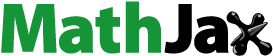
ABSTRACT
Ersho, an undefined starter culture used for Ethiopian injera production, is well-known as a source of yeasts. However, there has not been negligible research done on the use of yeasts isolated from ersho samples for making bread. So, the present study was aimed at isolating and screening yeasts from ersho samples and evaluating their impact on the nutritional, microbial, and sensory quality of bread. A total of 55 ersho samples were collected from different locations in Ethiopia. An experimental research design was used. Three yeast isolates (4A, 14D, and 54A) were selected from a total of 220 yeast isolates. Yeast isolates 4A, 14D, 54A, 14D54A, and bakery yeast were used to make BY1, BY2, BY3, BY4, and control breads, respectively. Compared to other breads, BY4 exhibited superior qualities. Protein, TCC (total coliform count), TVC (total viable count), and TFC (total fungal count) of control bread were 13.56%, 0, 3.1, and 2.1 log cfu/g, respectively. Protein,TCC, TVC, and TFC of BY4 bread were 14.84%, 0, 2.4, and 1.6 log cfu/g, respectively. Color, texture, taste, odor, and overall acceptability mean scores of control bread were 6.6, 6.7, 6.9, 7.1, and 6.9, respectively, while those of BY4 bread were 6.8, 7.2, 7.2, 7.3, and 7.3, respectively. The shelf lives of the control and BY4 breads were 4 and 6 days, respectively. The results of the present study indicate that the wild yeasts isolated from the ersho samples can be used for the development of bakery yeast.
KEYWORDS:
Introduction
Around the world, bread has been regarded as a crucial component of practically every human diet.[Citation1] In all of its forms, bread is an essential component of the human diet, but for many people, it represents much more. It has a wealth of nutrients, including vitamins, minerals, and carbohydrates (which provide energy). The bread supplies roughly 10% of a person’s daily requirement for protein, minerals (such as iron, zinc, and copper), vitamins (such as thiamine and niacin), and necessary fiber, depending on its kind and origin.[Citation2]
Wheat, water, a leavening agent, salt, sugars, fats or oils, and optional additives are just a few of the ingredients used to make bread. The ingredients are skillfully prepared to give the bread’s nutrients, flavor, aroma, structure, and texture. Bread leavening agents, either biological or chemical, must be used to raise flour dough. Biological leavening agents are microorganisms that convert the carbohydrates in the dough into carbon dioxide gas, which causes the dough to expand before baking.[Citation3] The yeast that is frequently employed as a biological leavening agent in baking bread and other bakery products is known by the common name “baker’s yeast” (Saccharomyces cerevisiae). It performs crucial functions in the baking of bread, such as raising the flour dough volume by producing carbon dioxide, maintaining the flavor of the bread by creating a variety of flavor compounds, assisting in the development of the dough’s structure and texture by stretching due to the expansion of gas bubbles, and producing some nutritional benefits from the bread through its fermentation.[Citation4]
Despite the widespread production and consumption of baked goods, notably breads, Ethiopia struggles to create bread due to a shortage of manufacturers of bakery yeast plants. Imported commercial bakery yeasts were used to make bread and other baked goods. Due to the rising demand for bread, the bakery industry is expanding steadily, and commercial bakery yeast imports are rising as well. In order to import commercial bakery yeasts for baking bread, Ethiopia used up 213,227,807 ETB (Ethiopian birr) in 2015 and 293,010,632 ETB in 2016.[Citation5] Despite not having developed a bakery yeast production facility, Ethiopia is rich in a variety of yeasts. To date, there has been no a research done for comparing yeast starter cultures obtained from ersho for bread-making. In order to develop yeast starter cultures from ersho samples for manufacturing bread in Ethiopia, the current study was conducted.
Ersho is a traditional Ethiopian sourdough. It is a fluid saved from previously fermented Ethiopian injera dough. It is a spontaneously fermented product. It is used as a back-sloping starter culture for making Ethiopian injera.[Citation6] Ersho is well known as a source of yeasts.[Citation5,Citation7] However, the utilization of yeasts isolated from ersho samples for bread fermentation has not been previously studied.
Materials and methods
Study areas
The study areas are Gondar, Bahir Dar, Debre Markos, Addis Ababa, and Debre Berhan cities, which are located in Ethiopia.
Research design
The experimental research design was used to isolate, screen, and identify yeasts from ersho samples for evaluation of their impact on the nutritional, microbial, and sensory quality of bread from 2021 to 2024.
Sample collection
A total of 55 ersho samples were collected from households in the study areas. Sterilized bottles were used to collect the ersho samples. The collected ersho samples were transported to the Biotechnology Laboratory, Gondar University, Ethiopia for isolation, screening, identification, and application of yeast isolates for bread production. Sample numbers from 1–15, 16–25, 26–35, 36–45, and 46–55 were collected from Gondar, Bahir Dar, Debre Markos, Addis Ababa, and Debre Berhan cities, respectively ().
Table 1. Description of the study areas and number of samples collected from them.
Isolation and purification of yeasts
The collected ersho samples were homogenized by vortexing before serial dilution. Then, a ten-fold serial dilution was prepared for each collected ersho sample by using saline solution. From the prepared serial dilution (10−3-10−5), 0.1 ml of suspension was spread on the surface of pre-solidified yeast extract peptone dextrose agar plates (YPD) (yeast extract 10 g/L, peptone 20 g/L, dextrose 20 g/L, chloramphenicol 0.05 g/L, agar 20 g/L, pH 5.6). The plates were incubated at 30°C for 3 days. After incubation, a total of 220 colonies (4 colonies from each sample were taken and designated as A, B, C, and D) were selected randomly and purified by repeating sub-culturing on YPD agar plates.[Citation8]
Screening yeast isolates
Gas production, yeast growth rate, acid tolerance,[Citation9] hydrogen sulfide production, stress exclusion test, flocculation test, temperature tolerance, carbohydrate utilization capacity, osmotic tolerance,[Citation10] ethanol tolerance,[Citation11] and dough raising capacity[Citation12] were used for screening yeast isolates.
Safety and beneficial role of yeast isolates
Three yeast isolates (4A, 14D, and 54A) were screened from 220 yeast isolates and evaluated for their safety and beneficial roles. Yeast isolates 4A and 14D were obtained from ersho samples collected from Gondar city, while yeast isolate 55A was obtained from ersho samples collected from Debre Berhan city. Hemolytic activity,[Citation13] gelatinase activity,[Citation14] biogenic amine production tests,[Citation15] and antibiotic resistance tests[Citation16] were used to assess the safety of yeast isolates. Beneficial roles of yeast isolates like antimicrobial activity,[Citation17–19] proteolytic activity,[Citation19] and exopolysaccharide production[Citation20] were measured.
Identification of yeast isolates
Phenotypic characterization
Yeast phenotypic characterization was done using colony morphology, cellular morphology, carbohydrate fermentation, carbohydrate assimilation, nitrogen assimilation, growth of yeast isolates in 50% glucose, and growth of yeast isolates at 37°C.[Citation21]
Genotypic characterization
DNA extraction was done according to the protocol of the Gen Elute genomic DNA purification kit. After the extraction of DNA, they were subjected to polymerase chain reaction amplification. Internally transcribed spacer regions (ITS1 and ITS4) of 5.8S ribosomal DNA (5.8S rDNA) were amplified using a universal primer. Conventional PCR (country: UK, brand: TC-412) was used. The reaction mixture for PCR amplification for each yeast isolate DNA was prepared using 20 μl volume containing 0.4 μl ITS-1 primer (5”-TCC GTA GGT GAA CCT GCG G-3‘), 0.4 μl ITS-4 primer (5’-TCC TCC GCT TAT TGA TAT GC-3”), 4.0 μl master mix (BioDyne5×FIREPolR master mix), 12.2 μl PCR water, and 3.0 μl DNA sample. The PCR conditions were an initial denaturation at 95°C for 5 min, followed by 30 cycles of denaturation at 95°C for 30 sec, annealing at 56°C for 40 seconds, extension at 72°C for 1 min, and final extension at 72°C for 5 min. DNA extraction products and PCR products were separated on a 1% agarose gel containing 3 μl of ethidium bromide and visualized under UV light (gel documentation system).[Citation22]
The PCR products were sequenced. After obtaining DNA sequencing, the sequencing errors were edited using BioEdit Sequence. After editing, the DNA sequences of yeast isolate DNA samples were compared with GenBank National Center for Biotechnology Information (NCBI) reference sequences by using the basic alignment search tool (BLAST). Blasted sequences were aligned using Clustal W. The phylogenetic tree of yeast isolates was constructed using molecular evolutionary genetic analysis 7 (MEGA 7), using a maximum likelihood algorithm character estimation method.[Citation22]
Evaluation of selected yeast isolates for making bread
Bread production process
The selected yeast isolates (4A, 14D, and 54A) and their combinations were evaluated for their dough-raising capacity. Single isolates (4A, 14D, and 54A) and a combination isolate (14D54A) showed the highest dough-raising capacity (), and they were used for bread production. Using selected yeast isolates, five types of bread were prepared in triplicate. BY1: bread made with yeast isolate 4A; BY2: bread made with yeast isolate 14D; BY3: bread made with yeast isolate 54A; BY4: bread made with yeast isolates 14D and 54A; and control: bread made with commercial bakery yeast, cy2.
Selected yeast isolates and commercial yeast (control) were grown in YPD broth for 72 hr at 30°C. The cultures were centrifuged for 25 min at 5,000 rpm, washed twice with saline water, and the supernatants were discarded. The pellets were weighed and used as yeast starter culture for making bread. Bread was prepared by using wheat flour (50 g), yeast pellet (0.6 g), table sugar (3 g), salt (0.5 g), and distilled water (40 ml). These ingredients were mixed and incubated at 30°C for 3 hr. Then, the dough samples were baked in a Microwave oven (country: India, brand: MC2846BV) at 180°C for 20 minutes.[Citation10]
Bread quality analysis
Physical properties
Breads’ volume and weight were measured after breads cooled at room temperature for 3 hr. For measuring bread volume, the seed displacement method was followed. Can lid was filled with rice grains. The grains were poured out of the can lid. The bread was put in cans with lid. The can lid was refilled with rice grains. The excess grains were measured in a measuring cylinder, and they were considered the volume of the bread. The weight of the bread was measured using a balance.[Citation23] Bread specific volume was calculated by dividing bread volume by bread weight. Two grams of bread samples were ground using a mortar and pestle. Then, they were transferred into falcon tubes, which contain 20 ml of distilled water. The mixture was shaken and placed at room temperature for 3 hr. Then, the bread’s pH was measured by a pH meter.[Citation24] Two grams of dried bread samples (dried at 80°C until constant weight was obtained) and 10 ml of 0.1 N sodium bicarbonate were mixed for 2 min and left for 20 min at room temperature. Then, the mixture was centrifuged at 3000 rpm for 15 min and the supernatant was discarded. The pellet was drained at a 45-degree angle for 10 min. Then, it was weighted, dried, and weighted.[Citation25]
Where W2: weight of tube plus drained sample, W1: weight tube plus dried sample and W3: weight of sample.
where n time of incubation
Microbial analysis
Ten-fold serial dilution of bread samples was prepared, and 0.1 ml bread suspension was spread on the surface of plate count agar plates (PCA), violet red bile agar plates (VRBA), and potato dextrose agar plates (PDA +0.01% chloramphenicol) for counting total viable counts, total coliforms, and total fungi, respectively. The PCA and VRBA plates were incubated at 37°C for 48 hr while the PDA plates were incubated at 28°C for 5 days. After incubation, the colonies were counted using a colony counter and reported as colony-forming units per gram (cfu/g).[Citation26]
Sensory analysis
Twenty participants (10 bread sellers and 10 customers) were used to evaluate the taste, odor, texture, color, and general acceptance of the bread samples. For sensory evaluation, a nine-point hedonic scale was employed: Dislike extremely: 1, dislike very much: 2, dislike moderately: 3, dislike slightly: 4, neither like nor dislike: 5, like slightly: 6, like moderately: 7, like very much: 8, and like extremely: 9. Before and after testing each piece of bread, the judges were asked to rinse their mouths with water.[Citation27]
Proximate analysis
The nutrient contents of fresh baked breads were determined. Protein,[Citation28] fat, carbohydrate, ash, and moisture content of the bread were measured.[Citation29]
Shelf life analysis
The bread samples were placed in sterile polyethylene bags and stored at room temperature until mold growth was observed visually on the surface of the bread samples. pH, titratable acidity, alkaline water retention capacity, staling rate, sensory, and microbial analyses were done during the storage period at a two-day interval. Visual observation of bread was carried out every day.
Data analysis
In terms of mean and standard deviation, the effect of yeast isolates on the physicochemical, microbiological, and sensory quality of bread was determined. The mean and standard deviation were calculated using SPSS version 25. The presence or absence of statistically significant differences among yeast starters with regard to their impacts on bread quality was measured using post hoc (Duncan) analysis. The significance level was established at p ≤ .05.
Results
Isolation and purification of yeast isolates
A total of 55 ersho samples were collected from the study sites. Four colonies (designated as A, B, C, and D) were randomly selected and isolated from each ersho sample. A total of 220 yeast colonies were purified from 55 ersho samples.Yeast extract peptone dextrose agar plates supplemented with chloramphenicol (0.01%) were used for the isolation and purification of yeast isolates.
Screening yeast isolates
Yeast isolates had different degrees of gas production capacity, and they were grouped as follows: 4 isolates were strong gas producers, 57 isolates were moderate gas producers, 154 isolates were weak gas producers, and 5 isolates were none gas producers. Four strong gas-producing and thirty-five moderate gas-producing yeast isolates were selected for further testing ().
Table 2. Screening, safety, and beneficial role of yeast isolates.
Yeast isolates show varying degrees of hydrogen sulfide (H2S) production, such as 18 isolates that were none H2S producers (green), 2 isolates that were low H2S producers (light brown), 14 isolates that were high H2S producers (dark brown), and 5 isolates that were very high H2S producers (black color). None of the H2S producers (18 isolates) and the low H2S producers (2 isolates) were selected for further testing ().
Yeast isolates exhibited different growth rates as measured by their optical density (OD). Twelve (12) isolates exhibited moderate growth rates and intermediate biomass yields (OD value 1.3–1.69), and eight (8) yeast isolates exhibited high growth rates, which showed a high biomass yield (OD value ≥ 1.7). A high growth rate of eight yeast isolates was selected for further testing ().
Various yeast isolates exhibited varying maximum dough-raising volumes and times needed to reach such levels. The range of the single yeast isolates’ dough-raising capacity was 121 to 143%, with an average of 134% . The rise in dough volume was almost higher in dough fermented with mixed yeasts than in dough fermented with a single yeast isolate ().
Safety and beneficial role of yeast isolates
All yeast isolates showed negative responses to hemolytic activity, gelatinase activity, and biogenic amine production. There was no clear halo zone, liquified broth, or purple color formation in blood agar plates, gelatin broth, or amino acid broth, respectively (). All yeast isolates were antibiotic-resistant (Table S5).
All yeast isolates showed proteolytic activity and antimicrobial activity against pathogens like Salmonella typhimurium, Shigella dysenteriae, Staphylococcus aureus, Escherichia coli, and Candida albicans. All yeast isolates produce exopolysaccharides. Yeast isolate 4A was the highest exopolysaccharide producer, while yeast isolate 14D was the least exopolysaccharide producer ().
Factors affecting the growth of yeast isolates
Yeast isolates grew at different pH values and showed different responses. As the pH increased from highly acidic to less acidic, their growth increased, and vice versa. There was a significant difference in the growth rate of yeast isolate 4A in all pH values at p ≤ .05 (). Yeast isolates could grow at 25, 30, 37, and 40°C. All yeast isolates were not grown at 45°C. The optimum temperature for their maximum growth was 30°C. The growth of yeast isolate 14D was significantly higher at temperature 30°C than at other temperature values at p ≤ .05 ().
Table 3. Factors affect the growth of yeast isolates (ethanol, osmosis (log cfu/g), pH, T0 (OD)).
All yeast isolates were grown at 6, 10, and 15% ethanol concentrations. The maximum growth of yeast isolate 4A was observed at a 10% ethanol concentration, while the maximum growth of other yeast isolates was observed at a 6% ethanol concentration. There were significant differences among the growth of yeast isolates for their ethanol tolerance at p ≤ .05 (). Dextrose and sucrose were the major sugars that were utilized by yeast isolates as carbon sources. Some yeast isolates utilized fructose and galactose as major sugars, while others utilized them as low-carbon sources. Yeast isolate 54A utilized maltose, while others did not. All yeast isolates did not utilize lactose ().
Identification of yeast isolates
All yeast isolates had a circular shape, an entire margin, a white color, budding replication, and could grow in 50% glucose and at body temperature. Both yeast isolates 14D and 54A were small in size and had umbonate elevation morphology. Yeast isolates 4A and 14D had a sphere-shaped cellular morphology, while the 54A yeast isolate had an elliptical cellular morphology ().
Table 4. Morphological, physiological and molecular identification of yeast isolates.
Glucose, galactose, fructose, maltose, and sucrose were fermented and assimilated by all yeast isolates. Lactose, arabinose, mannose, and starch were not assimilated by all yeast isolates. All yeast isolates did not ferment and assimilate lactose sugar. All yeast isolates produced acids from arabinose and mannose sugars but not gas ().
Molecular identification was done for the selected three yeast isolates (4A, 14D, and 54A), and they were identified as Saccharomyces cerevisiae isolate KRSAN1, Saccharomyces cerevisiae strain JYC2577, and Kazachstania humilis clone Turkey strain 270_M45B3–3, respectively (). The evolutionary relationship of yeast isolates is presented in .
Evaluation of bread quality made with yeast starters
Physical properties of breads
In the present study, the specific volume of all breads was higher than that of control bread. The mean specific volumes of BY1, BY2, BY3, BY4, and control breads were 3.6, 3.9, 3.6, 4.1, and 3.5 ml/g, respectively (). At 0 day bread storage, the highest pH was observed in control bread, while the least pH was observed in bread BY4. The alkaline water retention capacity (AWRC) of BY4 bread was the highest, and the AWRC of BY3 bread was the least. There were significantly differences in pH and AWRC values for breads at p ≤ .05. The pH of control bread and the AWRC of BY4 bread were significantly higher than other breads at p ≤ .05 (). During bread storage, the pH and AWRC of the breads decreased while the staling rate (SR) of the breads increased. Except for bread BY4, there were significantly different in pH, AWRC, and SR of breads during bread storage at p ≤ .05. The AWRC of bread storage during the first day, second day, fourth day, and sixth day were 307, 210, 188, and 157%, respectively ().
Figure 3. Specific volume of breads. The numbers with different letters are significantly different with respect to volume, weight and specific volume at p ≤ .05.

Table 5. pH, AWRC (%) and SR (%) of breads during bread storage.
Microbial load of breads
Total viable count (TVC) and total fungal count (TFC) were measured during the storage of bread, but total coliform count was not detected. Visual observation of mold growth on the surface of the bread was used to determine the shelf life of the bread. When mold growth begins visual observation on the surface of bread, it was spoiled. Based on this principle, the shelf lives of the control, BY1, BY2, BY3, and BY4 breads were 4, 6, 5, 4, and 6 days, respectively (). At 0 day storage, TVC of control, BY1, BY2, BY3, and BY4 breads were 3.1, 2.5, 2.6, 3.2, and 2.4 log cfu/g, respectively. The TVC of the overall mean of the breads was 2.7 log cfu/g. At 0 day storage, the TFC of control, BY1, BY2, BY3, and BY4 breads were 2.1, 1.9, 2.0, 2.1, and 1.6 log cfu/g, respectively. The TFC of the overall mean of the breads was 1.9 log cfu/g. All BY1, BY2, BY3, BY4, and control breads were full-filled to the microbial standard limit, and they were consumer-acceptable breads. The TVC of BY3 and control breads were significantly higher than other breads at p ≤ .05 ().
Table 6. TCC, TVC and TFC (log cfu/g) of breads during bread storage.
When the bread storage period was increased, the total viable count (TVC) and total fungal count (TFC) of breads increased. The TVC and TFC of breads were increased from 2.7 to 5.3 and 1.9 to 2.5 log cfu/g, respectively. There was a significant difference among the TVC of BY1, BY2, BY3, BY4, and control breads during the 0, 2, 4, and 6-day bread storage periods at p ≤ .05 ().
Sensory analysis of breads
Almost all the sensory properties of the breads were improved in terms of color, texture, taste, aroma, and overall acceptability when they were compared with control bread (bread made with bakery yeast). During bread storage, the color, texture, taste, odor, and overall acceptability of breads decreased. During 0 day storage, bread sensory properties were measured. The highest color, texture, taste, odor, and overall acceptability scores were observed in breads BY4, BY4, BY1, BY1, and BY4, respectively. The overall acceptability scores of BY1, BY2, BY3, BY4, and control breads were 7.1, 6.6, 6.8, 7.3, and 6.9, respectively (). shows the types of breads made with different wild yeasts (isolated from ersho samples) and commercial bakery yeast.
Figure 4. Breads made with different yeast starters. BY1, BY2, BY3, BY4, control breads made using yeast isolates 4A, 14D, 54A, 14D54A, and commercial bakery yeast, respectively.

Table 7. Mean scores of sensory quality of bread samples during storage.
Bread sensory properties like color, texture, taste, odor, and overall acceptability decreased when the storage period of bread was increased. During 4 days of storage, control bread’s color, texture, odor, and overall acceptability mean scores decreased from 6.6 to 5.1, from 6.7 to 4.2, from 7.1 to 4.7, and from 6.9 to 4.5, respectively. During bread storage, the sensory properties of bread’s color score decreased from 6.5 to 3.6; texture score decreased from 7.0 to 3.9; taste score decreased from 7.0 to 3.7; odor score decreased from 7.1 to 3.7; and overall acceptability score decreased from 7.0 to 3.5. Sensory properties of bread storage at 0 and 2 days showed almost no significant differences among bread sensory properties at p > .05 ().
Proximate analysis
The protein, ash, fat, and moisture content of breads improved when they were compared with control bread. But the carbohydrate content of breads decreased when they were compared with control bread, except BY2 bread. The overall mean protein, fat, ash, and moisture content of breads were 14.15, 1.27, 1.40, and 22.85%, respectively ().
Discussion
Food quality, like nutrition, sensory, and microbial quality, was determined through their ingredients and the type of starters used. The use of different starters in food production resulted in food quality variations. Bread made with a mixture of Saccharomyces cerevisiae (F514) and commercial baker’s yeast had the highest scores in texture, taste, and overall acceptability mean scores (14.13, 18.5, and 92, respectively), followed by bread made with S. cerevisiae (F707) (13.25, 17.5, and 87.63, respectively), whereas minimum scores were recorded in bread made with S. cerevisiae (F514) (12.25, 16.25, and 81.13, respectively).[Citation30]
The dough sample fermented with yeast isolate CS2 had the highest leavening activity, followed by PW7 and commercial yeast (control). The bread made with yeast isolate PW7 had the highest values of moisture (24.10%), protein (4.90%), and fat (7.45%), while bread made with AC1 had the highest value of carbohydrate (89.11%). Bread made with yeast isolate AC2 had a better appearance, texture, and taste than bread made with other yeast isolates.[Citation4]
In the present study, different yeast starters showed different bread quality in terms of nutrition, sensory, and microbial quality. The reason might be that yeast starter diversity is high. Because yeast starters showed variation in exopolysaccharide production, antimicrobial activity, organic acid production, gas production, and proteolytic activity
Yeast isolates showed different gas production capacities (strong, medium, low, and none). Based on gas production, 4 strong gas producers and 35 medium gas producers were selected from 220 yeast isolates for further testing (). Gas production is the basic criteria for selecting high-performing bakery yeasts.[Citation31]
Yeast isolates showed a very strong, strong, low, and none response to hydrogen sulfide production. Twenty isolates (18 isolates were none-H2S producers and 2 isolates were low H2S producers) were selected from 39 yeast isolates for further testing (). This result was comparable to the work of Tika et al.[Citation10] who evaluated both none-H2S producers and low-H2S producers for bread production. Strong and very strong H2S-producing yeast isolates were not recommended for use in bread production. This is a result of the high levels of H2S in food, which give the food an unpleasant odor and flavor.
The maximum dough-raising volumes and times required to reach such levels varied among different yeast isolates. The single yeast isolates’ capacity to raise dough ranged from 121 to 143%, with a mean value of 134% (). This result was higher than the finding of Zotta et al.[Citation32] [Citation33]; * who reported that the mean dough volume raising capacity of yeasts was 105%. However, this result was lower than the finding of Olabisi et al.[Citation11] who reported that the average dough volume-raising capacity of yeasts was 165%.
In comparison to dough fermented with a single yeast isolate, almost all dough fermented with a mixture of yeasts rose in volume more (). This result was consistent with the conclusion reached by Liliane et al.[Citation13] The ability of yeast isolates to raise dough varied. The diversity of yeast species, which affects how much gas is produced, may be the cause of this variation.[Citation34]
Different temperatures and pH levels were used to grow different yeast isolates, and they responded differently. Their growth increased when the pH value decreased from high to low, and vice versa. Their maximum growth occurred at a temperature of 30°C (). These results were consistent with what was reported in Onyimba et al.’s findings.[Citation35] However, these results were in contrast to those of Umeh et al.,[Citation29] who stated that some yeast isolates could grow at 45°C.
All yeast isolates were grown at 6, 10, and 15% ethanol concentrations. The maximum growth of yeast isolate 4A was observed at a 10% ethanol concentration, while the maximum growth of other yeast isolates was observed at 6% ethanol (). These results were similar to the work of Onyimba et al.[Citation35] but the results disagreed with the finding of Umeh et al.[Citation29] who reported that all yeast isolates were not grown at a 15% ethanol concentration.
Dextrose and sucrose were the major sugars that were utilized by yeast isolates as carbon sources. All yeast isolates did not utilize lactose (). This result was in agreement with the finding of Tika et al.[Citation10] but the result disagreed with the finding of Sefinew et al.[Citation36] as all yeast isolates did not utilize dextrose. According to Tsegaye et al.[Citation37] who reported that some yeast isolates did not utilize dextrose, sucrose, and fructose as carbon sources,
Three yeast isolates (4A, 14D, and 54A) were screened and used to make breads. BY1, BY2, BY3, BY4, and control breads were made using yeast isolates 4A, 14D, 54A, 14D54A, and cy2 (commercial bakery yeast), respectively. The mean specific volumes of BY1, BY2, BY3, BY4, and control breads were 3.6, 3.9, 3.6, 4.1, and 3.5 ml/g, respectively. The overall mean specific volume of the breads was 3.8 ml/g (). This result was higher than the finding of Arjun et al.[Citation38] who reported the breads specific volume was 3.1 ml/g but this result was lower than the finding of Mostafa et al.[Citation39] who reported the breads’ specific volume was 7.1 ml/g. Differences in carbon dioxide production capacity,[Citation40] and variations in the nutrient content of bread flour (40) may be the causes of variations in bread-specific volume.
The pH and alkaline water retention capacity (AWRC) of the breads decreased while their staling rate (SR) increased during storage. The AWRC of bread during 0 day, 2nd day, 4th day, and 6th day storage were 307, 210, 188, and 157%, respectively (). This result was in accordance with the finding of Mostafa et al.[Citation39] who reported that the AWRC of bread during 0 day, 1st day, 2nd day, and 3rd day storage were 382, 323, 286 and 268%, respectively. The reasons for bread’s physical property variation might be differences in yeast diversity,[Citation30] degree of exopolysaccharide production variation, and differences in protein, fat, and moisture content of the breads.[Citation41]
Total viable count (TVC) and total fungal count (TFC) were measured during the storage of bread, but total coliforms were not counted. The shelf lives of the control, BY1, BY2, BY3, and BY4 breads were 4, 6, 5, 4, and 6 days, respectively (). The shelf lives of the breads were varied. Differences in antimicrobial metabolite production capacity[Citation42] and differences in the way of controlling mechanisms[Citation43] may be the causes.
The total viable count (TVC) of bread at 0 day storage ranged from 2.4 to 3.1 log cfu/g, with 2.7 log cfu/g being the average across all breads (). This result was similar to the finding of Roya et al.[Citation44] who stated that breads had a TVC of 2.7 log cfu/g. This result was lower than the finding of Sasikala et al.[Citation45] who reported that the TVC of breads was 3.7 log cfu/g. This result was contradicted by the finding of Ayoade et al.[Citation46] who observed that the TVC of breads was zero.
The average total fungal count (TFC) of BY1, BY2, BY3, and BY4 breads at zero-day storage was 1.9 log cfu/g (). This result was higher than the finding of Sasikala et al.[Citation45] who reported that the TFC of breads was 1.6 log cfu/g. However, it was lower than the finding of Zaber et al.[Citation28] who reported that the TFC of the breads was 2.9 cfu/g. This result contradicted the finding of Omorodion et al.[Citation47] who observed that the TFC of the breads was zero.
The total viable count (TVC) and total fungal count (TFC) of breads increased as the bread storage time increased. The TVC increased from 2.7 to 5.3 log cfu/g, while the TFC of breads increased from 1.9 to 2.5 log cfu/g (). These results were consistent with the findings of Omorodion et al.[Citation47] who noted that the TVC and TFC of the breads increased from 4.0 to 6.1 and 0.0 to 5.6 log cfu/g, respectively, after 8 days of bread storage.
Breads varied in their total viable and total fungal counts. The causes could be differences in hygienic practices during the bread production process and packing stages,[Citation28] purity of bread ingredients from microbe contamination and the working environment,[Citation48] and type of microbial contamination.[Citation49] Additionally, breads with a pH above 5, a high spore load, and a storage temperature over 25°C were linked to the growth of microbes and the spoilage of breads.[Citation50]
During zero-day bread storage, color, taste, odor, texture, and overall acceptability had overall mean scores of 6.5, 7.0, 7.1, 7.0, and 7.0, respectively (). These results were greater than the findings of Mehmet et al.[Citation51] who reported that the color, taste, odor, texture, and overall acceptability scores of breads were 5.4, 4.0, 4.0, 3.8, and 3.8, respectively. But these results were lower than the findings of Tadlo et al.[Citation52] who reported that the breads’ color, taste, odor, texture, and overall acceptability scores were 7.4, 7.6, 7.5, 7.5, and 7.4, respectively. Bitrus et al.[Citation23] who reported that the bread taste mean score was 7.0, which is similar to the present study result. Akinyede et al.[Citation53] who reported that the odor and texture scores of breads were 7.1, which is closely similar to the present study results. Mostafa et al.[Citation39] who reported that the overall acceptability score of breads was 8.8 which is higher than the present study result. Tamrat et al.[Citation54] who reported that the texture mean score of breads was 4.7, which is lower than the present study result.
In the present study, a mixed yeast starter performed better than a single yeast starter culture in terms of improving the sensory qualities of breads (). This result was in agreement with the finding of Sarah et al.[Citation30] who reported that mixed yeast starter cultures produced bread with better sensory qualities than single yeast starter cultures. The sensory characteristics of breads varied. The causes could be a variety of yeast strains used, variability in the bread metabolite production process,[Citation32,Citation55,Citation56] and variability in the degree of dough fermentation.[Citation57]
The sensory properties of bread decreased during storage (). This result was in accordance with the finding of Pooja et al.[Citation58] who reported that the sensory mean score of bread decreased from 7 to 6 for 10 days of bread storage. The reduction of physical and sensory properties of breads during bread storage is caused by the loss of volatile flavor molecules, off-flavor molecules formation,[Citation59] the growth of microbes that cause discoloration and slimy rope formation, and the degradation of bread by secreting different enzymes like amylase and protease enzymes.[Citation60] Additionally, proteolytic and lipid oxidation reduce the nutritional values of breads.[Citation58]
BY1, BY2, BY3, BY4, and control breads had protein contents of 14.18, 13.46, 14.11, 14.84, and 13.56%, respectively. The average protein content across the breads BY1, BY2, BY3, and BY4 was 14.15% (). This result was lower than that of Mostafa et al.[Citation39] who found that breads had a protein content of 16.24%. The findings of Tadlo et al.[Citation52] and Adamczyk et al.[Citation61] who reported that breads had protein contents of 9.47 and 9.49%, respectively, were lower than the current study result. The nutrient contents of breads varied. The reasons might be use of yeast strain diversity,[Citation30] and bread flour nutrient content variation.[Citation62]
Conclusion
In the present study, all breads made with different yeast starters (isolated from ersho samples) showed better quality in terms of nutritional, microbial, shelf life, and sensory quality compared with control bread made with commercial bakery yeast. The results of the current study indicate that ersho samples (traditional Ethiopian sourdoughs) have been proven to be a reliable source for the isolation of yeast starters for production improved bread quality. The present study was also achieved by screening three yeasts (Saccharomyces cerevisiae isolate KRSAN1, Saccharomyces cerevisiae strain JYC2577, and Kazachstania humilis) from ersho samples as suitable commercial bakery yeast starter development. However, further research will be needed to optimize biomass production and identify the type of metabolites produced by each yeast starter.
Acknowledgments
We would like to acknowledge the Department of Industrial and Environmental Biotechnology, Institute of Biotechnology for providing laboratory space, laboratory chemicals, and laboratory media for doing this research. We would also like to acknowledge the panelists who participated in this work.
Disclosure statement
No potential conflict of interest was reported by the author(s).
Additional information
Funding
References
- Scheuer, P.; Ferreira, J.; Mattioni, B.; Miranda, M.; Francisco, A. Optimization of Image Analysis Techniques for Quality Assessment of Whole-Wheat Breads Made with Fat Replacer. Food Sci. And Technol. 2015, 35(1), 133–142. DOI: 10.1590/1678-457X.6560.
- Odedeji, J.; Ojo, A.; Arogundade, L.; Oyeleke, G. Proximate Composition and Consumers Acceptability of Bread Produced from Blends of Soy-Cheese and Wheat Flour. J. Of Environ. Sci. Toxicol. Food Technol. 2014, 8(2), 2319–2399. DOI: 10.9790/2402-08224144.
- ElSheikha, A.; Montet, D. Fermented Foods—Artisan Household Technology to Biotechnology Era. 2016.
- Balarabe, M.; Sambo, S.; Mohammed, D.; Ayodeji, O. Screening of Fermentative Potency of Yeast Isolates from Indigenous Sources for Dough Leavening. Int. J. Microbiol. Biotechnol. 2017, 10(1), 33–37.
- Elsa, B. Characterization of Indigenous Wild Yeasts and Evaluation of their Bread Leavening Capacity. MSc thesis, Addis Ababa University, Addis Ababa, Ethiopia 2017.
- Alemayehu, A.; Meskerem, M.; Hailay, G. Injera Preparation from Taro (Colocasia Esculenta) and Teff (Eragrostis tef) Flour. Int. J. Sci. Basic And Appl. Res. 2016, 30, 196–204.
- Belay, T.; Andualem, B.; Anteneh, T.; Diriba, M.; Zewdu, T.; Gary, W. Molecular Characterization of Fermenting Yeast Species from Fermented Teff Dough During Preparation of Injera Using ITS DNA Sequence. Int. J. Food Sci. 2019, 2019, 1–8. DOI: 10.1155/2019/1291863.
- Mogesse, A. Microbial Flora and Some Chemical Properties of Ersho, a Starter for Teff (Eragrostis Tef) Fermentation. World J. Of Microbiol. Biotechnol. 1994, 10(1), 69–73. DOI: 10.1007/BF00357567.
- Elsa, B.; Tefera, A.; Muleta, D.; Wessel, G. Optimization of the Cultivation Conditions of Indigenous Wild Yeasts and Evaluation of Their Leavening Capacity. bioRxiv. 2019, 18, 553818.
- Tika, B.; Timilsina, P.; Yadav, A.; Pandey, G.; Joshi, Y.; Bhujel, S.; Neupane, K.; Neupane, K. Selection and Characterization of Potential Baker’s Yeast from Indigenous Resources of Nepal. Biotechnol. Res. Int. 2017, 2017, 1–10. DOI: 10.1155/2017/1925820.
- Olabisi, O. Isolation and Characterization of Palm Wine Strains of Saccharomyces cerevisiae Potentially Useful as Bakery Yeasts. Eur. J. Of Exp. Biol. 2017, 7(11), 1–13.
- Jiya, M.; Damisa, D.; Abdullahi, I.; Chukwu, V. Comparative Studies of the Dough Raising Capacity of Local Yeast Strains Isolated from Different Sources. Anchor University J. Sci. And Tecnol. 2020, 1(1), 90–95.
- Liliane, A.; Lopes, N.; Cardarelli, H. Safety and Probiotic Functionality of Isolated Goat Milk Lactic Acid Bacteria. Annals Of Microbiol. 2019, 69(13), 1497–1505. DOI: 10.1007/s13213-019-01533-z.
- Syal, P.; Vohra, A. Probiotic Potential of Yeasts Isolated from Traditional Indian Fermented Foods. Int. J. Of Microbiol. Res. 2013, 5(2), 390–398. DOI: 10.9735/0975-5276.5.2.390-398.
- Stellah, B.; Mukisa, I.; Byaruhanga, Y.; Muyanja, C. Probiotic Potential of Lactic Acid Starter Cultures Isolated from a Traditional Fermented Sorghum-Millet Beverage. Int. J. Of Microbiol. 2020, 2020, 1–13. DOI: 10.1155/2020/7825943.
- Hossain, M.; Afrin, S.; Humayun, S.; Ahmed, M.; Saha, B. Identification and Growth Characterization of a Novel Strain of Saccharomyces Boulardii Isolated from Soya Paste. Fron. Nut. 2020, 7, 27. DOI: 10.3389/fnut.2020.00027.
- Mangala, L.; Nilanjana, D. Molecular Identification of Probiotic Yeast Strains and Their Characterization. Asian J. Pharm. Clin. Res. 2017, 10(10), 339–343. DOI: 10.22159/ajpcr.2017.v10i10.20052.
- Samah, S.; Elsayed, M.; Bahout, A.; Bayoumi, M. Studies on Beneficial Yeasts Isolated from Some Egyptian Dairy Products. Zagazig Veterinary. J. 2016, 44(1), 75–84. DOI: 10.21608/zvjz.2016.7834.
- Ogunsakin, O.; Vanajakshi, V.; Anu-Appaiah, A.; Vijayendra, N.; Walde, G.; Banwo, K.; Sanni, I.; Prabhasankar, P. Evaluation of Functionally Important Lactic Acid Bacteria and Yeasts from Nigerian Sorghum as Starter Cultures for Gluten-Free Sourdough Preparation. LWT - Food Sci. Technol. 2017, 82, 326–334. DOI: 10.1016/j.lwt.2017.04.048.
- Rusinova, V.; Ognyanov, M.; Georgiev, Y.; Kambourova, M.; Adamov, A.; Krasteva, V. Production and Chemical Characterization of Exopolysaccharides by Antarctic Yeasts Vishniacozyma Victoriae and Tremellomycetes Sp. Appl. Sci. 2022, 12(4), 1805. DOI: 10.3390/app12041805.
- Supriya, N. Diversity among the yeasts isolated from naturally fermented cashew apple juice (Doctoral dissertation, Goa University) 2016.
- Angel, A.; Petrova, G.; Angelov, A. D.; Stefanova, P.; Bokossa, I.; Tchekessi, C.; Marco, M. L.; Gotcheva, V. Molecular Identification of Yeasts and Lactic Acid Bacteria Involved in the Production of Beninese Fermented Food Degue. Open Biotechnol. J. 2017, 11(1), 94–104. DOI: 10.2174/1874070701711010094.
- Bitrus, J.; Amadi, O.; Nwagu, T.; Nnamchi, C.; Moneke, A. Application of Wild Yeast (Saccharomyces cerevisiae) Isolates from Palm Wine and Honey in Baking of Cassava/Wheat Composite Bread. Food And Nut. 2020, 11, 695–711. DOI: 10.4236/fns.2020.117050.
- Rebaz, A. Lactic Acid Bacteria as Bio-Preservatives in Bakery–Role of Sourdough Systems in The Quality, Safety and Shelf Life of Bread, PhD dissertation, University of Plymouth 2017.
- Khalek, M.; Mohamed, T.; Ibrahim, M. Determination of the Staling Rate of Egyptian Balady Bread by Using Texture Profile Analysis (TPA): A New Method. Arab Universities J. Of Agri. Sci. 2019, 27(5), 2527–2538.
- Segun, S.; Adeoye, A.; Oladipo, I.; OLADIPO, I. C. Microbial Assessment and Proximate Composition of Bread Samples Collected from Different Bakeries in Ogbomoso, Oyo State, Nigeria. Notulae Sci. Biol. 2021, 13(1), 10873–10873. DOI: 10.15835/nsb13110873.
- Dashen, M.; Edia-Asuke, U.; Amapu, T.; Adesokan, D.; Olugbodi, F. Effect of Fermented Dough on the Organoleptic Quality and Shelf-Life of Bread. UMYU J Microbiol Res. 2016, 1(1), 104–114. DOI: 10.47430/ujmr.1611.014.
- Zaber, A. Acrylamide Quantitation and Quality Assessment of Locally Available Breads in Chattogram. Chattogram Vet. And Ani. Sci. Uni. Chattogram, Bangladesh. 2022.
- Umeh, S.; Okpalla, J.; Okafor, J. Novel Sources of Saccharomyces Species as Leavening Agent in Bread Making. Int. J. Of Trend In Sci. Res. And Dev. 2019, 3(2), 827–832. DOI: 10.31142/ijtsrd21526.
- Sarah, E.; Yousif, E.; Fattah, A.; Shams, M. Evaluation of Pan Bread Produced by Using Baker’s Yeast Derived from Distilled Biomass. Arab Universities J. Of Agri. Sci. 2018, 26, 1917–1930. DOI: 10.21608/ajs.2018.31660.
- Elsa, B.; Tefera, A.; Muleta, D.; Fantahun, S.; Wessel, G. Molecular Identification and Performance Evaluation of Wild Yeasts from Different Ethiopian Fermented Products. J. Food Sci. Technol. 2020, 57(9), 3436–3444. DOI: 10.1007/s13197-020-04377-7.
- Zotta, T.; Di Renzo, T.; Sorrentino, A.; Reale, A.; Boscaino, F. Selection of Non-Saccharomyces Wine Yeasts for the Production of Leavened Doughs. Microorganisms. 2022, 10(9), 1–13. DOI: 10.3390/microorganisms10091849.
- Li, Z.; Song, K.; Li, H.; Ma, R.; Cui, M. Effect of Mixed Saccharomyces cerevisiae Y10 and Torulaspora Delbrueckii Y22 on Dough Fermentation for Steamed Bread Making. Int. J. Of Food Microbiol. 2019, 303, 58–64. DOI: 10.1016/j.ijfoodmicro.2019.05.009.
- Jelena, D.; Pejin, D.; Popov, S.; Dodic, S.; Mastilović, J.; Puskas, V.; Popov-Raljicc, J. Evaluation of Fermentative Activities of Different Strains of Saccharomyces cerevisiae in Bread Dough. Uni. Novi Sad, Faculty Of Tech., Bul. Cara Lazara. 2011, 34(21000), 1–7.
- Onyimba, I.; Job, M.; Akor, R. Dough Leavening Potentials of Some Indigenous Strains of Saccharomyces cerevisiae Isolated from Fruits. Afr. J. Of Nat. Sci. 2019, 1119–1104.
- Sefinew, T.; Setargie, A.; Ousman, B. Isolation and Characterization of Saccharomyces cerevisiae from Ethiopian Mustard (Brassica carinata). J. Of Academia And Indu. Res. 2019, 8(4), 81–84.
- Tsegaye, Z.; Tefera, G.; Gizaw, B.; Abatenh, E. Characterization of Yeast Species Isolated from Local Fruits Used for Bakery Industrial Application. J. Appl. Microbiol. Res. 2018, 1, 21–26.
- Arjun, G.; Laxmi, A.; Ranjana, P. Preparation and Quality Evaluation of Buckwheat Incorporated Bread. J. Nut. Food Processing. 2021, 4(5), 1–10. DOI: 10.31579/2637-8914/058.
- Mostafa, S.; Rizk, I.; Kishk, Y.; Siham, M. Production and Evaluation of Gluten Free Balady Bread. Curr. Sci. Int. 2020, 9(2), 340–349.
- Mouna, A.; Misbah, A.; Elabed, S.; Haggoud, A.; Mohammed, I.; Koraichi, S. Isolation and Characterization of Potential Starter Yeasts from Traditional Moroccan Sourdoughs. Microbial. Biotechnol. Lett. 2021, 49(4), 502–509.
- Shereen, L.; Hafez, H. Improving the Nutritive Value and Quality Parameters of Gluten Free Bread by Using Natural and Economical Ingredients. Amer. J. Of Food Sci. And Technol. 2020, 8(6), 257–262.
- Syrokou, M.; Paramithiotis, S.; Kanakis, C.; Papadopoulos, G.; Tarantilis, P.; Skandamis, P.; Drosinos, E.; Mataragas, M.; Drosinos, E. H. Effect of Dough-Related Parameters on the Antimold Activity of Wickerhamomyces anomalus Strains and Mold-Free Shelf Life of Bread. Appl. Sci. 2022, 12(9), 1–12. DOI: 10.3390/app12094506.
- Sellitto, V.; Zara, S.; Fracchetti, F.; Capozzi, V.; Nardi, T. Microbial Biocontrol as an Alternative to Synthetic Fungicides: Boundaries Between Pre-And Postharvest Applications on Vegetables and Fruits. Fermentation. 2021, 7(2), 60. DOI: 10.3390/fermentation7020060.
- Roya, A.; Sami, M.; Abedi, R.; Mohammadi, R.; Mirlohi, M. Microbial and Fungal Contamination of Wheat Flour, Dough, and Bread Samples Collected from Isfahan, Iran. Egy. J. Of Vet. Sci. 2020, 51(2), 163–170. DOI: 10.21608/ejvs.2020.19056.1118.
- Sasikala, S.; Madhanisha, S.; Bhuvaneshwari, K.; Kavarshi, K. Microbial Quality Analysis of Locally Packed and Commercially Available Bread in Chennai Region of Tamil Nadu, India. Asian J. Of Food Res. Nut. 2023, 2(1), 1–5.
- Ayoade, R.; Mohammed, S.; Auta, R. Bread Produced from Composite Flours Using Saccharomyces cerevisiae Isolated from Local Fermented Beverage (Burkutu). FUDMA J. Sci. 2020, 4(2), 758–766. DOI: 10.33003/fjs-2020-0402-327.
- Omorodion, N.; Oviwighoyovwe, P. Effect of Storage Conditions on the Microbial and Proximate Composition of Bread Made from Wheat Flour and White Flour. Asian Food Sci. J. 2022, 21(1), 21–30. DOI: 10.9734/afsj/2022/v21i130397.
- Juliana, L.; Bernardi, A.; Morassi, L.; Silva, B.; Copetti, M.; Sant’ana, A. Incidence, Populations and Diversity of Fungi from Raw Materials, Final Products and Air of Processing Environment of Multigrain Whole Meal Bread. Food. Res. Int. 2016, 87, 103–108. DOI: 10.1016/j.foodres.2016.07.002.
- Debonne, E.; Van Bockstaele, F.; De Leyn, I.; Devlieghere, F.; Eeckhout, M. Validation of in-Vitro Antifungal Activity of Thyme Essential Oil on Aspergillus Niger and Penicillium Paneum Through Application in Par-Baked Wheat and Sourdough Bread. LWT. 2018, 87, 368–378. DOI: 10.1016/j.lwt.2017.09.007.
- Soboleva, E.; Sergacheva, E.; Ternovskoy, G. Use of a Probiotic Yeast Strain in Technology of Bread from Wheat Flour. Вес. Ме. хол. 2016, 4(4), 11–15. DOI: 10.21047/1606-4313-2016-15-4-11-15.
- Mehmet, M. Quality Evaluation of Bread Prepared from Wheat–Chufa Tuber Composite Flour. Foods. 2023, 12(3), 1–11. DOI: 10.3390/foods12030444.
- Tadlo, Y.; Moges, D.; Satheesh, N.; Salmerón, I. Effect of Brewery Spent Grain Level and Fermentation Time on the Quality of Bread. Int. J. Of Food Sci. 2022, 2022, 1–10. DOI: 10.1155/2022/8704684.
- Akinyede, A.; Akande, M.; Babatuyi, C.; Oguntuase, S. Storage Effects on Microbiological and Some Antioxidant Potentials of Partially Substituted Bread Produced from Wheat Flour and Fresh Coconut Meat. J. Of Food Technol. 2020, 7(2), 178–188. DOI: 10.18488/journal.58.2020.72.178.188.
- Tamrat, K.; Workineh, A.; Muhaba, S.; Oli, L.; Deribe, M.; Semhal, M.; Lamsgen, Y. Development of Nutritionally Enhanced Wheat Breads Supplemented with Tef (Eragrostis tef (Zucc.) Trotter) Grain Flour. J. Of Food Nut. Sci. 2020, 7, 77–83.
- Timmermans, E.; Langie, I.; Bautil, A.; Brijs, K.; Buve, C.; Van Loey, A.; Courtin, C.; Van der Meulen, R.; Courtin, C. M. Study of the Fermentation Characteristics of Non-Conventional Yeast Strains in Sweet Dough. Foods. 2023, 12(4), 830. DOI: 10.3390/foods12040830.
- Zhou, N.; Schifferdecker, A.; Gamero, A.; Compagno, C.; Boekhout, T.; Piskur, J.; Knecht, W. Kazachstania gamospora and Wickerhamomyces subpelliculosus: Two Alternative Baker’s Yeasts in the Modern Bakery. Int. J. Of Food Microbiol. 2017, 250, 45–58. DOI: 10.1016/j.ijfoodmicro.2017.03.013.
- Akyuz, G.; Maz, B. Physicochemical and Sensory Characterization of Bread Produced from Different Dough Formulations by Kluyveromyces lactis. J. Of Food Processing And Preservation. 2020, 44(6), 1–10. DOI: 10.1111/jfpp.14498.
- Pooja, G.; Sonkar, C.; Masih, D. Effect of Food Additives on the Quality and Storage of Whole Wheat Flat Bread (Chapatti). Pharma. Inn. J. 2021, 10(3), 306–312.
- Katsi, P.; Kosma, I.; Michailidou, S.; Argiriou, A.; Badeka, A.; Kontominas, M. Characterization of Artisanal Spontaneous Sourdough Wheat Bread from Central Greece: Evaluation of Physico-Chemical, Microbiological, and Sensory Properties in Relation to Conventional Yeast Leavened Wheat Bread. Foods. 2021, 10(3), 1–23. DOI: 10.3390/foods10030635.
- Pacher, N.; Burtscher, J.; Johler, S.; Etter, D.; Bender, D.; Fieseler, L.; Domig, K. Ropiness in Bread a Re-Emerging Spoilage Phenomenon. Foods. 2022, 11(19), 3021. DOI: 10.3390/foods11193021.
- Adamczyk, G.; Ivanisova, E.; Kaszuba, J.; Bobel, I.; Khvostenko, K.; Chmiel, M.; Falendysh, N. Quality Assessment of Wheat Bread Incorporating Chia Seeds. Foods. 2021, 10(10), 1–10. DOI: 10.3390/foods10102376.
- Cherinet, K. Physicochemical Techno Functional Properties of Recently Released Ethiopian Bread Wheat Triticum aestivum L Varieties Grown in Kulumsa, Arsi, Ethiopia. PhD dissertation, Addis Ababa University 2020.