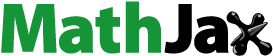
ABSTRACT
In order to find a green and safe solution as the base solution for the quantitative detection of glucose, the neutral solution Na2S2O3 was selected as the base solution. A three-electrode electrochemical system was designed. In this system, the working electrode was copper sheet, the counter electrode was platinum electrode, and the reference electrode was saturated KCl calomel solution electrode. Cyclic voltammetry (CV) and chronoamperometry(i-t) methods were used for the experiment. CV scanning results showed that glucose could produce the oxidation-reduction reaction on the surface of the copper electrode, and the parameters of i-t were adjusted. According to the results of i-t scanning, the quantitative detection model of glucose was established. The model was . The regression parameter R2 was 0.99672. Glucose was tested with different base solutions. Results showed that Na2S2O3 solution was a good base solution. The i-t control experiments showed that the proposed method were effective in Na2S2O3 solution.
Introduction
Sugar plays many important roles in the plant life, one of which is the source of energy to sustain plant life. In the human body, glucose is an important energy and metabolic agent, and the liver is the main source of glucose production.[Citation1–3] Glucose can trigger a neural signal in the brain to achieve the effects of reducing food intake and regulating a high-protein diet.[Citation4] Glenn et al. showed that glucose could treat anxiety disorders.[Citation5] Glucose had also been linked to cognitive impairment. Riby et al. showed that the intake of glucose and the improvement of glucose could memory in the elderly.[Citation6] However, taking so much glucose in that it would cause intestinal inflammation, and the right amount of glucose would promote intestinal regulation. Different concentrations of glucose had different effects on intestinal immunity.[Citation7,Citation8]
A number of methods had been reported for the detection of glucose, including chromatography,[Citation9,Citation10] fluorescence method,[Citation11,Citation12] colorimetry,[Citation13–16] spectrophotometry,[Citation17] electrochemistry[Citation18,Citation19] and others. High performance liquid chromatography (HPLC) had the advantages of high sensitivity and high separation efficiency, but it had the problems of long time consuming, high cost and complicated operations. The fluorescence method was highly sensitive, but it required large instruments and a substantial amount money for a single use. The colorimetric method had the advantages of low cost and practicality, but it had two problems, firstly it relied on enzymes and color developers, secondly the operational was complexity. Spectrophotometry had the advantages of simplicity, rapidity, and no needing for expensive instruments, but it relied on nanoparticles, the synthesis of nanoparticles, and generally requires water-soluble treatment, which was a major challenge. Electrochemical analysis had the advantages of high sensitivity and wide measurement range, but it suffered from the problem of short life of electrode life during the experiments. Since most electrode materials were susceptible to corrosion in acidic environments, most industries choose to use alkaline electrolytes.[Citation20] The oxidation of metal electrodes in alkaline solutions had been extensively studied by electrochemical methods.[Citation21] Although the corrosion of electrodes in acidic solutions was avoided, the destructive effect on electrodes could not be avoided in alkaline solutions. M. Herrmann et al. had shown that sintered Si ceramics have high corrosion resistance, but the electrode surface was corroded after electrochemical testing in NaOH solution.[Citation22] Therefore, in recent years, there had been an increasing focus on alternative solvents that were environmentally friendly, safe and relatively inexpensive.[Citation23] No corrosion in the environment of neutral solution, the stability of working electrode was greatly enhanced, and the life of the electrode could be extended.[Citation24,Citation25] Gisele A.B. Mello et al.[Citation26] studied the oxidative reactivity of glucose on platinum electrode in phosphate buffer solution (PH = 7) and found that Pt has highly activity. Lindsey Goodnight et al. studied a non-enzyme sensor for the detection of glucose in neutral solution and used cyclic voltammetry to determine the oxidation reaction of glucose in Na2SO4 solution.[Citation27] Therefore, it had practical significance to establish a simple, rapid, safe green method for glucose detection.
In this paper, a method for the quantitative detection of glucose solution based on Na2S2O3 solution was studied. A three-electrode electrochemical system was adopted, with the platinum electrode as the counter electrode, the saturated KCl as the reference electrode, the copper sheet as the working electrode and 0.1 mol/L Na2S2O3 solution as the base solution. The experiment used cyclic voltammetry (CV) and chronoamperometry (i-t) methods to quantitatively detect glucose. As the base solution and active agent, Na2S2O3 solution could promote the formation of hydroxide film on the electrode surface, which was conducive to promoting the oxidation reaction of glucose. This method provided a new approach to using a green, safe and neutral solution for the detection of glucose.
Material and method
Reagents and materials
Na2S2O3 was purchased from Sinopharm Chemical Reagent Co., Ltd, Shanghai, China; Glucose was purchased from Guanghua Chemical Reagent Co., Ltd, Guangdong, China; Erythritol was purchased from Sanyuan Biotechnology Co., Ltd, Shandong, China; Psicose was purchased from Juping Technology Co., Ltd, Shandong, China; CHI-614E electrochemical workstation was purchased from Chenhua Instrument, Shanghai, China; Model 85-2A automatic stirrer was purchased from Xinrui Instrument Co., Ltd, Jiangsu; Pipetting gun was purchased from Dalong Xingchuang Experimental Instrument Co., Ltd, Beijing; Copper sheet was purchased from Yucheng Metal Material Co., Ltd, Shanghai; Distilled water.
Reaction mechanism
When Na2S2O3 solution was used as the base solution, OH− produced would be adsorbed on the electrode surface:
OH−→OH*+e−
Copper sheet would produce changes:
Cu→Cu2+→Cu3+
Na2S2O3 was adsorbed on the surface of the copper electrode and oxidized by the copper electrode to form Na2S4O6. Na2S4O6 on the electrode surface reacted with Na2S2O3 in the solution to form Na2S5O6,
S2O32-→S2O3−+e−
2S2O3−→S4O62-
S4O62-+ S2O32-→S5O62-+SO32-
When the glucose solution was added, the glucose was oxidized:
ROH+R’CHO+Cu3++e−→R’COOR+Cu2++H2O
CV experiment
CV experiment was carried out at room temperature of 25°C, the working electrode was copper sheet, the base solution was 0.1 mol/L Na2S2O3 solution, the solution to be measured was 0.1 mol/L glucose solution, and the average scanning rate was 0.05 V/s.
I-t experiment
The quantitative detection of glucose mainly adopted i-t experiment, using 0.1 mol/L Na2S2O3 solution as electrolytic substrate, copper sheet as working electrode and the contact area with solution was 0.25 × 2 cm2. The i-t experiment was conducted under the condition of 25°C room temperature, +0.1 V potential, and constant temperature automatic mixer in continuous operation. The total detection time was 1200s. Glucose solution was added to the base solution from 200s, and quantitative detection started. During the detection, 50 μL of 0.1 mol/L glucose solution was added from the automatic syringe every 50s. A total of 20 drops were added in each experiment (including the glucose solution at 200 seconds).
Control experiment
Distilled water, 0.1 mol/L NaOH solution and 0.1 mol/L Na2S2O3 solution were used as base solutions to perform i-t experiments on 0.1 mol/L glucose, and compared the results. Using Na2S2O3 solution as the base solution, 0.1 mol/L erythritol and 0.1 mol/L psicose were scanned respectively and compared the i-t data obtained.
Results and discussion
CV scanning
Using Na2S2O3 as the base solution, the copper sheet of the working electrode was pre-treated first. Under the continuous scanning of the working electrode, the effect of the working electrode was more obvious and stable. As shown in , 300 rounds of continuous scanning were performed at a scanning speed of 0.05 V/s. The positive potential was the oxidation peak, while the opposite potential was the reduction peak. After 120 rounds of scanning, it was obviously indicated that working electrode was in a stable state.
CV experiments with different scanning rates
In the CV experiment, 20 mL of 0.1 mol/L Na2S2O3 was taken as the base solution for the no-load experiment, and 100 μL of distilled water was added to the base solution, which was shown in . Then 20 mL of 0.1 mol/L Na2S2O3 was taken as the base solution, and 100 μL of 0.1 mol/L glucose was added to the base solution, which was shown in . The scanning rates of the two CV experiments were 0.02 V/s, 0.03 V/s, 0.04 V/s, 0.05 V/s, 0.06 V/s, 0.07 V/s, 0.08 V/s, 0.09 V/s and 0.10 V/s, respectively, and the voltage scanning ranged from −1 V to 0.4 V.
The data of the CV experiment data without load was subtracted from the CV experiment data with glucose (subtracted according to the scanning rate). shows the CV diagram after subtraction, which indicated that the current value of the oxidation peak was relatively stable. At the same time, the redox peak of Na2S2O3 containing glucose was smaller than that of unloaded Na2S2O3 (see ), indicating that the copper electrode had a strong ability to oxidize Na2S2O3 solution as a catalyst.
I-t experiment
To ensure that the results of the experiment were stable, repeatable and accurate, i-t data were recorded for 10 groups for the determination of distilled water and 10 groups for the determination of glucose. The voltage was set to +0.1 V. Firstly, 20 mL 0.1 mol/L Na2S2O3 was taken as the base solution for the no-load experiment. 50 μL distilled water was added every 50s at the beginning of 200s. The i-t diagram of water addition was shown in . Then 20 mL 0.1 mol/L Na2S2O3 was taken as the base solution for quantitative detection of glucose solution. Starting from 200s, 50 μL 0.1 mol/L glucose solution was added every 50s. The i-t diagram of the glucose solution was measured as which was shown in .
Figure 2. I-t tests: (a) I-t with distilled water; (b) I-t with glucose solution; (c) Comparison of no-load and measured glucose solution current response signals; (d) Difference diagram of current response between no-load and measured glucose solution; (e) Non-linear fitting of glucose concentration and current density.

The collected distilled water i-t data and glucose i-t data were averaged separately. The comparison of the i-t data in two different states was shown in . It showed that the current value of the distilled water addition was smaller than that of the glucose addition. In the i-t experiment with the addition of distilled water, Na2S2O3 as a basic solution would be adsorbed on the surface of the copper electrode and oxidized to S5O62-. Due to the reduction of Cu3+, the oxidation capacity of the copper electrode became weak, so the current response signal showed an upward trend. After the addition of glucose solution, it could also be oxidized under the catalysis of the copper electrode. However, it was not as strong as in pure Na2S2O3 solution. The addition of glucose inhibited the oxidation of Na2S2O3. In addition, it was observed that the current response curve of distilled water tended to rise smoothly, while the current response curve of glucose was stepped every 50s. The response of the two states was completely different.
Then formula 1 was used to calculate the current difference between glucose and distilled water, where m was the difference, m1 was glucose i-t data and m2 was the distilled water i-t data.
As shown in , the i-t data difference between glucose and distilled water was negative. It was shown that the current intensity of glucose was greater than that of distilled water. Therefore, in the same solution environment, the current signal of two substances being oxidized was greater than that of a single substance. When the liquid to be tested was added at 200s, the current value did not change much and showed a downward trend after 60s (see . The difference peaked at around 300s and then showed an upward trend. The difference value of the last 1000s of the i-t experiment was a stepped shape (see , which represents the reaction after adding glucose solution every 50s. According to this phenomenon, the glucose solution could be detected quantitatively.
Table 1. The difference of current response at 1000s between no-load and glucose solution.
I-t nonlinear fitting
The average value of the current response signal was taken every 50s and then divided by the contact area between the electrode and the solution to obtain the current density. According to formula 2, the glucose concentration in the solution per 50s could be calculated. Where CN was the concentration after the addition of the glucose solution, N (1,2,……,20) was the number of times the glucose solution was added(20 drops in total). C0 was the initial concentration of the glucose solution.
LogNormal nonlinear function was used for fitting (see , the R2 of which was 0.99672. The processed i-t data were suitable for nonlinear fitting and the error was small, so the quantitative detection of glucose would be more accurate. Formula 3 was used to obtain the current density to glucose concentration formula, where y was the current density and x was the glucose concentration:
The formula of glucose concentration to current density was derived from formula 3, as shown in formula 4:
Control experiment
In control experiment 1, 0.1 mol/L Na2S2O3 solution, distilled water and 0.1 mol/L NaOH solution were each used as the base solution to perform quantitative detection of 0.1 mol/L glucose solution and these three types of experiments were compared (see . The results indicated that when distilled water was used as the base solution, the change in the current response signal was very small. The reason was that the OH− electrolyzed out of the water became less. And the Cu3+ produced on the surface of copper was also became less under the action of OH−. So the ability to oxidize glucose was weak. When NaOH solution was used as the base solution, more OH− could be electrolyzed in the electrolytic tank than distilled water, and the Cu3+ produced on the surface of copper was also relatively more, so its ability to oxidize glucose was stronger than distilled water, and the current response signal was the largest. But as an alkaline solution, if the experiment was not done properly, it would be harmful to the human body. When Na2S2O3 solution was used, its oxidation capacity was gradually weakened, and the current response curve was significantly different from that of distilled water and NaOH. However, compared with distilled water, it could quantitatively detect glucose solution by the obvious change in current. Compared with NaOH solution, Na2S2O3 solution (commonly known as baking soda) was less harmful to the human body than NaOH solution, although the current response signal of NaOH solution for quantitative detection of glucose was stable and obvious.
Figure 3. Control tests: (a) Controlled experiments with different electrolytes; (b) Controlled experiments with different sugar solutions.

In control experiment 2, to ensure the repeatability and stability of the experiment, 10 groups of i-t experiments were carried out on 0.1 mol/L psicose and 0.1 mol/L sucrose respectively. They were averaged to obtain the mean value and finally to obtain the i-t experimental data of two groups of different sugar solutions. The difference value was obtained by subtracting the i-t data of distilled water from the i-t data of the two sets. showed the difference diagram of the three sugar solutions. The intensity of the current responsed signal after 200s was observed (there was no test solution in the base solution before 200s, the addition of test solution was started at 200s, and then 50 μL test solution was added every 50s) and compared with glucose. The results of the i-t experiment showed that the electrochemical response signal strength of different sugars was different. Psicose was a kind of ketose, which was weak in oxidization. Because of the whole i-t oxidation process, the current response of psicose was larger than that of glucose. Erythritol was a commonly used food sweetener. As shown in , with the addition of erythritol, the ability of the electrode to oxidize Na2S2O3 was decreasing. Due to the antioxidant properties of erythritol, the difference of the erythritol current response signal decreased and increased rapidly from 200s to 466s, and became positive for the second 466s (see ). The experimental results indicated that Na2S2O3 solution could be used to discriminate different sugar solutions.
Table 2. Difference of i-t data of different sugar solutions to be tested after 200s.
Conclusion
The glucose non-enzymatic electrochemical quantification method in a neutral solution environment was studied. Na2S2O3 solution was used as the base solution. An electrochemical system with three electrodes was designed. The working electrode was copper sheet, the counter electrode was platinum electrode, and the reference electrode was saturated KCl calomel. CV and i-t methods were used to scan the glucose solution. Continuous CV scanning experiments showed that the copper sheet had good repeatability and stability after 120 rounds of scanning. The voltage of the i-t experiment was parameterized based on the results of the CV experiments at different scanning speeds. I-t experiments were best performed at +0.1 V. According to the collected data, a glucose quantitative detection model was established. The model was .The regression parameter R2 was 0.99672. Therefore, a non-linear regression prediction model was suitable for the prediction of glucose concentration in Na2S2O3 solution environment. Compared with distilled water, Na2S2O3 solution had a better effect. Because when Na2S2O3 solution was used as the base solution, it could improve the formation of hydroxide film on the electrode surface, promoting the oxidation reaction of glucose and increasing the current value of glucose solution detection. Compared to NaOH solution, the i-t current curve of Na2S2O3 solution was also obvious. According to the i-t comparison experiment of different sugars, Na2S2O3 solution could be used to distinguish between different sugars solutions. Based on the above results it was shown that Na2S2O3 solution could be used for quantitative detection of sugar solution. It provided an idea for using neutral solution as electrolytic substrate to ensure environmental protection and safety of the experiment.
Authors contribution
Jingyu Zhang: Methodology, Investigation, Visualization, Writing – original draft. Yaping Xie: Validation, Formal analysis, Investigation, Review and editing. Wenhui Han: Data collection, Validation, Visualization, Formal analysis. Guohua Hui: conceptualization, resources, funding acquisition, review and editing, visualization, supervision. Yue Zheng: Validation, Visualization, Formal analysis, Investigation. Qiansheng Ye: Validation, Formal analysis, Investigation. Yuxuan Xu: Visualization, Formal analysis, Investigation. Xinnan Chen: Visualization, Formal analysis, Investigation. Qi Lv: Formal analysis, Investigation, Resources, Review and editing. Chengxiang Yong: Formal analysis, Investigation. Yongzhi Lai: Formal analysis, Investigation. Xinao Shen: Formal analysis, Investigation. Peng Wu: conceptualization, resources, funding acquisition, review and editing, visualization, supervision, project administration.
Ethical approval
This article does not contain any studies with human participants or animals performed by any of the authors.
Acknowledgments
The authors acknowledge programmers who contributed to the instrumentation and devices. Additionally, thanks to the people who fabricated the experimental materials.
Disclosure statement
No potential conflict of interest was reported by the author(s).
Data availability statement
Data sharing does not apply to this article, as no datasets were generated or analyzed during the current study.
Additional information
Funding
References
- Chen, M.; Zhang, Y.; Zeng, S.; Li, D., You, M., Zhang, M., Wang, Z., Wei, L., Chen, Y., Ruan, Z. CD36 Regulates Diurnal Glucose Metabolism and Hepatic Clock to Maintain Glucose Homeostasis in Mice. ISCIENCE. 2023, 26(4), 106524. DOI: 10.1016/j.isci.2023.106524.
- Macaya, D. J.; Nikolou, M.; Takamatsu, S.; Mabeck, J. T.; Owens, R. M.; Malliaras, G. G. Simple Glucose Sensors with Micromolar Sensitivity Based on Organic Electrochemical Transistors. Sens. Actuators B Chem. 2007, 123(1), 374–378. DOI: 10.1016/j.snb.2006.08.038.
- Muaz, M. Z. M.; Mashitah, M. D. Electricity Generation from Sewerage Sludge in Microbial Fuel Cell, Considering the Effect of Glucose. Appl. Mech. Mater. 2015, 793, 393–397. DOI: 10.4028/www.scientific.net/amm.793.393.
- Delaere, F.; Akaoka, H.; De Vadder, F.; Duchampt, A.; Mithieux, G.; Lyon, D. Portal Glucose Influences the Sensory, Cortical and Reward Systems in Rats. European J. Neurosci. 2013, 38(10), 3476–3486. DOI: 10.1111/ejn.12354.
- Luyten, L.; Schroyens, N.; Luyck, K.; Fanselow, M. S.; Beckers, T. No Effect of Glucose Administration in a Novel Contextual Fear Generalization Protocol in Rats. Transl. Psychiatry. 2016, 6(9), e903–e903. DOI: 10.1038/tp.2016.183.
- Riby, L. M.; Marriott, A.; Bullock, R.; Hancock, J.; Smallwood, J.; McLaughlin, J. The Effects of Glucose Ingestion and Glucose Regulation on Memory Performance in Older Adults with Mild Cognitive Impairment. Eur. J. Clin. Nutr. 2009, 63(4), 566–571. DOI: 10.1038/sj.ejcn.1602981.
- Yu, Y.; Yang, W.; Yu, T.; Zhao, X.; Zhou, Z.; Yu, Y.; Xiong, L.; Yang, H.; Bilotta, A. J.; Yao, S., et al. Glucose promotes regulatory T cell differentiation to maintain intestinal homeostasis. iScience. 2022, 25(9), 105004.
- Sitter, T.; Sauter, M. IN-DEPTH REVIEW. Peritoneal Dialysis International: Journal of the International Society for Peritoneal Dialysis. 2005, 25(5), 415–425. DOI: 10.1177/089686080502500502.
- Taulor, R. B.; Sooo, V. C. An h.p.l.c. study of the initial stages of dextrose decomposition in neutral solution. Journal of Pharmacy and Pharmacology. 2011, 30(1), 510–511. DOI: 10.1111/J.2042-7158.1978.TB13305.X
- Soyseven, M.; Sezgin, B.; Arli, G. A Novel, Rapid and Robust HPLC-ELSD Method for Simultaneous Determination of Fructose, Glucose and Sucrose in Various Food Samples: Method Development and Validation. J. Food Compos. Anal. 2022, 107(September 2021), 104400. DOI: 10.1016/j.jfca.2022.104400.
- Zhou, S.; Min, X.; Dou, H.; Sun, K.; Chen, C.-Y.; Chen, C.-T.; Zhang, Z.; Jin, Y.; Shen, Z. Facile fabrication of dextran-based fluorescent nanogels as potential glucose sensors. Chem. Commun. 2013, 49(82), 9473–9475. DOI: 10.1039/c3cc45668d.
- Pushina, M.; Penavic, A.; Farshbaf, S.; Anzenbacher, P. Fluorescent Sensor Array for Quantitative Determination of Saccharides. ACS Sens. 2021, 6(11), 4001–4008. DOI: 10.1021/acssensors.1c01371.
- Xu, B.; Xiang, X.; Ding, Z.; Luo, Z.; Huang, J. A Colorimetric Assay for Detection of Glucose by Enzymatic Etching of Triangular Gold Nanosheets. Mater. Chem. Phys. 2023, 301(March), 127619. DOI: 10.1016/j.matchemphys.2023.127619.
- Cai, T.; Gao, Y.; Yan, J.; Wu, Y.; Di, J. Visual Detection of Glucose Using Triangular Silver Nanoplates and Gold Nanoparticles. R.S.C. Adv. 2017, 7(46), 29122–29128. DOI: 10.1039/c7ra00593h.
- Zhang, X.; Sucre-Rosales, E.; Byram, A.; Hernandez, F. E.; Chen, G. Ultrasensitive Visual Detection of Glucose in Urine Based on the Iodide-Promoted Etching of Gold Bipyramids. ACS Appl. Mater. Interfaces. 2020, 12(44), 49502–49509. DOI: 10.1021/acsami.0c16369.
- Radhakumary, C.; Sreenivasan, K. Naked Eye Detection of Glucose in Urine Using Glucose Oxidase Immobilized Gold Nanoparticles. Anal. Chem. 2011, 83(7), 2829–2833. DOI: 10.1021/ac1032879.
- Ma, Y.; He, W.; Fu, X.; Gao, X. Detection of Glucose by Copper Ion Catalytic ABTS-H2O2 Spectrophotometry. J. Phys.: Conf. Ser. 2020, 1676(1), 012126. DOI: 10.1088/1742-6596/1676/1/012126.
- Ahmad, R.; Khan, M.; Mishra, P.; Jahan, N.; Ahsan, M. A.; Ahmad, I.; Khan, M. R.; Watanabe, Y.; Syed, M. A.; Furukawa, H., et al. Engineered Hierarchical CuO Nanoleaves Based Electrochemical Nonenzymatic Biosensor for Glucose Detection. J. Electrochem. Soc. 2021, 168(1), 017501.
- Li, H.; Guo, C. Y.; Xu, C. L. A Highly Sensitive Non-Enzymatic Glucose Sensor Based on Bimetallic Cu-Ag Superstructures. Biosens. Bioelectron. 2015, 63, 339–346. DOI: 10.1016/j.bios.2014.07.061.
- Lin, T. W.; Liu, C. J.; Dai, C. S. Ni3S2/carbon nanotube nanocomposite as electrode material for hydrogen evolution reaction in alkaline electrolyte and enzyme-free glucose detection. Appl. Catal. B. 2014, 154–155, 213–220. DOI: 10.1016/j.apcatb.2014.02.017.
- Shi, Q.; Diao, G.; Mu, S. Electrochemical Oxidation of Glucose on Gold Nanoparticle-Modified Reduced Graphene Oxide Electrodes in Alkaline Solutions. Funct. Mater. Lett. 2015, 8(3), 8–11. DOI: 10.1142/S1793604715400044.
- Herrmann, M.; Sempf, K.; Wendrock, H.; Schneider, M.; Kremmer, K.; Michaelis, A. Electrochemical Corrosion of Silicon Carbide Ceramics in Sodium Hydroxide. J. Eur. Ceram. Soc. 2014, 34(7), 1687–1693. DOI: 10.1016/j.jeurceramsoc.2013.12.043.
- Yang, Y.; Gao, W.; Xu, B.; Li, Q.; Jiang, T. Study on Oxygen Pressure Thiosulfate Leaching of Gold without the Catalysis of Copper and Ammonia. Hydrometallurgy. 2019, 187(September 2018), 71–80. DOI: 10.1016/j.hydromet.2019.05.006.
- Murthy, A. P.; Theerthagiri, J.; Madhavan, J.; Murugan, K. Enhancement of Hydrogen Evolution Activities of Low-Cost Transition Metal Electrocatalysts in Near-Neutral Strongly Buffered Aerobic Media. Electrochem. Commun. 2017, 83, 6–10. DOI: 10.1016/j.elecom.2017.08.011.
- Wang, Z.; Lin, Z.; Diao, P. Hybrids of Iridium-Cobalt Phosphates as a Highly Efficient Electrocatalyst for the Oxygen Evolution Reaction in Neutral Solution. Chem. Commun. 2019, 55(20), 3000–3003. DOI: 10.1039/c8cc10278c.
- Mello, G. A. B.; Cheuquepán, W.; Feliu, J. M. Investigation of Reactivity of Pt Basal Planes Towards Glucose Electro-Oxidation in Neutral Solution (pH 7): Structure-Sensitivity Dependence and Mechanistic Study. J. Electroanal. Chem. 2020, 878, 114549. DOI: 10.1016/j.jelechem.2020.114549.
- Goodnight, L.; Butler, D.; Xia, T.; Ebrahimi, A. Non-Enzymatic Detection of Glucose in Neutral Solution Using PBS-Treated Electrodeposited Copper-Nickel Electrodes. Biosensors. 2021, 11(11), 1–12. DOI: 10.3390/bios11110409.