2), 28–35. DOI: 10.4274/ejgg.galenos.2020.280.
- Zhu, J.; Liu, Y.; Zhu, C.; Wei, M. Effects of Different Drying Methods on the Physical Properties and Sensory Characteristics of Apple Chip Snacks. LWT - Food Sci. Technol. 2022, 154, 112829. DOI: 10.1016/j.lwt.2021.112829.
- Šturm, L.; Črnivec, I. G. O.; Istenič, K.; Ota, A.; Megušar, P.; Slukan, A.; Humar, M.; Levic, S.; Nedović, V.; Kopinč, R., et al. Encapsulation of Non-Dewaxed Propolis by Freeze-Drying and Spray-Drying Using Gum Arabic, Maltodextrin and Inulin as Coating Materials. Food Bioprod. Process. 2019, 116, 196–211. DOI: 10.1016/j.fbp.2019.05.008.
- Joshi, A.; Rupasinghe, H.; Khanizadeh, S. Impact of Drying Processes on Bioactive Phenolics, Vitamin C and Antioxidant Capacity of Red-Fleshed Apple Slices. J. Food Process Preserv. 2011, 35(4), 453–457. DOI: 10.1111/j.1745-4549.2010.00487.x.
- Hafezi, N.; Sheikhdavoodi, M. J.; Sajadiye, S. M. The Effect of Drying Kinetic on Shrinkage and Colour of Potato Slices in the Vacuum- Infrared Drying Method. Int. J. Agr. Food Res. 2015, 4(1), 24–31. DOI: 10.24102/ijafr.v4i1.476.
- Nowak, D.; Jakubczyk, E. The Freeze-Drying of Foods-The Characteristic of the Process Course and the Effect of Its Parameters on the Physical Properties of Food Materials. Foods. 2020, 9, 1488.
- Kahraman, O.; Malvandi, A.; Vargas, L.; Feng, H. Drying Characteristics and Quality Attributes of Apple Slices Dried by a Non-Thermal Ultrasonic Contact Drying Method. Ultrason. Sonochem. 2021, 73, 105510. DOI: 10.1016/j.ultsonch.2021.105510.
- Assegehegn, G.; Brito-de la Fuente, E.; Franco, J. M.; Gallegos, C. The Importance of Understanding the Freezing Step and Its Impact on Freeze-Drying Process Performance. J. Pharm. Sci. 2019, 108(4), 1378–1395. DOI: 10.1016/j.xphs.2018.11.039.
- Archaina, D.; Sosa, N.; Rivero, R.; Schebor, C. Freeze-Dried Candies from Blackcurrant (Ribes Nigrum L.) and Yoghurt. Physicochemical and Sensorial Characterization. LWT - Food Sci. Technol. 2019, 100, 444–449. DOI: 10.1016/j.lwt.2018.10.049.
- Çavdaroğlu, E.; Yemenicioğlu, A. Utilization of Stalk Waste Separated During Processing of Sun-Dried Figs (Ficus Carica) as a Source of Pectin: Extraction and Determination of Molecular and Functional Properties. LWT. 2022, 154, 112624. DOI: 10.1016/j.lwt.2021.112624.
- Garcia Loredo, A. B.; Guerrero, S. N. Correlation Between Instrumental and Sensory Ratings by Evaluation of Some Texture Reference Scales. Int. J. Food Sci. 2011, 46(9), 1977–1985. DOI: 10.1111/j.1365-2621.2011.02709.x.
- Khalloufi, S.; Ratti, C. Quality Deterioration of Freeze-Dried Foods as Explained by Their Glass Transition Temperature and Internal Structure. J. Food Sci. 2003, 68(3), 892–903. DOI: 10.1111/j.1365-2621.2003.tb08262.x.
- Fang, Z.; Bhandari, B. Spray Drying, Freeze Drying and Related Processes for Food Ingredient and Nutraceutical Encapsulation. In Encapsulation Technologies and Delivery Systems for Food Ingredients and Nutraceuticals; Nissim, G. McClements, D. J., Eds.; WP Woodhead Publishing: Oxford, 2012; pp. 73–109. DOI: 10.1533/9780857095909.2.73.
- Salvador, A.; Camacho, M. D. M.; Martínez-Navarrete, N. Influence of Formulation on the Quality and Stability of a Freeze-Dried Mandarin Product. Curr. Res. Food Sci. 2022, 20, 1047–1053. DOI: 10.1016/j.crfs.2022.06.004.
- Rezvankhah, A.; Emam‐Djomeh, Z.; Safari, M.; Salami, M.; Askari, G. Investigating the Effects of Maltodextrin, Gum Arabic, and Whey Protein Concentrate on the Microencapsulation Efficiency and Oxidation Stability of Hemp Seed Oil. J. Food Process Preserv. 2022, 46(6), e16554. DOI: 10.1111/jfpp.16554.
- Cemeroğlu, B. S. Gıda Analizleri. Bizim Grup Basıdmevi, Ankara, Türkiye, 2013, p. 480. ISBN:978-605-63419-3-9.
- Oikonomopoulou, V. P.; Krokida, M. K. Structural Properties of Dried Potatoes, Mushrooms, and Strawberries as a Function of Freeze-Drying Pressure. Dry. Technol. 2012, 30(4), 351–361. DOI: 10.1080/07373937.2011.639475.
- Nielsen, S.S. Food Analysis. 4th Edition, Food Science Text Series, Springer, USA, 2010, p. 602. http://dx.doi.org/10.1007/978-1-4419-1478-1.
- Sponton, O. E.; Perez, A. A.; Osella, C.; Cuffia, F.; Fenoglio, C.; Piagentini, A.; Santiago, L. G. Squalene Encapsulation by Emulsification and Freeze-Drying Process: Effects on Bread Fortification. J. Food Sci. 2023, 88(6), 2523–2535. DOI: 10.1111/1750-3841.16576.
- Sakooei-Vayghan, R.; Peighambardoust, S. H.; Javad Hesari, J.; Soltanzadeh, M.; Peressini, D. Properties of Dried Apricots Pretreated by Ultrasound-Assisted Osmotic Dehydration and Application of Active Coatings. Food Technol. Biotechnol. 2020, 58(3), 249–259. DOI: 10.17113/ftb.58.03.20.6471.
- Savas, E. The Modelling of Convective Drying Variables’ Effects on the Functional Properties of Sliced Sweet Potatoes. Foods. 2022, 11(5), 741–761. DOI: 10.3390/foods11050741.
- Bardakçı, B.; Seçilmiş, H. Investigation of Chemical Composition of Rose Oil from Isparta Region by GC-MS and FTIR Spectroscopy Technique. SDUFASJS. 2006, 1, 64–69.
- Altuğ, T; Elmacıd, Y. Gıddalarda Duyusal Değerlendirme. 2. Edtiiton, Sidas Medya, İzmir, Türkiye, 2011, p. 134.
- Golpira, F.; Maftoonazad, N.; Ramaswamy, H. S. Evaluation of Freeze Drying and Electrospinning Techniques for Saffron Encapsulation and Storage Stability of Encapsulated Bioactives. J. Compos. Sci. 2021, 5(12), 326. DOI: 10.3390/jcs5120326.
- Murali, S.; Kar, A.; Mohapatra, D.; Kalia, P. Encapsulation of Black Carrot Juice Using Spray and Freeze Drying. Food Sci. Technol. Int. 2015, 21(8), 604–612. DOI: 10.1177/1082013214557843.
- Zuidam, N. J.; Shimoni, E. Overview of Microencapsulates for Use in Food Products or Processes and Methods to Make Them. In Encapsulation Technologies for Active Food Ingredients and Food Processing; Springer New York: Berlin/Heidelberg, Germany, 2010 pp. 3–29. DOI: 10.1007/978-1-4419-1008-0_2.
- Mahdavi, S. A.; Jafari, S. M.; Assadpoor, E.; Dehnad, D. Microencapsulation Optimization of Natural Anthocyanins with Maltodextrin, Gum Arabic and Gelatin. Int. J. Biol. Macromol. 2016, 85, 379–385. DOI: 10.1016/j.ijbiomac.2016.01.011.
- Popa, V. M.; Gruia, A.; Raba, D.; Dumbrava, D.; Moldovan, C.; Bordean, D.; Mateescu, C. Fatty Acids Composition and Oil Characteristics of Linseed (Linum Usitatissimum L.) from Romania. J. Agroaliment. Processes Technol. 2012, 18, 136–140.
- Bayrak, A.; Kiralan, M.; Ipek, A.; Arslan, N.; Cosge, B.; Khawar, K. M. Fatty Acid Compositions of Linseed (LinumUsitatissimum L.) Genotypes of Different Origin Cultivated in Turkey. Biotechnol. Biotechnol. Equip. 2010, 24(2), 1836–1842. DOI: 10.2478/V10133-010-0034-2.
- Goyal, A.; Sharma, V.; Upadhyay, N.; Gill, S.; Sihaget, M. Flax and Flaxseed Oil: An Ancient Medicine & Modern Functional Food. J. Food Sci. Technol. 2014, 51(9), 1633–1653. DOI: 10.1007/s13197-013-1247-9.
- Hong, K.; Chen, L.; Gu, H.; Zhang, X.; Chen, J.; Nile, S. H.; Hu, M.; Gong, D.; Song, K.; Hou, X., et al. Novel Insight into the Relationship Between Metabolic Profile and Fatty Acid Accumulation Altering Cellular Lipid Content in Pineapple Fruits at Different Stages of Maturity. J. Agric. Food. Chem. 2021, 69(30), 8578–8589. DOI: 10.1021/acs.jafc.1c02658.
- Ciurzyńska, A.; Popkowicz, P.; Galus, S.; Janowicz, M. Innovative Freeze-Dried Snacks with Sodium Alginate and Fruit Pomace (Only Apple or Only Chokeberry) Obtained within the Framework of Sustainable Production. Molecules. 2022, 27(10), 3095. DOI: 10.3390/molecules27103095.
- Jakubczyk, E.; Kamińska-Dwórznicka, A.; Ostrowska-Ligęza, E. The Effect of Composition, Pre-Treatment on the Mechanical and Acoustic Properties of Apple Gels and Freeze-Dried Materials. Gels. 2022, 8(2), 110–128. DOI: 10.3390/gels8020110.
- Ratti, C. Hot Air and Freeze-Drying of High-Value Foods: A Review. J. Food Eng. 2021, 49, 311–319. DOI: 10.1016/S0260-8774(00)00228-4.
- Ciurzyńska, A.; Galus, S.; Karwacka, M.; Janowicz, M. The Sorption Properties, Structure and Shrinkage of Freeze-Dried Multi-Vegetable Snack Bars in the Aspect of the Environmental Water Activity. LWT - Food Sci. Technol. 2022, 171, 114090. DOI: 10.1016/j.lwt.2022.114090.
- Egas-Astudillo, L. A.; Martínez-Navarrete, N.; Camacho, M. M. Impact of Biopolymers Added to a Grapefruit Puree and Freeze-Drying Shelf Temperature on Process Time Reduction and Product Quality. Food Bioprod. Process. 2020, 120, 143–150.
- Ciurzyńska, A.; Marczak, W.; Lenart, A.; Janowicz, M. Production of Innovative Freeze-Dried Vegetable Snack with Hydrocolloids in Terms of Technological Process and Carbon Footprint Calculation. Food Hydrocoll. 2020, 108, 105993. DOI: 10.1016/j.foodhyd.2020.105993.
- Oyinloye, T. M.; Yoon, W. B. Effect of Freeze-Drying on Quality and Grinding Process of Food Produce: A Review. Processes. 2020, 8(3), 354. DOI: 10.3390/pr8030354.
- Athmaselvi, K. A.; Kumar, C.; Balasubramanian, M.; Roy, I. Thermal, Structural, and Physical Properties of Freeze-Dried Tropical Fruit Powder. J. Food Process. 2014, 3, 1–10. DOI: 10.1155/2014/524705.
- Jiamjariyatam, R. Development of Ready-To-Eat Rice Starch-Based Puffed Products by Coupling Freeze-Drying and Microwave. Int. J. Food Sci. Technol. 2016, 51(2), 444–452. DOI: 10.1111/ijfs.12989.
- Palzer, S.; Dubois, C.; Gianfrancesco, A. Generation of Product Structures During Drying of Food Products. Dry. Technol. 2012, 30(1), 97–105. DOI: 10.1080/07373937.2011.622060.
- Telis, V. R. N.; Martínez-Navarrete, N. Biopolymer Engineering in Food Processing, 1st ed.; CRC Press: Florida, 2012; (Chapter 8). DOI: 10.1201/b12048.
- Silva-Espinoza, M. A.; Salvador, A.; Camacho, M. D. M.; Martínez-Navarrete, N. Impact of Freeze-Drying Conditions on the Sensory Perception of a Freeze-Dried Orange Snack. J. Sci. Food Agric. 2021, 101, 4585–4590. DOI: 10.1002/jsfa.11101.
- Townrow, S.; Roussenova, M.; Giardiello, M.; Alam, A.; Ubbink, J. Specific Volume-Hole Volume Correlations in Amorphous Carbohydrates: Effect of Temperature, Molecular Weight, and Water Content. J. Phys. Chem B. 2010, 114, 1568–1578. DOI: 10.1021/jp908462k.
- Malik, N.; Gouseti, O.; Bakalis, S. Effect of Freezing on Microstructure and Reconstitution of Freeze-Dried High Solid Hydrocolloid-Based Systems. Food Hydrocoll. 2018, 83, 473–484. DOI: 10.1016/j.foodhyd.2018.05.008.
- Marques, L. G.; Prado, M. M.; Freire, J. T. Rehydration Characteristics of Freeze-Dried Tropical Fruits. LWT - Food Sci. Technol. 2009, 42(7), 1232–1237. DOI: 10.1016/j.lwt.2009.02.012.
- Serna-Cock, L.; Vargas-Muñoz, D. P.; Aponte, A. A. Structural, Physical, Functional and Nutraceutical Changes of Freeze-Dried Fruit. Afr. J. Biotechnol. 2015, 14, 442–450. DOI: 10.5897/AJB2014.14189.
- Wan, J.; Ding, Y.; Zhou, G.; Luo, S.; Liu, C.; Liu, F. Sorption Isotherm and State Diagram for Indica Rice Starch with and without Soluble Dietary fiberJ. Cereal Sci. 2018, 80, 44–49. DOI: 10.1016/j.jcs.2018.01.003.
- Malik, N.; Muttakin, S.; Lopez-Quiroga, E.; Watson, N. J.; Fryer, P. J.; Bakalis, S.; Gouseti, O. Microstructure and Reconstitution of Freeze-Dried Gum Arabic at a Range of Concentrations and Primary Drying Temperatures. Food Hydrocoll. 2020, 104, 105712. DOI: 10.1016/j.foodhyd.2020.105712.
- Nemzer, B.; Vargas, L.; Xia, X.; Sintara, M.; Feng, H. Phytochemical and Physical Properties of Blueberries, Tart Cherries, Strawberries, and Cranberries as Affected by Different Drying Methods. Food Chem. 2018, 262, 242–250. DOI: 10.1016/j.foodchem.2018.04.047.
- Djendoubi Mrad, N.; Bonazzi, C.; Boudhrioua, N.; Kechaou, N.; Courtois, F. Influence of Sugar Composition on Water Sorption Isotherms and on Glass Transition in Apricots. J. Food Eng. 2018, 111, 403–411. DOI: 10.1016/j.jfoodeng.2012.02.001.
- Homer, S.; Kelly, M.; Day, L. Determination of the Thermo-Mechanical Properties in Starch and Starch/Gluten Systems at Low Moisture Content – a Comparison of DSC and TMA. Carbohydr. Polym. 2014, 108, 1–9. DOI: 10.1016/j.carbpol.2014.02.049.
- Goula, A. M.; Adamopoulos, K. G. Effect of Maltodextrin Addition During Spray Drying of Tomato Pulp in Dehumidified Air: I. Drying Kinetics and Product Recovery. Drying Technol. 2008, 26, 714–725. DOI: 10.1080/07373930802046369.
- Uscanga, M. A.; Salvador, A.; Camacho, M. M.; Martínez-Navarrete, N. Impact of Freeze-Drying Shelf Temperature on the Bioactive Compounds, Physical Properties and Sensory Evaluation of a Product Based on Orange Juice. Int. J. Food Sci. 2021, 56, 5409–5416. DOI: 10.1111/ijfs.15086.
- Valentina, V.; Pratiwi, A. R.; Hsiao, P. Y.; Tseng, H. T.; Hsieh, J. F.; Chen, C. C. Sensorial Characterization of Foods Before and After Freeze-Drying. Austin. Food Sci. 2016, 1, 1027.