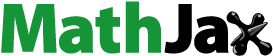
ABSTRACT
To effectively utilize the by-products of skipjack tuna (Katsuwonus Pelamis) for the preparation of peptides with lipid-lowering activity, alkaline proteinase was selected from six commercial proteases to hydrolyze dark muscle. An L16 (54) orthogonal design was employed to optimize the hydrolysis conditions, resulting in the production of a high-peptide-content hydrolyzate named KPHs-AL. This hydrolyzate exhibited the ability to significantly reduce TG content. KPHs-AL also showed higher persuasive pancreatic lipase (PL) and cholesterol esterase (CEase) inhibitory potential than various molecular weight (Mw) fractions and other enzymatic hydrolyzates, with IC50 values of 5.14 and 8.51 mg/mL, respectively. Furthermore, KPHs-AL exerted a combined effect of regulating lipid accumulation in FAA-induced steatosis HepG2 cells, leading to decreased total cholesterol (TC) and total triglyceride (TG). Based on the high binding likelihood and interaction with specific binding sites predicted by Pepsite2, 38 peptide sequences were identified as potential PL and CEase inhibitors. Hence, KPHs-AL holds promise as a beneficial component of functional food and exhibits the potential for anti-obesity effects through the alleviation of lipid accumulation
Introduction
Obesity is defined as a chronic, complex disease characterized by abnormal lipid metabolism and excessive fat accumulation, which may impair health. It affects various system in the body, including cardiovascular, aerodigestive, circulatory and genitourinary systems, and can lead to a range of non-communicable diseases. Recent statistics from the WHO Global Health Observatory show that globally, more than 1 billion children, adolescents and adults are obese. The prevalence rate has nearly tripled since 1975, resulting in a significant rise in healthcare costs associated with related disease. Consequently, obesity remains an ongoing global public health problem.[Citation1,Citation2] C-1: References should be number format? Pancreatic lipase (PL) catalyzes the lipid hydrolysis of triacylglycerols into glycerol monoesters and fatty acids, playing an essential role in the digestion and absorption of dietary fats in the digestive tract. Similarly, cholesterol esterase (CEase) primarily hydrolyzes dietary cholesterol esters, liberating cholesterol and free fatty acids tofacilitate the absorption process of the body.[Citation3] Inhibiting the activities of both enzymes can effectively control the hydrolysis of dietary fats and cholesterol esters, subsequently regulating the plasma levels of triglycerides and cholesterol. This approach provides an effective strategy for treating metabolic disorders and managing obesity-associated complications.[Citation4,Citation5] In this regard, various synthetic drugs based on the principle of PL and CEase inhibition have been developed and proven useful in managing obesity-associated complications.[Citation6] For instance, Orlistat has been clinically proven to be effective in significantly and sustainably treating obesity by inhibiting PL’s enzymatic action. However, these drugs come with severe gastrointestinal adverse effects, reduced levels of fat-soluble vitamins, and high long-term costs, imposing certain limitations.[Citation7] Given these concerns with synthetic drugs, there is a growing need to discover and understand safer, more economical natural alternatives.
In recent years, the exploration of bioactive peptides has opened up exciting new avenues for addressing lipid disorders.[Citation8] Peptides, short chains of amino acids, have demonstrated a wide range of biological activities, including the ability to modulate lipid metabolism. These bioactive peptides have garnered significant attention due to their potential in improving lipid profiles and, consequently, their implications for health and medicine. Sajid Maqsood’s group systematically investigated the potential anti-obesity activities of camel milk-derived bioactive peptides, including in vitro inhibition of PL[Citation9] and CEase[Citation10]of camel milk protein hydrolyzate, the anti-obesity potential and interactions mechanism[Citation11]of camel whey protein hydrolyzate,[Citation12] and the inhibitory potential of camel casein hydrolyzate against key lipid-digesting enzymes.[Citation13] Several proteins or by-products from the food industry are considered high-quality sources of peptides or hydrolyzates that interfere with fat digestion and absorption, and alleviate lipid accumulation, such as Cuminum cyminum seeds,[Citation14] olive seeds,[Citation15] Phoenix dactylifera L. seeds,[Citation16] amaranth seeds[Citation17] and brewer’s spent grain.[Citation18]
Skipjack Tuna (Katsuwonus Pelamis) is a species of significant commercial importance, primarily sought after for its use in canned food products [.Citation19] As consumer demand for this species steadily increases, a substantial portion of the fish, including not just the desirable white meat but also the head, tail, viscera, bones, and dark muscle, inevitably becomes available as by-products.[Citation20] To date, a wide range of bioactive peptides has been identified from those processing by-products of tun, specifically, antioxidant and angiotensin-I-converting enzyme inhibitory peptides from dark muscle[Citation21,Citation22] or blood[Citation23] or roe[Citation24] protein hydrolyzates, antioxidant peptides from the in vitro gastrointestinal digestion products of the head[Citation25]or milt protein hydrolyzate[Citation26] or bone collagen,[Citation27,Citation28] high fischer ratio oligo-peptides hydrolyzate from minced meat.[Citation28] Peptides with lipid-lowering properties can be incorporated into functional foods and nutraceuticals, providing individuals with dietary options to naturally manage their lipid levels. These products offer a convenient and appealing way to promote heart health through everyday consumption. Nonetheless, there are still gaps in the research on the potential of skipjack tuna dark muscle protein hydrolyzate to alleviate lipid accumulation, especially the PL and CEase inhibitory activities.
The objective of this work was to explore the potential of skipjack tuna dark muscle protein hydrolyzates, generated using commercial enzymes, to inhibit two key metabolic enzymes and reduce lipid accumulation in HepG2 cells. L16 (54) orthogonal design was used to optimize the hydrolysis process for the release of potential inhibitory peptides derived from dark muscle. The obtained hydrolyzate underwent characterization, including analysis of its molecular weight (Mw) distribution, amino acid (AA) composition, and identification of peptide sequences. In addition, the effects of these potent hydrolyzates were assessed through in vitro assays and in-silico methods to gain insights into the possible interactions of inhibitory peptides with pancreatic lipase (PL) and cholesterol esterase (CEase).
Materials and methods
Chemicals and reagents
The commercially available Katsuwonus pelamis were provided by Zhejiang Xingye Group co., Ltd. (Zhoushan, China) and transported to our biology laboratory to separate dark muscle. Alkaline proteinase (from Bacillus licheniformis, 200 U/mg), papain (from Carica papaya, 600 U/mg), acid protease (from Aspergillus niger, 50 U/mg), pancreatin (from bovine pancreas, 4 U/mg), neutral protease (from Bacillus subtilis, 200 U/mg), bromelain (from pineapple stem, 1200 U/mg), for the enzymolysis of dark muscle, were purchased from Nanning Donghenghuadao Biotechnology Co., Ltd. (Nanning, China). LC/MS grade 0.1% formic acid-acetonitrile (v/v) and 0.1% formic acid-water were purchased from Thermo Fisher Scientific Inc. (NY, USA). Other analytical grade chemicals and reagents for biochemical assays were also purchased from Sigma Aldrich (St. Louis, MO, USA). Dulbecco’s modified Eagle’s medium (DMEM) was obtained from Thermo Fisher Scientific Inc. (NY, USA), Fetal bovine serum (FBS) was purchased from Zhejiang Tianhang Biotechnology Co., Ltd. (Hangzhou, China). Sodium palmitate (10 M) and Sodium oleate (20 M) were purchased from Kunchuang Technology Development Co., Ltd. (Xian, China). The kits for total cholesterol (TC), triglyceride (TG), and oil red O staining were obtained from Jiancheng Bioengineering Institute (Nanjing, China).
Preparation & optimization of katsuwonus pelamis dark muscle hydrolysates (KPHs)
The pre-homogenized Katsuwonus pelamis dark muscle was hydrolyzed as the working parameters listed in . A constant-flow pump system was equipped with 0.5 M sodium hydroxide and 0.5 M hydrochloric acid was equilibrated to the desired pH (Lohand, DG150, Hangzhou, China). After that, the reaction system was heated to 100°C to inactivate these enzymes. Dark muscle from Katsuwonus pelamis was enzymatically hydrolyzed using different proteases to obtain distinct hydrolyzates. Specifically, hydrolysis with neutral protease resulted in KPHs-NP, alkaline protease yielded KPHs-AL, papain produced KPHs-PP, acid protease generated KPHs-ACP, bromelain led to KPHs-BM, and pancreatin produced KPHs-PC. These hydrolyzates were designated based on the respective proteases used for the hydrolysis process. The molecular weight fractions of KPHs-AL were categorized as follows: KPHs-AL-I for those with a molecular weight greater than 10 kDa, KPHs-AL-II for those ranging between 5 kDa and 10 kDa, KPHs-AL-III for those within the 5 kDa to 3 kDa range, and KPHs-AL-Ⅳ for those spanning between 3 kDa and 0.15 kDa. The supernatant was collected by centrifugation (Himac, CF16RN, Tokyo, Japan) and lyophilized for further use (Marin Christ, α1–4 LD plus, Germany). A five-factor-four level orthogonal design[Citation28] was applied to optimize the hydrolysis parameters to discriminate the lipid accumulation inhibitory effect and peptide content of different hydrolyzates. The peptides fractions were separated using a Cogent Scale TFF ultrafiltration system (Merck Millipore, Darmstadt, Germany) equipped with Mw cutoff membranes of 10, 5, 3 and 0.15 kDa, which are used for further bioactivity assay after dialyzation and lyophilization.
Table 1. Hydrolysis parameters for Katsuwonus pelamis dark muscle.
Characterization of KPHs
Peptide content
The peptide content of the hydrolyzates was determined by the trichloroacetic acid precipitation-biuret colorimetric method.[Citation29] Specifically, the hydrolyzates were pre-precipitated with 10% trichloroacetic acid, the centrifuged supernatant was mixed with biuret reagent, and the absorbance was measured at 540 nm to calculate the peptide concentrations (mg/mL) with the standard curve of Gly-Gly-Tyr-Arg tetrapeptide. In addition, the peptide content (mg/mg) was normalized according to the mass of the lyophilized powder.
Molecular weight distribution
The molecular weight (Mw) distribution of the hydrolyzates was determined by size exclusion chromatography as the same process described in our previous methods.[Citation30] Specifically, samples and the standard mix were eluted with acetonitrile/H2O/TFA (45:55:0.1) at a flow rate of 1.0 mL/min by using Agilent 1260 Infinity II HPLC System (Agilent Technologies, Inc., CA, USA) equipped with a TSK gel G2000 SWXL analytical column (7.8 × 300 mm, 5 µm, TOSOH, Tokyo, Japan). The function of standard Mw and retention time was used to calculate the Mw distribution of KPHs.
Amino acid composition
The amino acid (AA) composition of the hydrolyzates was determined by three processes[Citation31]: ion exchange chromatography separation, ninhydrin derivatization and absorbance monitoring at 570 nm/440 nm (HITACHI L-8900, Tokyo, Japan). Prior to the 60 min standard protein hydrolyzate analysis, the samples and high-purity hydrochloric acid (containing 2% phenol, m/v) were maintained at 110°C, 100% nitrogen atmosphere for 22 h to fully hydrolyze.
Identification of peptide sequences
Peptide sequences identification for samples was conducted using a Q Exactive HF LC/MS system (Thermo Scientific, CA, USA). The sample was injected into an 80-min gradient system equipped with a Nano Chrom XP C18 column (250 mm × 7.5 μm, 3 μm, Eksigent, CA, USA). The binary mobile phase consisting of 0.1% formic acid in acetonitrile (solvent A) and water (solvent B) was used at a constant flow rate of 600 nL/min. The elution procedure was performed as follows: 0 min, 94% solvent A, 5 min, 88% solvent A, 57 min, 71% solvent A, 71 min, 60% solvent A, 72 min, 5% solvent A, 80 min, 5% solvent A. Data analysis and library search for the MS/MS information collected in positive ion mode were performed with PEAKS studio version 6.0. Only peptides with a Denovo resolution confidence >80% or contained in the protein sequences from the species were identified in the following step.[Citation8]
In vitro analysis of lipid accumulation inhibition
Pancreatic lipase inhibition assay
Pancreatic lipase (PL) inhibitory assay was carried out according to the method previously described by with minor modifications.[Citation32] The preincubation system (37°C, 10 min) consisted of 50 μL of KPHs or fractions, 50 μL of PL (1 mg/mL) in 0.1 M Tris-HCl, pH 7.4. Then, 50 μL p-nitrophenyl butyrate substrate (5 mM) was added to initiate the incubation reaction for 20 min, and the Abs405 was measured to calculate the inhibition rate.
where Aa is the absorbance obtained without inhibitor (KPHs) and with PL, Ab is the absorbance obtained without (KPHs) and PL, Ac is the absorbance obtained with inhibitor and PL, and Ad is the absorbance obtained with inhibitor and without PL.
Cholesterol esterase inhibition assay
Cholesterol esterase (CEase) inhibition assay was performed following the previous method[Citation33]with slight modification. The KPHs or fractions (50 μL) at different concentrations were mixed with 100 μL sodium phosphate buffer (100 mM, pH 7.0) containing taurocholic acid (5.16 mM) and p-nitrophenyl butyrate substrate (5 mM). Then, 50 μL CEase (5 μg/mL) initiated the reaction system and the Abs405 after 30 min was measured to calculate the inhibition rate.
Where Aa is the absorbance obtained without inhibitor (KPHs) and with CEase, Ab is the absorbance obtained without (KPHs) and CEase, Ac is the absorbance obtained with inhibitor and CEase, and Ad is the absorbance obtained with inhibitor and without CEase.
Cellular analysis of lipid accumulation inhibition
Cell culture and treatment
HepG2 cell line (CSTR:19375.09.3101HUMSCSP510) from the Cell Bank of Chinese Academy of Science (Shanghai, China) was routinely cultured in DMEM high-glucose medium supplemented with 10% heat-inactivated fetal calf serum (FBS) and maintained at 37°C, 5% CO2 and 95% humidified atmosphere (Forma 3111 CO2 incubator, Thermo Forma, USA). HepG2 cells were seeded into 96-well plates (1 × 104/well) overnight and treated with free fatty acids (FFAs, 400 μM sodium oleate and 200 μM sodium palmitate) and/or different concentrations of KPHs for 24 h.
Cell viability assay
HepG2 cells were seeded into 96-well plates (1 × 104/well) overnight and treated with FFAs and/or KPHs for 24 h. After treatment, an additional 10 μL CCK-8 reagent was added to each well and incubated at 37°C for 1 h. The cell viability was measured by Cell Counting Kit-8 (CCK-8) (Beyotime Biotechnology, Shanghai, China). The absorbance at 450 nm was measured to calculate cell viability using the following equation[Citation34]:
Oil red O staining
HepG2 cells were seeded into 6-well plates (2 × 105/well) overnight and treated with FFAs and/or KPHs for 24 h. Cells were fixed with 4% paraformaldehyde for 10 min at room temperature. Following fixation, the slides underwent a series of carefully executed steps. They were rinsed with distilled water twice and subsequently immersed in 60% isopropanol for a duration of 5 minutes. Subsequently, freshly prepared Oil Red O reagent was applied to the slides and allowed to incubate for precisely 15 minutes. After the incubation period, the slides underwent thorough washing with distilled water until complete removal of Oil Red O dye was achieved. For further staining, hematoxylin stain was applied to the slides for exactly 1 minute, followed by a subsequent rinse with distilled water, adhering to the manufacturer’s guidelines (Solarbio, Beijing, China). The slices were acquired images with a Biomicroscope C×31inverted microscope (OLYMPUS, Tokyo, Japan). Orlistat was used as the positive control.
Biochemical parameters assay
HepG2 cells were seeded into 6-well plates (2 × 105/well) overnight and treated with FFAs and/or KPHs (5 mg/mL and 10 mg/mL) for 24 h. Orlistat (10 µg/mL) was used as the positive control. Intracellular levels of TG and TC were measured by commercial kits according to the manufacturer’s instructions, and normalized by quantification of total protein concentration.
In silico analysis of KPHs
The PeptideRanker Server (http://distilldeep.ucd.ie/PeptideRanker/, accessed February 22, 2022) based on the N-to-1 neural network returns the predicted bioactivity probability ranking report of KPHs, and the peptides predicted over the threshold of 0.8 were labeled. Subsequently, the ToxinPred server (https://webs.iiitd.edu.in/raghava/toxinpred/design.php, accessed February 23, 2022) was used to predict the toxicity of given peptides to help identify the particular peptide without development restrictions. Pepsite2(http://pepsite2.russelllab.org/help, accessed February 25, 2022) was used to validate the interactions between PL (PDB code: 1ETH) or CEase (PDB code: 1AQL) and the above output peptide sequences to identify the promising peptide fragments. Predicted matches with statistical significance (P < .05) were highlighted as the potential binding sites and the candidate peptides that interacted with amino acids at the catalytic and substrate binding sites. Within the limits of the Pepsite2 server for input peptide sequences, the sequences containing more than ten amino acid residues were bisected to obtain p-values.
Statistical analysis
Results were presented as mean ± standard deviation. Data were analyzed by one-way variance method (ANOVA), followed by multiple tests using the Prism 9.0 software (GraphPad, La Jolla, CA) to assure the significant difference at P < .05.
Results and discussions
Preparation of lipid-lowering hydrolysates
Katsuwonus pelamis dark muscle hydrolyzates (KPHs) were prepared using six commercially available proteases. The enzyme inhibition assay and cellular TG accumulation assay were performed to screen the potent lipid-lowering peptides fraction ( & ). The IC50 values of the protein hydrolyzates produced by alkaline proteinase (KPHs-AL), neutral protease (KPHs-NP) and papain (KPHs-PP) on PL were 5.14, 7.55 and 14.56 mg/mL, respectively, which were significantly lower than other hydrolyzates. In addition, KPHs-AL showed the lowest IC50 value (8.51 mg/mL) for inhibiting CEase activity. KPHs-AL exhibited the most competitive TG content reduction performance in the hepatic steatosis cell model (The results of FFA-induced steatosis in HepG2 cells were shown in Figure S1), which was 38.13 ± 0.03%, suggesting that Alkaline protease may be a high-quality manufacturer of lipid-lowering peptides from Katsuwonus pelamis dark muscle. Alkaline proteinase has been reported to have differential effects on hydrolyzate activity due to its different cleavage patterns.[Citation33] Several reports demonstrated that alkaline proteinase hydrolyzates obtained from porcine liver or scallops had better functional activity than other commercial enzymatic hydrolyzates[Citation35] and.[Citation36]
Figure 1. Effects of Katsuwonus pelamis dark muscle hydrolyzates (KPHs) prepared using different commercial proteases on TG contents in FFA-induced HepG2 cells.

Table 2. Inhibitory effects of Katsuwonus pelamis dark muscle hydrolyzates (KPHs) prepared using different commercial proteases on PL and CEase.
With regards to this, the hydrolysis parameters of KPHs-AL were comprehensively designed and analyzed based on the five-factor-four level orthogonal experiment to obtain a more reliable combination (). The results of range (R) analysis revealed that the levels change of temperature (A) and hydrolysis time (D) had a great influence on the lipid-lowering performance, which were the optimal factors, but the effects of liquid to material (C), pH (B) and enzyme concentration (E) were all insignificant. In terms of peptides content, the influential order of the four factors within the extreme value R was A > D > C > B > E, which also indicated the significant effect of factors A and D. In combination with the result of decrease rate of TG content and peptides content, the superior parameters combination was determined to A1B1C4D2E3, which were 45°C, pH 8, liquid to material 5:1 mL/g, hydrolysis time 4 h and enzyme concentration 5000 U/g.
Table 3. Design of L16 (54) orthogonal experiment for the preparation of KPHs-AL.
Separation and characterization of lipid-lowering hydrolysates
The in vitro inhibitory effects of KPHs-AL fractions on PL or CEase were elucidated with the concentration–response curve . The different Mw fractions of KPHs-AL presented a concentration-response percentage inhibition activity, and KPHs-AL-Ⅳ (3 kDa–0.15 kDa) exhibited a general inhibition percentage, which was 88.07% to PL and 78.33% to CEase at the concentration of 25 mg/mL, which was comparable to KPHs-AL-III (5 kDa–3 kDa). The percentage inhibition activity of KPHs-AL-I (>10 kDa) and KPHs-AL-II (10 kDa–5 kDa) is lower than the KPHs-AL and other fractions. However, the KPHs-AL fraction showed the best half inhibitory concentration value of 5.14 mg/mL and 8.51 mg/mL compared with different Mw fractions. In addition, a significant decrease (P < .05 vs Model) in TG content was also observed in FFA-induced HepG2 cells with KPHs-AL intervention, which was only about 45.06% of the lipid accumulation in the FFA model group . Heretofore, KPHs-AL fractions failed to present a significant cytotoxic effect below the test range of 5–30 mg/mL
Figure 2. Effects of KPHs-AL fractions on PL (A) or CE (B) inhibition, cellular TG contents (C) and cell viability (D) in HepG2 cells. # P < .05 vs. Control group.

The Mw distribution of KPHs-AL was determined by the peak area normalization method using the calibration equation fitted to the standard Mw mix (). For KPHs-AL, the percentage of Mw in the range of 3 kDa–0.15 kDa was the highest (91.32%) among other Mw fractions, which were 1.6% (>10 kDa). Previous research indicated that low Mw peptides generated by enzymatic hydrolysis had shown great potential for bioactivity.[Citation37] In general, the absorption of high molecular weight (HMW, >1,000) peptides within the human body is relatively limited. The human body possesses the capacity to absorb peptides of various molecular sizes. However, smaller peptides are generally more readily absorbed. Nevertheless, it is crucial to note that the absorption characteristics of peptides are influenced by multiple factors beyond just their molecular weight. Factors such as peptide structure, gastrointestinal conditions, and the presence of synergistic compounds can also impact their absorption within the body. Therefore, further research is warranted to gain a deeper understanding of the absorption and metabolism of KPHs-AL within the human body. These studies will contribute to a comprehensive assessment of the feasibility of KPHs-AL as a potential therapeutic approach or dietary supplement
The amino acid composition of KPHs-AL is summarized in , and 16 amino acids including glutamic acid (11.26 ± 0.05 g/100 g pro.), lysine (5.59 ± 0.01 g/100 g pro.) and aspartic acid (5.24 ± 0.04 g/100 g pro.) were detected. Furthermore, the branched-chain amino acids (BCAAs, Ile, Leu and Val) accounted for 17.24% of total amino acids, and glutamic and aspartic acids accounted for 18.39% and 8.49% in KPHs-AL, respectively. Previous reports indicated that the BCAAs,[Citation34] Asp and Glu[Citation15] in the peptide sequences were considered to have regulatory functions in lipid metabolism. The study conducted by D. Ruan, et al showed that Dietary Lys supplementation influenced the activities of coenzymes in connection with lipid metabolism and promoted fatty acid β-oxidation.[Citation34] It had been reported that leucine (Leu) decreased fat deposition .
Table 4. Amino acid composition of KPHs-AL.
Inhibitory effects of KPHs-AL on lipid accumulation
Combined with the Oil Red O staining results, the co-treatment of KPHs-AL significantly decreased the lipid droplet deposition in FFA-induced HepG2 cells . Simultaneously, the effects of KPHs-AL on TC and TG in steatosis HepG2 cells were determined, as shown in . Orlistat co-treated (positive control) could extremely decreased intracellular TC (P < .01) and TG (P < .01) levels. Compared with the model group, similar effects to positive control were observed in KPHs-AL (5 mg/mL and 10 mg/mL), which significantly attenuates FFA-induced lipid accumulation in HepG2 cells.
Identification and molecular interaction of KPHs-AL
Forasmuch as the potent inhibitory activities in vitro and cellular lipid-lowering effect, the fraction was used for database search and de novo sequencing of peptide sequences. In the sequencing phase, the peptide sequences with an average local confidence (ALC) value of ≥ 80% were screened for subsequent procedures (data not shown). Overall, a total of 1938 nontoxic and potentially bioactive peptides were identified from the KPHs-AL fraction (Table S1), as PeptideRanker predicted probability >0.8 and ToxinPred SVM score <0. Moreover, the protein–peptide interaction in-silico prediction of potential bioactive peptides was performed by the Pepsite 2 server. Table S2- 5 shows the P-values and interacting acceptor residues of the best match between the identified peptides in KPHs-AL fraction and PL or CEase. The peptide fragments with foul residue numbers were equally divided and submitted to the server, with statistical significance in both portions retained.
Among the identified peptides, 1845 and 662 peptide fragments were confirmed to bind with PL or CEase (P < .05). In particular, both portions of 58 and 11 peptide fragments (up to 16 residues) tend to interact with enzymes. Apart from the high binding likelihood, interactions with specific binding sites of PL (PDB code: 1ETH) were taken into consideration, as these activity residues were regarded as potential indicators of inhibitory properties. The interaction of the active site maintained almost all peptide fragments that significantly binding to PL, and 1766 peptide fragments could bind to 3 catalytic sites (SER153、PHE216 and HIS264) simultaneously. Hence, these peptides were proposed to be potential PL inhibitors. Previously study showed that many bioactive peptides involved in the binding of CEase active sites, including catalytic triads (SER194, ASP320, andHIS435) and oxygen anion holes (GLY107, ALA108, and ALA195), may inhibit the process of cholesterol ester catalytic hydrolysis to release free cholesterol and reduce dietary cholesterol digestion/absorption. Next, the peptide fragments with high interaction likelihood (P < .05) were evaluated with putative CEase binding profiles. However, only 38 peptide sequences were found to be characterized by interaction residues favorable for the development of reductase inhibitors and showed reasonable interactions with the above active sites.
Consequently, the peptide fragments with favorable binding profiles with PL and CEase were cross-checked, emphasized, and shown in . Selected peptide sequences were confirmed to have a high statistical significance of binding (P < .05), further supported by the abundant interactions with specific binding sites. In terms of interaction with PL, the association of the catalytic triad with peptide residues predominated, while other peptide sequences, including AFF, CTF, FAL, FFF, FTF, LAF and VFF, also cooperated with SER153 and PHE216. As shown in , it was found that peptide residues frequently bound on CEase include catalytic residues SER194 and HIS435, and oxygen anion hole residues GLY107 and ALA108, but no interaction of peptide sequences with ASP320 was noted. Selected peptide sequences included a number of Phe (F), Leu (L) and Pro (P), as seen in . Hydrophobic residues were considered involved in restricting the functionality of cholesterol esterase and lipase. The presence of hydrophobic residues at the N-terminus may have followed this assumption.
Table 5. Potential binding sites between multienzyme inhibitory peptides and PL using PepSite 2 server and their P-values.
Table 6. Potential binding sites between multienzyme inhibitory peptides and CEase using PepSite 2 server and their P-values.
Conclusion
In the present study, the qlkaline proteinase protein hydrolyzate from skipjack tuna dark muscle may affect intracellular lipid accumulation by interacting with PL and CEase. Moreover, 38 peptide fragments were identified and predicted as potential inhibitors of PL and CEase that can bind to multiple active sites on key metabolic enzymes. These potential peptides could be candidates for further activity validation and mechanistic studies during more challenging in vitro and animal experiments. This research not only enhances our understanding of the economic potential of utilizing dark muscle from fishery processing by-products but also contributes valuable insights into novel peptides with the capacity to modulate lipid metabolism-related enzymes. These peptide sequences, with their inhibitory potential, will undergo comprehensive investigation in subsequent studies. The subsequent research stemming from this study is expected to build upon these significant findings and explore various aspects that can enhance our understanding and potentially unlock practical applications of the alkaline proteinase protein hydrolyzate from skipjack tuna dark muscle.
CRediT author statement
Fangfang Huang: Investigation, Validation, Methodology, Writing – review & editing. Qingfei Dai: Formal analysis. Kewei Zheng: Formal analysis. Qingbao Ma: Investigation, Methogology. Yu Liu: Formal analysis. Wei Jiang and Xiaojun Yan: Writing – original draft, Writing – review & editing, Project administration, Funding acquisition.
Ethics statement
This research did not include any human subjects or animal experiments.
Supplemental Material
Download ()Acknowledgments
Funding for this research was provided by Public Projects of Zhejiang Province (LGN19C200017), National Key R&D Program of China (2020YFD0900900) and Zhoushan Science and Technology Project (2020C21004).
Disclosure statement
No potential conflict of interest was reported by the author(s).
Supplementary data
Supplemental data for this article can be accessed online at https://doi.org/10.1080/10942912.2023.2267788
Additional information
Funding
References
- The Lancet, G.; Hepatology. Obesity: Another Ongoing Pandemic. Lancet. Gastroenter. Hepa. 2021, 6(6), 411. DOI: 10.1016/S2468-1253(21)00143-6.
- WHO. World Obesity Day 2022 – Accelerating Action To Stop Obesity. https://www.who.int/news/item/04-03-2022-world-obesity-day-2022-accelerating-action-to-stop-obesity. 2022 (Accessed Mar 4, 2022).
- Zhao, S.; Wu, Y.; Hu, L. Identification and Synthesis of Selective Cholesterol Esterase Inhibitor Using Dynamic Combinatorial Chemistry. Bioorg. Chem. 2022, 119, 105520. DOI: 10.1016/j.bioorg.2021.105520.
- Singh, H.; Singh, J. V.; Kaur, N.; Sanduja, M.; Singh, G.; Bedi, P. M. S.; Sharma, S. Rational Approaches, Design Strategies, Structure Activity Relationship and Mechanistic Insights for Esterase Inhibitors. Mini. Rev. Med. Chem. 2018, 18(10), 837–894. DOI: 10.2174/1389557517666170807124507.
- T, B. B.; R, M.; TNK, S.; N, S. A Review on Pancreatic Lipase Inhibitors from Natural Sources: A Potential Target for Obesity. Curr. Enzyme. Inhib. 2021, 17(2), 83–97. DOI: 10.2174/1573408017666210121114441.
- Bessesen, D. H.; Van Gaal, L. F. Progress and Challenges in Anti-Obesity Pharmacotherapy. Lancet. Diabete. Endocri. 2018, 6(3), 237–248. DOI: 10.1016/S2213-8587(17)30236-X.
- Goldman, V. E.; Naguib, M. N.; Vidmar, A. P. Anti-Obesity Medication Use in Children and Adolescents with Prader–Willi Syndrome: Case Review and Literature Search. J. Clin. Med. 2021, 10(19), 4540. DOI: 10.3390/jcm10194540.
- Huang, Y.-P.; Dias, F. F. G.; Leite Nobrega de Moura Bell, J. M.; Barile, D. A Complete Workflow for Discovering Small Bioactive Peptides in Foods by LC-MS/MS: A Case Study on Almonds. Food Chem. 2022, 369, 130834. DOI: 10.1016/j.foodchem.2021.130834.
- Mudgil, P.; Kamal, H.; Yuen, G. C.; Maqsood, S. Characterization and Identification of Novel Antidiabetic and Anti-Obesity Peptides from Camel Milk Protein Hydrolysates. Food Chem. 2018, 259, 46–54. DOI: 10.1016/j.foodchem.2018.03.082.
- Mudgil, P.; Baby, B.; Ngoh, Y.-Y.; Vijayan, R.; Gan, C.-Y.; Maqsood, S. Identification and Molecular Docking Study of Novel Cholesterol Esterase Inhibitory Peptides from Camel Milk Proteins. J. Dairy. Sci. 2019, 102(12), 10748–10759. DOI: 10.3168/jds.2019-16520.
- Baba, W. N.; Mudgil, P.; Baby, B.; Vijayan, R.; Gan, C.-Y.; Maqsood, S. New Insights into the Cholesterol Esterase- and Lipase-Inhibiting Potential of Bioactive Peptides from Camel Whey Hydrolysates: Identification, Characterization, and Molecular Interaction. J. Dairy. Sci. 2021, 104(7), 7393–7405. DOI: 10.3168/jds.2020-19868.
- Sabika, J.; Hina, K.; Priti, M.; Mohamed, H. H.; Sajid, M. Camel whey protein hydrolysates displayed enhanced cholesteryl esterase and lipase inhibitory, anti-hypertensive and anti-haemolytic properties. LWT. 2018, 98, 212–218. DOI: 10.1016/j.lwt.2018.08.024.
- Mudgil, P.; Baba, W. N.; Kamal, H.; FitzGerald, R. J.; Hassan, H. M.; Ayoub, M. A.; Gan, C.-Y.; Maqsood, S. A Comparative Investigation into Novel Cholesterol Esterase and Pancreatic Lipase Inhibitory Peptides from Cow and Camel Casein Hydrolysates Generated Upon Enzymatic Hydrolysis and in-Vitro Digestion. Food Chem. 2022, 367, 130661. DOI: 10.1016/j.foodchem.2021.130661.
- Siow, H.-L.; Choi, S.-B.; Gan, C.-Y. Structure–Activity Studies of Protease Activating, Lipase Inhibiting, Bile Acid Binding and Cholesterol-Lowering Effects of Pre-Screened Cumin Seed Bioactive Peptides. J. Funct. Food. 2016, 27, 600–611. DOI: 10.1016/j.jff.2016.10.013.
- Prados, I. M.; Marina, M. L.; García, M. C. Isolation and Identification by High Resolution Liquid Chromatography Tandem Mass Spectrometry of Novel Peptides with Multifunctional Lipid-Lowering Capacity. Food Res. Int. 2018, 111, 77–86. DOI: 10.1016/j.foodres.2018.05.009.
- Mostafa, H.; Al-Ahbabi, N.; Adiamo, O. Q.; Mudgil, P.; Maqsood, S. Phoenix Dactylifera L. Seed Protein Hydrolysates as a Potential Source of Peptides with Antidiabetic and Anti-Hypercholesterolemic Properties: An in vitro Study. Food Biosci. 2022, 49, 101916. DOI: 10.1016/j.fbio.2022.101916.
- Fisayo Ajayi, F. Identification and Characterization of Cholesterol Esterase and Lipase Inhibitory Peptides from Amaranth Protein Hydrolysates; X: Food Chemistry, 2021. Vol. 12, p. 100165.
- Garzón, A. G., Cian, R E., Aquino, M E., Drago, S R. Isolation and Identification of Cholesterol Esterase and Pancreatic Lipase Inhibitory Peptides from Brewer’s Spent Grain by Consecutive Chromatography and Mass Spectrometry. Food Funct. 2020, 11(6), 4994–5003. 10.1039/D0FO00880J
- Ferreiro, N.; Rodrigues, N.; Veloso, A. C. A.; Fernandes, C.; Paiva, H.; Pereira, J. A.; Peres, A. M. Impact of the Covering Vegetable Oil on the Sensory Profile of Canned Tuna of Katsuwonus Pelamis Species and Tuna’s Taste Evaluation Using an Electronic Tongue. Chemosensors. 2022, 10(1), 18. DOI: 10.3390/chemosensors10010018.
- Ali, M. Y.; Jung, H. J.; Jannat, S.; Jung, H. A.; Choi, J. S. In vitro Antidiabetic and Antioxidant Potential of the Ethanolic Extract of Skipjack Tuna (Katsuwonus Pelamis) Heart. J. Food Biochem. 2016, 40(4), 583–592. DOI: 10.1111/jfbc.12257.
- Chi, C.-F.; Hu, F.-Y.; Wang, B.; Li, Z.-R.; Luo, H.-Y. Influence of Amino Acid Compositions and Peptide Profiles on Antioxidant Capacities of Two Protein Hydrolysates from Skipjack Tuna (Katsuwonus Pelamis) Dark Muscle. Mar. Drugs. 2015, 13(5), 2580–2601. DOI: 10.3390/md13052580.
- Qiao, Q.-Q.; Luo, Q.-B.; Suo, S.-K.; Zhao, Y.-Q.; Chi, C.-F.; Wang, B. Preparation, Characterization, and Cytoprotective Effects on HUVECs of Fourteen Novel Angiotensin-I-Converting Enzyme Inhibitory Peptides from Protein Hydrolysate of Tuna Processing By-Products. Front. Nutrit. 2022, 9, 9. DOI: 10.3389/fnut.2022.868681.
- Mongkonkamthorn, N.; Malila, Y.; Yarnpakdee, S.; Makkhun, S.; Regenstein, J. M.; Wangtueai, S. Production of Protein Hydrolysate Containing Antioxidant and Angiotensin -I-Converting Enzyme (ACE) Inhibitory Activities from Tuna (Katsuwonus Pelamis) Blood. Processes. 2020, 8(11), 1518. DOI: 10.3390/pr8111518.
- Intarasirisawat, R.; Benjakul, S.; Wu, J.; Visessanguan, W. Isolation of Antioxidative and ACE Inhibitory Peptides from Protein Hydrolysate of Skipjack (Katsuwana Pelamis) Roe. J. Funct. Food. 2013, 5(4), 1854–1862. DOI: 10.1016/j.jff.2013.09.006.
- Zhang, L.; Zhao, G.-X.; Zhao, Y.-Q.; Qiu, Y.-T.; Chi, C.-F.; Wang, B. Identification and Active Evaluation of Antioxidant Peptides from Protein Hydrolysates of Skipjack Tuna (Katsuwonus Pelamis) Head. Antioxidants. 2019, 8(8), 318. DOI: 10.3390/antiox8080318.
- Wang, Y.-M.; Li, X.-Y.; Wang, J.; He, Y.; Chi, C.-F.; Wang, B. Antioxidant Peptides from Protein Hydrolysate of Skipjack Tuna Milt: Purification, Identification, and Cytoprotection on H2O2 Damaged Human Umbilical Vein Endothelial Cells. Process Biochem. 2022, 113, 258–269. DOI: 10.1016/j.procbio.2022.01.008.
- Ding, D.; Du, B.; Zhang, C.; Zaman, F.; Huang, Y. Isolation and Identification of an Antioxidant Collagen Peptide from Skipjack Tuna (Katsuwonus Pelamis) Bone. Rsc. Adv. 2019, 9(46), 27032–27041. DOI: 10.1039/C9RA04665H.
- Zhou, C.; Hu, J.; Ma, H.; Yagoub, A. E. A.; Yu, X.; Owusu, J.; Ma, H.; Qin, X. Antioxidant Peptides from Corn Gluten Meal: Orthogonal Design Evaluation. Food Chem. 2015, 187, 270–278. DOI: 10.1016/j.foodchem.2015.04.092.
- Zhang, Z.; Jiang, S.; Zeng, Y.; He, K.; Luo, Y.; Yu, F. Antioxidant Peptides from Mytilus Coruscus on H2O2-Induced Human Umbilical Vein Endothelial Cell Stress. Food Biosci. 2020, 38, 100762. DOI: 10.1016/j.fbio.2020.100762.
- Zhang, Z.; Hu, X.; Lin, L.; Ding, G.; Yu, F. Immunomodulatory Activity of Low Molecular-Weight Peptides from Nibea Japonica in RAW264.7 Cells via NF-Κb Pathway. Mar. Drugs. 2019, 17(7), 404. DOI: 10.3390/md17070404.
- Jiang, S.; Zhang, Z.; Yu, F.; Zhang, Z.; Yang, Z.; Tang, Y.; Ding, G. Ameliorative Effect of Low Molecular Weight Peptides from the Head of Red Shrimp (Solenocera Crassicornis) Against Cyclophosphamide-Induced Hepatotoxicity in Mice. J. Funct. Foods. 2020, 72, 104085. DOI: 10.1016/j.jff.2020.104085.
- Du, X.; Bai, M.; Huang, Y.; Jiang, Z.; Chen, F.; Ni, H.; Li, Q. Inhibitory Effect of Astaxanthin on Pancreatic Lipase with Inhibition Kinetics Integrating Molecular Docking Simulation. J. Funct. Foods. 2018, 48, 551–557. DOI: 10.1016/j.jff.2018.07.045.
- Karami, Z.; Butkinaree, C.; Yingchutrakul, Y.; Simanon, N.; Duangmal, K. Comparative Study on Structural, Biological and Functional Activities of Hydrolysates from Adzuki Bean (Vigna Angularis) and Mung Bean (Vigna Radiata) Protein Concentrates Using Alcalase and Flavourzyme. Food Res. Int. 2022, 161, 111797. DOI: 10.1016/j.foodres.2022.111797.
- Chen, H.; Qi, X.; Guan, K.; Gu, Y.; Wang, R.; Li, Q.; Ma, Y. Peptides Released from Bovine α-Lactalbumin by Simulated Digestion Alleviated Free Fatty Acids-Induced Lipid Accumulation in HepG2 Cells. J. Funct. Foods. 2021, 85, 104618. DOI: 10.1016/j.jff.2021.104618.
- López-Pedrouso, M.; Borrajo, P.; Pateiro, M.; Lorenzo, J. M.; Franco, D. Antioxidant Activity and Peptidomic Analysis of Porcine Liver Hydrolysates Using Alcalase, Bromelain, Flavourzyme and Papain Enzymes. Food Res. Int. 2020, 137, 109389. DOI: 10.1016/j.foodres.2020.109389.
- Wang, Z.; Liu, X.; Xie, H.; Liu, Z.; Rakariyatham, K.; Yu, C.; Shahidi, F.; Zhou, D. Antioxidant Activity and Functional Properties of Alcalase-Hydrolyzed Scallop Protein Hydrolysate and Its Role in the Inhibition of Cytotoxicity in vitro. Food Chem. 2021, 344, 128566. DOI: 10.1016/j.foodchem.2020.128566.
- Du, Z.; Li, Y. Review and Perspective on Bioactive Peptides: A Roadmap for Research, Development, and Future Opportunities. J. Agric. Food Res. 2022, 9, 100353. DOI: 10.1016/j.jafr.2022.100353.