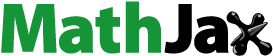
ABSTRACT
Reducing ice cream fat content without losing its quality characteristics is a nutritional requirement of many consumers and it is a challenge for the ice cream industry. The objective of this study was to evaluate low-fat hard ice cream formulations using modified cassava starch as a fat substitute. It was used in a proportion of 10% and hydrocolloids such as carboxymethyl cellulose (CMC), guar gum, and xanthan gum were used as stabilizers. Mixture viscosity, drip-through time, melting percentage, total solids, titratable acidity, pH, and hardness were determined. The best formulations were the treatments with a mixture of 67% CMC, 33% guar gum, and 100% xanthan gum; they had a viscosity above 5000 cP, melting percentage lower than 7%, and overrun higher than 93%. In addition, a sensory acceptance evaluation was performed on the two best treatments and compared to a low-fat ice cream using a commercial stabilizer and an ice cream with a 7% fat ratio. The sensory results showed that the samples did not present significant differences, which is important because the best formulations can compete with the commercial stabilizers currently used for this type of ice cream.
Introduction
People are increasingly choosing low-fat foods and beverages, as well as new foods with this feature, because reducing dietary fat is one of the main nutritional goals of many consumers.[Citation1] The use of fat replacers in the food industry becomes important because people require foods that have the right sensory characteristics and that also help prevent diseases. For instance, the consumption of saturated fats is related to the development of cardiovascular and metabolic diseases.[Citation2] However, removing fats from food products can negatively affect their overall quality such as viscosity, texture, appearance, and taste.[Citation3]
From a physical point of view, ice cream is a very complex food as it constitutes a four-phase food system (emulsion, gel, suspension, and foam). It is a partially frozen foam that contains between 40 and 50% air (v/v) and its components (water, carbohydrates, lipids, proteins, and minerals) play a fundamental role in the elaboration, conservation, and final texture of the product.[Citation4]
Normally, ice cream contains 10–16% fat from dairy or nondairy sources. Fat is an important component because it affects its dryness, preservation of its initial shape after freezing, resistance to melting, and softness after hardening. Therefore, eliminating or reducing its fat content leads to many defects in the final quality of the product.[Citation2]
Modified starches have been widely used as fat replacements in ice cream. Surendra Babu et al.[Citation5] used modified sweet potato starch treated with citric acid as a fat replacement in ice creams and reported that reduced-fat ice creams were very acceptable to sensory panelists with physicochemical characteristics and acceptance similar to those with a normal fat content. Surapat and Rugthavon[Citation6] used modified starch (α-Starch or MT-01) as a fat substitute in coconut milk ice cream – a milky white opaque liquid extracted from the grated pulp of ripe coconuts. The amount of fat in the coconut milk was reduced to different levels (1–8%), then, the fat substitute was added. The results showed that the reduction of the fat content and the increase of the starchy substitute tended to increase the viscosity, overrun, and deformation force of the ice cream, but did not have a significant effect on the melting rate and pH, i.e., the results did not vary with respect to the control sample.
The viscosity, texture, drip-through time, and melting percentage are characteristics or parameters that determine the quality of a good ice cream. Incorporating maltodextrin (0, 1, and 2% w/w) and resistant starch (0, 1 and 2% w/w) into the low-fat ice cream formulation (4% fat) resulted in ice cream with an increase in an overrun of 45% for concentrations of maltodextrin and resistant starch higher than 1.5%, and viscosity showed a significant increase from 748 cP to 1264 cP for high concentrations of maltodextrin and resistant starch.[Citation7] When incorporating modified starch, whey protein concentrate, and inulin to soft low-fat ice creams, there was a decrease in general acceptance compared to the control sample with all fat content, especially in the treatments containing whey protein concentrate and modified starch.[Citation8]
Despite the aforementioned studies, there are few on the application of modified cassava starch and hydrocolloids in the manufacture of low-fat hard ice cream. Therefore, the purpose of this study was to use modified cassava starch and evaluate different concentrations of CMC, xanthan gum, and guar gum as stabilizers in the physicochemical and sensory properties of low-fat ice creams and compare the most suitable formulations with a commercial ice cream premix at the sensory level.
Materials and methods
Raw materials
The following ingredients were used to make the ice cream: Whole milk powder (Adecoagro, Buenos Aires, Argentina), according to the typical composition shown on the label, with 26% fat, 4% moisture, and 70% SNF (solids-nonfat); margarine (Astra, CI Sigra S.A., Bogotá, Colombia) with 77% fat; cane sugar (Riopaila Castilla, Pradera, Colombia); soy lecithin (E-322, Colorisa, Sabaneta, Colombia); carrageenan (Ceamgel M 9422, Compañía Española de Algas Marinas, S.A., Porriño, Spain); CMC (Gelycel F1 1500, Amtex Colombia, Medellín, Colombia); guar gum (Guangrao Liuhe Chemical Co., LTD. Shandong, China); xanthan gum (Foodchem International Corporation, Shanghai, China); cross-linked cassava starch (Gel®Lact XP, Poltec, La Estrella, Colombia); and Gelatin 280° Bloom (Colorisa, Sabaneta, Colombia)
Formulation
The formulation used to make the low-fat ice cream was determined by preliminary tests. For 100 kg of ice cream mix, the composition was 12% fat, 14.5% sugar, 0.3% stabilizer and emulsifier in a 3:1 ratio, respectively, and 10.6% milk solids not fat. The stabilizer contained hydrocolloid (CMC, guar gum, and xanthan gum) and gelatin in a 4:1 ratio, respectively, while the emulsifiers were composed of carrageenan and soy lecithin in a 1:1 ratio, respectively. Each 30 kg run underwent a fat replacement of 10% cross-linked cassava starch. A Simplex-Latex design was created to compare the effects of the three hydrocolloids in 11 treatments, whose order was completely random. In the formulation, the proportion of hydrocolloids varied () and the other ingredients remained constant.
Table 1. Percentage share of hydrocolloids.
Ice cream preparation
The raw materials were weighed, and all solid ingredients and powders were mixed. During melting and heating, a double-jacketed automatic kettle (Servinox, Rionegro, Antioquia, Colombia) was used at a temperature between 55–60°C to heat the water and melt the margarine. The dried material was mixed with the liquid phase using a turbomix (CA2618, Carpigiani, Bologna, Italy). The mixture was fed to the homogenizer (3DD13–2941, Regis Chicago USA) at 1500 psi. It was then pasteurized at a temperature of 72°C for 30 min. The resulting mixture went to aging for hydration of the components at a temperature of 4–6°C for 24 h. Finally, it was taken to the machine to whip the ice cream for 16–20 min, stored in 3 kg containers, and kept at −25°C and −30°C for hardening. The samples were taken before and after whipping for their respective analysis.
Chemical-physical analysis
Titratable acidity was expressed as lactic acid percent (AOAC, 947.05), pH was determined with a HANNA instruments potentiometer (AOAC 981.12), and soluble solids were determined by refractometer (AOAC 925.10).[Citation9]
Viscosity
The methodology mentioned by Surapat and Rugthavon[Citation6] was used, with some modifications. The temperatures of the samples ranged between 4°C and 6°C, and a Brookfield DV-III ULTRA digital rheometer was used. When setting the equipment up, a reading of 20 data every 10 seconds was set until reaching the speed of 250 rpm. The characteristics of each sample were considered to select the equipment spindle; it was necessary to choose the appropriate accessory to measure the viscosity of each treatment. In total, 3 different spindles were used: RV4, RV5, and RV6. For the analysis, the maximum viscosity obtained at a speed of 13.16 rpm was taken as representative data.
Overrun
The increase of the ice cream volume during the cold whip is known as overrun, this increase refers to the volume of the mixture that enters the machine before being whipped.[Citation10] To measure the overrun we used plastic containers with a defined volume. After freezing the product in an ice cream maker, a sample of the produced mixture was taken, then it was weighed and overrun was calculated through by EquationEquation (1)(1)
(1) ).[Citation7]
Melting rate and drip-through time
Slow melting, maintaining good shape, and slower decomposition are important qualitative parameters that describe the quality of a good ice cream.[Citation11] A 2.5 mm mesh screen was used to measure this parameter. The samples were molded with an approximate weight of 60 g, then they were placed in plastic containers previously prepared with the mesh screen and duly labeled. The analysis was made for 1 h at room temperature (20°C) and the weight of the molten liquid was recorded based on the percentage of the initial weight, which was set as the melting rate. The drip-through time was also recorded during melting.[Citation12]
Hardness
It was determined with a texture analyzer (TA-XT2i, Stable Micro Systems, Godalming, U.K.) with a 50 kg load cell and using a 2 mm stainless steel cylindrical probe (P/2). The conditions of the analysis were as follows: a distance of 15 mm, probe speed during penetration was equal to 3.3 mm/s, and probe speed before and after penetration was 3.0 mm/s.[Citation5]
Sensory evaluation
The sensory properties of the products were evaluated by a consumer acceptance test with 100 panelists, 51 women and 49 men between 18 and 60 years of age. They rated the texture, taste, and general acceptance by an unstructured qualitative hedonic scale of 10 cm, where 0 = Dislike extremely and 10 = Like extremely.[Citation13] For this test, 2 treatments that obtained better quality results in the experimentation were selected, a commercial mix (Tecnas, Itagui, Antioquia, Colombia) and a 7% fat-reduced sample using one of the previously chosen best treatments.
Statistical design
Once the analyses of each treatment were made, a statistical test (desirability index) was conducted by R software (RS Team – Inc., Boston, MA, 2015). The most representative analyses we considered to choose the best formulations were viscosity, texture, melting percentage, drip-through time, and overrun ().
Table 2. Parameters for statistical analysis.
It was determined that the variables to be maximized were overrun, viscosity, and drip-through time; and the variables to be minimized were melting percentage and texture. In addition, ranges were established to add more detail to the qualification and be more rigorous in the selection of the best formulation, namely, d1 (desirability 1) and d0 (desirability 0). These were defined according to preliminary experiments (). From the values recorded in the experiment, the function takes default values d0 and d1 for each variable: d0 refers to the lowest desirability, while d1 refers to the highest desirability. For the maximized variables, the maximum response observed in the test is taken as d1, and the minimum as d0. On the contrary, for the minimized variables, the minimum response observed in the test is taken as d1, and the maximum response as d0. Regarding the overrun, values between 50% and 90% were established for d0 and d1, respectively, considering the qualification of a standard ice cream.[Citation14]
In the sensory evaluation, a statistical analysis was performed using a non-parametric Friedman and Holm test in R to determine the acceptance of the four selected treatments (multiple comparisons). The rating comprised the sum of the ranks of each category (taste, texture, and general acceptance) in a range scale from 1 to 4; 1 being the unpleasant end and 4 being the pleasant end.
Results and discussion
Physicochemical properties
These analyses were only taken into account to establish quality parameters (). Pandiyan et al[Citation15] reported an acidity (AT) for ice cream between 0.15 and 0.21% lactic acid. In turn, Surendra Babu et al[Citation5] report pH of 6.6–6.7 at 25°C. In traditional ice creams made with milk, the soluble solids (SS) range from 28 to 40%.[Citation16] The results obtained showed that the addition of cassava starch and hydrocolloids had no significant effect on the pH, SS, and AT values.
Table 3. Physicochemical properties of ice cream.
Viscosity
Viscosity is defined as the resistance to flow, and the higher its quantity, the more force it takes to freeze and aerate the ice cream. Water retention capacity, the degree of polymerization and branching are among the most critical factors influencing viscosity development in ice cream mixtures.[Citation17] The increase in viscosity could inhibit the development of coldness in frozen dairy desserts, due to the concentration of the thawed phase in the sheets, which could increase sufficiently to decrease the movement of water molecules and thus limit the formation of ice crystals.[Citation18] The viscosity of the ice cream mixture depends on the shear rate (pseudoplastic), the type and concentration of the stabilizer used, the solids and protein content of the mixture, the incorporation way of the stabilizer into the solution, the temperature at which this incorporation occurs, and the time at which the stabilizer is brought into contact with the mixture.[Citation19]
According to the analysis results, the treatments whose formulation contained 33% CMC-33% guar gum-33% xanthan gum; 67% CMC-33% xanthan gum; 100% xanthan gum; 100% CMC; 67% CMC-33% guar gum and 100% guar gum showed a significant effect on the viscosity of the ice cream mixture. However, we cannot infer that the variation of the hydrocolloids led to the increase in viscosity because the treatments whose mixtures contained only one hydrocolloid also showed good results, with viscosities above 5000 cP (). Adding modified cassava starch and hydrocolloids can increase the functional properties of the ice cream, such as water retention capacity, which can cause an increase in viscosity. Similar results were reported when adding resistant starch as a fat substitute, thus increasing the product viscosity from 748 cP to 1264 cP.[Citation7] Moreover, Zhao et al.[Citation20] reported that the addition of hydrocolloid mixtures tends to increase the rheological and sensory properties of low-fat ice cream. In general, the increase in viscosity may be related to the reaction of starch-hydrocolloids with the liquid part of the mixture and the high-water absorption capacity of these compounds.[Citation21] Incorporating hydrocolloids and modified starch can generate more swelling power and an increase in the viscosity of the cold paste, consequently increasing the viscosity of the ice cream mixture.[Citation8]
Overrun
The amount of air in the ice cream is very important due to its effect on its quality and profitability. As a result, it is necessary to consider the national standards NTC-1239 to achieve adequate overrun.[Citation14] Overrun depends on several factors, including the type of blend components such as fat content, solids, sweeteners, and the presence of stabilizing materials.[Citation11] The results obtained in both the overrun and the viscosity () show that these two variables have a proportional behavior, this means that in mixtures with a value greater than 5000 cP, the overrun is expected to be greater than 70%. However, it cannot be inferred that the higher the viscosity, the greater the overrun. The results showed that the treatments whose variation of hydrocolloids had 67% CMC-33% xanthan gum; 100% xanthan gum, and 67% CMC-33% guar gum, presented 96%, 100%, and 117.4% overrun, respectively. It is important to note that Colombian legislation sets a maximum overrun of 100% for ice cream.[Citation14] Similar studies show that the use of modified starch as a fat substitute in ice cream formulations leads to increased overrun.[Citation6]
The increase in the mixture viscosity resulting from the addition of polysaccharides does not always contribute to an increase in overrun, as samples with rigid polysaccharides show an overrun similar to that of the low-fat reference without polysaccharides. Instead, polysaccharide structure seems to play an important role in determining the development of overrun. Ice cream blends with flexible polysaccharides show a greater ability to incorporate air due to their low shear sensitivity and molecular flexibility. Rigid polysaccharides provide more robust behavior and tend to form a gel network in the whey phase, which is related to lower air uptake.[Citation22] Air cell structure is one of the most influential factors in melting rate, shape retention during melting, and rheological properties in ice cream melting, which correlate with creaminess. Smaller air cells improve product quality with respect to those three indicators. Changes in the size of the air cell could be directly attributed to changes in the rheological properties of the ice cream during freezing, at the beginning of this process, the apparent viscosity increases and leads to a reduction in the maximum size of the air cells due to the increase in the shear stress applied to break them.[Citation11]
Melting rate
There is an indirect relationship between the melting rate and the thermal diffusivity property in ice cream, so any factor that affects thermal diffusivity can influence the melting rate. Dairy fat or vegetable oils reduce the thermal diffusivity of ice cream so that the fat can act as an insulator against heat. In addition, the fat stabilizes the air cells in the ice cream structure with its partially fused fat environment, thus resulting in better shape retention during storage and consumption. Therefore, low-fat ice creams have weaker melting properties compared to standard ice creams.[Citation2]
Fat reduction does not prevent obtaining suitable results (). In some cases, the samples did not have a drip-through time, this could be caused by the different elements involved in the treatment, such as starch and hydrocolloids because they have the ability to bind water to their structure and it is not free within the product.[Citation20] Regarding the melted sample, in most cases, it did not fall into the arranged containers; it was trapped in the mesh. Some of the samples at the end of the analysis kept the shape of the mold, that is, they did not deform entirely ().
The highly viscous whey phase created by the addition of polysaccharides can contribute to a slower melting rate. As viscosity increased, flow resistance increased, which slowed the dripping rate through the mesh.[Citation22,Citation23] If the product melts too quickly, a complicated situation can occur. A fast-melting product is undesirable also because it tends to receive heat stroke easily. However, a very slow rate of melting can also indicate a faulty ice cream.[Citation11]
Hardness
The results show significant variations between each treatment (). It is observed that the treatments with better textures contained 67% CMC-33% guar gum; 100% CMC and 100% xanthan gum. Reducing the fat content in ice cream formulations affects hardness and other quality attributes in the finished product. Ice cream hardness is inversely related to fat content because fat reduction increases ice crystals and probably makes ice cream texture harder, so the hardness of low-fat ice cream is expected to be higher than that of standard ice cream.[Citation2] Surendra Babu et al.[Citation5] reported texture results of low-fat ice creams with a value of 27.62 N. While the hardness values of control ice creams were 13.05 N and 12.12 N. Mahdian and Karazhian[Citation24] noted that the reduction of fat in ice cream generates texture defects in the final product such as coldness, roughness, and brittle body. However, these characteristics were not generally observed in the evaluated treatments despite the sample with 33% CMC, 33% guar gum and 33% xanthan gum had a hardness close to that reported in literature.[Citation5]
Selection of the best treatments
A statistical desirability analysis was performed to select the best treatments to undergo a sensory acceptance evaluation together with two other treatments: in one, all additives (hydrocolloids, soy lecithin, carrageenan, gelatin) of the formulation were replaced by a commercial mixture (Tecnas) with the same fat content evaluated in the previous treatments, and the second had a reduced fat percentage to 7%, a change that generated an increase in the share of modified cassava starch by 40%. These treatments were selected to compare physicochemical properties and acceptance. The analysis showed that the best treatments were the one with only xanthan gum, and the 67% CMC and 33% guar gum, considering all the restriction parameters mentioned above. The 7% fat treatment formulation had only xanthan gum as hydrocolloid in the mixture, taking into account the mentioned desirability results.
Sensory analysis
The results of the sensory evaluation showed that the panelists adequately evaluated the selected treatments, and there were no significant differences between the established sensory parameters (). The sensory characteristics of the product would be expected to decrease by decreasing the amount of fat[Citation2]; but, because the panelists were not experts, they did not notice an increase in starch in the formulation, nor differences in the quality characteristics; therefore, the sensory result did not lead to a decrease in its general acceptance. This sensory result is very interesting, since the samples with such fat content, still close to 7%, with the proposed formulation could be marketed as a low-fat ice cream since consumers did not notice sensory differences versus the commercial mixtures. Although the sensory results do not show significant differences, it can be observed that the commercial sample has the highest hardness for the quality parameters evaluated, and the sample with 7% fat had the lowest drip-through time and the highest melting percentage (). The desirable effects of stabilizers on the sensory properties of ice cream result from their abilities to alter surface properties of the ice crystals or to alter their mouthfeel.[Citation11] All treatments presented an adequate and adjusted overrun. In addition, the commercial sample and the treatment with 7% fat had the highest viscosity of the mixture. The latter could be a constraint during processing, as equipment with specific capacity would be needed to transport the ice cream mixture. If the viscosity of the mixture is high, a laminar flow rather than a turbulent flow may result, and this could lead to a lack of control of the mixture because of the different maximum velocity ratios and the lack of mixing action across the diameter of the HTST conveying pipe or pasteurizers.[Citation19] Therefore, a laminar flow in the ice cream mix should be avoided as much as possible to prevent this type of inconvenience.
Table 4. Ice Cream Sensory Evaluation Score.
Table 5. Quality parameters of the four selected mixtures.
The viscosity of the ice cream mixture is important to impart desirable qualities to the final product, but the specific rheological parameters have not been well established. In general, as the viscosity increases, the melting strength and smoothness of the texture increase, but the creaming rate decreases. Although stabilizers have very beneficial functions in ice cream, their overuse can create problems. These limitations include undesirable melting characteristics, excess viscosity in the mixture, and contribution to a heavy and soggy body. Stabilizers have specific roles and rarely perform all desired functions so synergistic mixtures are often used. Trial and error are key to determine the correct combination and concentrations of the available hydrocolloids to perform the desired functions for a specific formulation and a niche market.[Citation11]
Conclusion
Applying modified cassava starch at 10% concentration and mixtures of hydrocolloids and emulsifiers at 0.3% concentration to the formulations resulted in increased viscosity, overrun, melting percentage, and drip-through time in ice cream. The best formulations according to the criteria established in the experiment were the treatments with the mixture of 67% CMC-33% guar gum and with 100% xanthan gum. Moreover, the results of the sensory acceptance test showed that there were no significant differences between low-fat ice creams with the established formulation and a sample made with a commercial stabilizer. It was compared sensorially with a sample with 7% fat, xanthan gum as a hydrocolloid, and a 40% share of modified starch; no significant difference was found with the other treatments. In general, low-fat ice creams with good physicochemical and quality properties were obtained. The viscosity, melting percentage, and overrun were higher than 5000 cP, 3.74%, and 93.55%, respectively. Therefore, fat substitutes used in this research may be an alternative to produce ice cream. It is recommended to compare the sensory characteristics of the best low-fat formulations with nonfat reduced commercial ice creams.
Credit authorship contribution statement
Sara Marimon-Valverde contributed to methodology, validation, formal analysis, and writing – original draft. Sebastián Lainez-Ramirez contributed to the investigation and formal analysis. José-Uriel Sepúlveda-Valencia contributed to methodology, validation, and conceptualization. Alejandro Mejia-Villota contributed to funding acquisition and conceptualization. Eduardo Rodriguez-Sandoval contributed to the investigation, methodology, resources, supervision, validation, visualization, and writing – review, and editing.
Disclosure statement
No potential conflict of interest was reported by the author(s).
Data availability statement
The datasets generated during and/or analyzed during the current study are available from the corresponding author on reasonable request
Additional information
Funding
References
- Swanson, B. G. Fat Replacers: Mimetics and Substitutes. In Nutraceutical and Specialty Lipids and Their Co-Products; Shahidi, F., Ed.; CRC Press, Taylor and Francis Group: Boca Raton, FL, 2006; Vol. Chap 20, pp 329–339.
- Akbari, M.; Mohammad, H. E.; Zahra, D. Application and Functions of Fat Replacers in Low Fat Ice Cream: A Review. Trends Food Sci. Tech. 2019, 86, 34–40. DOI: 10.1016/j.tifs.2019.02.036.
- Peng, X.; Yao, Y. Carbohydrates as Fat Replacers. Annu. Rev. Food Sci. Technol. 2017, 8, 331–351. DOI: 10.1146/annurev-food-030216-030034.
- Espinoza, L. A.; Purriños, L.; Centeno, J. A.; Carballo, J. Chemical, Microbial and Sensory Properties of a Chestnut and Milk Ice Cream with Improved Healthy Characteristics. Int. J. Food. Prop. 2020, 23, 2271–2294. DOI: 10.1080/10942912.2020.1856135.
- Surendra Babu, A.; Parimalavalli, R.; Jagan Mohan, R. Effect of Modified Starch from Sweet Potato as a Fat Replacer on the Quality of Reduced Fat Ice Creams. J. Food Meas. Charact. 2018, 12, 2426–2434. DOI: 10.1007/s11694-018-9859-4.
- Surapat, S.; Rugthavon, P. Use of Modified Starch as Fat Replacer in Reduced Fat Coconut Milk Ice Cream. Kasetsart J. Nat. Sci. 2003, 37, 484–492. DOI: 10.1007/s11694-018-9859-4.
- Azari-Anpar, M.; Khomeiri, M.; Ghafouri-Oskuei, H.; Aghajani, N. Response Surface Optimization of Low-Fat Ice Cream Production by Using Resistant Starch and Maltodextrin as a Fat Replacing Agent. J. Food Sci. Technol. 2017, 54, 1175–1183. DOI: 10.1007/s13197-017-2492-0.
- Sharma, M.; Singh, A. K.; Yadav, D. N. Rheological Properties of Reduced Fat Ice Cream Mix Containing Octenyl Succinylated Pearl Millet Starch. J. Food Sci. Technol. 2017, 54, 1638–1645. DOI: 10.1007/s13197-017-2595-7.
- AOAC. The Association of Official Analytical Chemists. Official Methods of Analysis. Horwitz W.and Latimer, G.W., Eds; 17th ed; Gaithersburg, MD, USA: AOAC International, 2000; pp 947.05, 981.12, 925.10.
- Goff, H. D.; Hartel, R. W. The ice cream industry. In Ice Cream, 7th ed, Goff, and H. D., and Hartel, R. W. Eds.; Springer Publishing: New York, 2013; pp. 14–16. DOI: 10.1007/978-1-4614-6096-1.
- Bahram-Parvar, M.; Mazaheri Tehrani, M. Application and Functions of Stabilizers in Ice Cream. Food Rev. Int. 2011, 27, 389–407. DOI: 10.1080/87559129.2011.563399.
- Bahram-Parvar, M.; Tehrani, M. M.; Razavi, S. M. Effects of a Novel Stabilizer Blend and Presence of κ-Carrageenan on Some Properties of Vanilla Ice Cream During Storage. Food Biosci. 2013, 3, 10–18. DOI: 10.1016/j.fbio.2013.05.001.
- Garmakhany, A. D.; Mirzaei, H. O.; Nejad, M. K.; Maghsudlo, Y. Study of Oil Uptake and Some Quality Attributes of Potato Chips Affected by Hydrocolloids. Eur. J. Lipid Sci. Technol. 2008, 110, 1045–1049. DOI: 10.1002/ejlt.200700255.
- ICONTEC. Norma técnica colombiana NTC-1239. In Helados y mezclas de helados, 3rd ed.; Bogota, Colombia: Instituto Colombiano de Normas Técnicas y Certificación (ICONTEC), 2002; pp 18.
- Pandiyan, C.; Annal Villi, R.; Kumaresan, G.; Rajarajan, G.; Elango, A. Effect of Incorporation of Whey Protein Concentrate on the Quality of Ice Cream. Anim. Sci. 2012, 8, 189–193.
- Clarke, C. The Science of Ice Cream. Royal Society of Chemistry, 2nd ed.; Cambridge, UK: Royal Society of Chemistry Publishing, 2015; pp 187.
- Marshall, R. T.; Goff, H. D.; Hartel, R. W. Ice Cream, 6th ed.; New York: Kluwer Academic/Plenum Publishers, 2003; pp 11–12.
- Schmidt, K. A.; Smith, D. E. Rheological Properties of Gum and Milk Protein Interactions. J. Dairy. Sci. 1992, 75, 36–42. DOI: 10.3168/jds.S0022-0302(92)77735-2.
- Goff, H. D.; Davidson, V. J. Flow Characteristics and Holding Time Calculations of Ice Cream Mixes in HTST Holding Tubes. J. Food Prot. 1992, 55, 34–37. DOI: 10.4315/0362-028X-55.1.34.
- Zhao, Y.; Khalesi, H.; He, J.; Fang, Y. Application of Different Hydrocolloids as Fat Replacer in Low-Fat Dairy Products: Ice Cream, Yogurt and Cheese. Food Hydrocolloids. 2023, 138, 138. DOI: 10.1016/j.foodhyd.2023.108493.
- Rezaei, R.; Khomeiri, M.; Kashaninejad, M.; Mazaheri-Tehrani, M.; Aalami, M. Effect of Resistant Starch and Aging Conditions on the Physicochemical Properties of Frozen Soy Yogurt. J. Food Sci. Technol. 2015, 52, 8164–8171. DOI: 10.1007/s13197-015-1895-z.
- Liu, X.; Sala, G.; Scholten, E. Role of Polysaccharide Structure in the Rheological, Physical and Sensory Properties of Low-Fat Ice Cream. Curr. Res. Food Sci. 2023, 7, 100531. DOI: 10.1016/j.crfs.2023.100531.
- Amador, J.; Hartel, R.; Rankin, S. The Effects of Fat Structures and Ice Cream Mix Viscosity on Physical and Sensory Properties of Ice Cream. J. Food Sci. 2017, 82, 1851–1860. DOI: 10.1111/1750-3841.13780.
- Mahdian, E.; Karazhian, R. Effects of Fat Replacers and Stabilizers on Rheological, Physicochemical and Sensory Properties of Reduced-Fat Ice Cream. J. Agric. Sci. Technol. 2013, 15, 1163–1174.