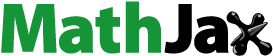
ABSTRACT
Foods with higher bioactive activities are being sought as a potential tool to prevent lifestyle-related disorders. In this context, germinated foods are preferred over conventional foods as they are rich in health promoting moieties. The current research was planned to assess the germination-induced modifications in physicochemical and bioactive profile of black chickpea (Cicer arietinum). For this purpose, black chickpeas (desi) were soaked for 12 h and germinated. The germinated black chickpeas were recovered after 24, 48, 72, 96, and 120 h of germination, separately, and their physicochemical and antioxidant profiles were studied. The phytochemical profiling of the germinated black chickpea revealed that germination time significantly influenced the chemical composition of black chickpea. It was observed that the phytochemical contents of the germinated chickpeas increased with an increase in germination time. However, the flavonoids such as rutin, luteolin, kaempferol, and quercetin were decreased, but isorhamnetin was increased as the germination time was extended. Moreover, the antioxidant capacity quantified by DPPH and ABTS activity of germinated chickpeas also increased with an increase in germination time. The highest content of DPPH antioxidant activity was observed at the 72 h of germination, while the highest concentration of ABTS was observed at the fourth day of germination. Conclusively, extended germination for 120 h can negatively influence the phytochemical and antioxidant profile of black chickpea, whereas optimum germination for 72 h was found satisfactory.
Introduction
Globally, legumes are regarded as nutritionally important and economically feasible component of the human diet, ancillary to cereals. Their eminence is confirmed by the diversification of their nutritional components which include proteins (20–45%), complex carbohydrates (60%), and dietary fiber (5–37%). Moreover, legumes are generally low in fats (~5%), barring chickpeas (15%), and peanuts and soybean with significantly higher fat content (45–50%). Additionally, an array of bioactive compounds are present in legumes that may protect against metabolic ailments.[Citation1] Due to low glycemic index and plethora of bioactive moieties, they are a pivotal alternative to cereals especially for people who are conscious in terms of their dietary intake and are therefore also a great choice for celiac and diabetic patients.[Citation2]
Legumes are consumed in a number of ways around the world[Citation3]; hence, hold a significant importance to fulfil dietary needs of low-income groups in developing countries. Furthermore, legumes are widely used in convenience food preparations such as canned foods, etc.[Citation4] Additionally, cultivation of legumes offers an alternative means of enriching soil with fertile ingredients via their symbiotic effect, significantly reducing the input costs alongside being more eco-friendly.[Citation5] Being a rich source of proteins and low in fat, legumes like chickpeas are preferred over other routine dietary choices.[Citation6] Chickpeas also provide sufficient economic return via exports to numerous countries.[Citation7] Despite these benefits, legumes cover far less cultivation area compared to cereals.[Citation6]
Chickpeas are commonly referred to as “the poor-man’s meat” owing to their high protein content which is a good protein alternative for vegetarians as well. Approximately 80% of the dry pulse mass is accounted for by protein and carbohydrates, it is also a rich source of minerals and vitamins.[Citation8] Consuming a cup of this pulse every day fulfills one-third of an adult’s daily protein requirement. Chickpeas contain fiber, vitamins, potassium, iron, magnesium, and selenium, all of which are excellent supporters of good heart health. An added bonus of chickpea consumption is the Selenium and beta-carotene antioxidants contained in them which help to remove free radicals and toxins that accumulate in the body as a consequence of metabolic processes occurring within the body, these antioxidants help in preventing cancer.[Citation9]
A number of techniques have been devised to increase the nutritional worth of seeds and grains.[Citation8] Germination augments the nutritional value of pulses and legumes by enhancing functional compounds that may prevent from various ailments.[Citation10] The germination of black chickpeas has been associated with a positive impact on its nutritional value. Germinated black chickpeas can be used to produce different convenience foods such as snacks, soups, and baked goods.[Citation11–13] These products are considered feasible due to their diversity in taste-profile and affordable cost. Chickpeas are preferred because they do not possess strong beany flavors that most other pulses do.[Citation14] In addition, another benefit of using this grain is the lack of some anti-nutritional compounds, such as trypsin inhibitors, that are commonly present in other beans.[Citation15]
The black chickpea’s nutritional characteristics such as total phenolic contents and antioxidant capability are greatly improved by germination. The significant protein and carbohydrate content of black chickpeas can improve properties such as thickening. As a result, black chickpea flour can be used to develop foods that have a balanced nutritional profile and increased sensory qualities.[Citation16] Amongst secondary metabolites, black chickpeas contain anti-nutritional components that can reduce their digestibility, nutritional value, and sensory quality. The suitable processing techniques, including germination, can minimize the negative impacts of the anti-nutritional components without compromising the desired sensory qualities of the black chickpeas. The current research was planned to explore the effect of germination time on quality characteristics of black chickpea such as physicochemical and antioxidant profiles.
Materials and methods
Procurement of raw materials
Black chickpeas (desi) were sourced from the local market followed by removal of any physically damaged grains and other unnecessary particles. The samples were stored in plastic bags before germination. The chemicals required were purchased from Sigma Aldrich. The distilled water was prepared at the laboratory using distillation assembly.
Germination of raw material
To produce germinated black chickpea samples, 3500 g of raw chickpeas were divided into seven equal batches each containing 500 g of the dry pulse. These batches were then processed according to their respective endpoints as indicated in . Black chickpea germinated for (a) 24 hours (b) 48 hours (c) 72 hours (d) 96 hours (e) 120 hours is shown in . After the germination time for each sample had been completed, the samples were mechanically crushed in a food processor to avoid any further enzymatic activity, ensuring that germination could no longer take place. Subsequently, the chickpeas were dried at a temperature of 65 ± 10°C till no moisture was visibly present, and a coarse texture was obtained. Each sample was then processed into a fine powdered form for further testing.
Table 1. Treatment Plan.
Extraction of phytochemicals
For the extraction of phytochemicals from non-germinated and germinated chickpea samples, an acidified methanol solution was made in which HCl, methanol, and double-distilled water were mixed in a ratio of 1:80:10, respectively. Following this, 15 g of each respective sample was dissolved in 150 mL of acidified methanol. The samples were then incubated at 25°C for 2.5 h. After incubation, the samples were kept in a shaking incubator at 30°C for 2 h. The extracts were then filtered using a doubled-up muslin cloth and then filtered twice with Whatman Filter Paper 42, to ensure that no residual chickpea flour was present. These extracts were then refrigerated until further use. The same protocol was repeated to produce a second batch of the extracts.
Proximate composition
The proximate composition of black chickpea samples including moisture (method 962.12, 41.1.02), crude fat (method 948.22, 40.1.05), and ash (method 942.05, 4.1.10) contents were estimated as per the guidelines established by AOAC,[Citation17] whereas the protein content was estimated via Bradford assay.[Citation18] The total carbohydrate contents were calculated via subtraction method.
Total phenolic content
The method reported by Straus et al.[Citation19] was used to determine the total phenolic contents. To quantify the total phenolic contents of chickpea samples, 0.1 mL sample extract, followed by 6 mL double distilled water, was added to a test-tube. The reaction mixture was then oxidized by adding 0.5 mL Folin-Ciocalteu Reagent and later neutralized with the addition of 1.5 mL of a 20% Sodium Carbonate solution. Finally, 1.9 mL double-distilled water was added to the test-tubes between 8 s to 8 min. This mixture was left to incubate for 30 min at 40°C, and the absorbances were taken at 765 nm using a spectrophotometer. Gallic acid was used as a standard. For this purpose, six different standard concentrations of gallic acid were prepared, i.e., 0, 10, 20, 30, 40, 50 ppm, using a 1000 ppm stock solution. These concentrations were treated similarly to the samples using the protocol explained earlier Acidified Methanol was used as a blank and the results were expressed as gallic acid equivalents (GAE)/g of black chickpea.
Total flavonoid content
The flavonoids in obtained extracts were assessed according to the guidelines of Heimler et al..[Citation20] To gauge the total flavonoid content of the samples, 1.25 mL of double distilled water was used to dilute 250 μL of the non-germinated and germinated chickpea extracts. A 5% Sodium Nitrite solution was prepared, and 75 μL of this was added to each diluted extract. The mixture was allowed to incubate for 6 min following which a 10% AlCl3 solution was prepared and a volume of 150 μL was added to each test-tube. The combination was left to react for 5 min after which 0.5 mL of a 1 M NaOH solution was added. Quercetin was used as a standard for the quantification of flavonoids in the sample. For this purpose, six different concentrations, i.e. 0, 10, 20, 30, 40, 50 ppm of quercetin were prepared from a 1000 ppm stock solution, and the spectrophotometric absorbance of the samples in triplicates was taken at 510 nm. Furthermore, the relation between the absorbance in relation to extinction coefficient at λmax was used to determine individual flavonoid[Citation21] as per below equation.
where A is absorbance value at λmax of respective flavonoid, DF is dilution factor, MW is molecular weight of respective flavonoid, e is extinction coefficient of respective flavonoid, l is path length (1 cm). Purposely, absorbance values for each sample were also taken in triplicates to check for the quantities of flavonoids known to be present in chickpeas such as Luteolin, Kaempferol, Isorhamnetin, and Rutin via spectrophotometric absorptions at 280 nm, 365 nm, 280 nm, 262 nm, respectively. The extinction coefficients used for calculating individual flavonoid concentrations are described in .
Table 2. Extinction coefficients and λ Max of Flavonoids.
Antioxidant activities
DPPH scavenging activity
The antioxidant potential of the germinated and non-germinated chickpea flours was determined on account of 2,2-Diphenyl-1-picrylhydrazyl (DPPH) radical scavenging effect. For this, the protocol outlined by Vulić et al.[Citation22] was followed according to which 1 mL of extract solution was mixed with 3 mL of methanol and 1 mL of 1 mM DPPH solution. This solution was allowed to vortex for 1 min, so it was properly mixed, after which it was allowed to stand for 10 min at 27°C. The absorbance of these samples was documented at 517 nm using a spectrophotometer. A blank was also prepared similarly in which the 1 mL of sample solution was replaced by methanol to act as a control. The DPPH scavenging activity was therefore calculated in accordance with the expression below.
where A is the absorbance of the control reaction mixture, B is absorbance of the sample reaction mixture.
ABTS scavenging activity
ABTS+ Scavenging Activity was measured according to the technique outlined by Raupp et al.[Citation23] with minor adaptations. For this, ABTS radicals were generated by mixing equal volumes of a 2.45 mM Potassium persulfate solution with 7 mM aqueous ABTS, which was incubated for 16 h in the dark at 4°C. Subsequently, 20 μL of the sample extract was added to 3 mL of the ABTS reagent, and this was incubated in a water bath at 30 ± 2°C for 30 min. Following this, the antioxidants present in the sample sequester unpaired electrons in the reaction mixture turning the solution’s color fade. A spectrophotometer was later used to record the absorbances of the samples at 734 nm, and a standard curve of Trolox was prepared to quantify the results.
Color profile
The color analysis of the non-germinated and germinated flours was carried out. For these, four parameters were measured, including the L (lightness), a* (-a; greenness or +a; redness), b* (-b; blueness or +b; yellowness) of the samples using a colorimeter. The data obtained were used to quantify the chroma and hue angles of the prepared prototypes, using the following expressions.
Statistical analysis
All the analyses were analyzed using one-way ANOVA using Statistix v8.1 software. Furthermore, the means were compared using Tukey’s honest significant difference (HSD) test. The statistical significance was considered to be p < .05.
Results
Proximate composition analysis
The proximate composition of the chickpea flours is an important determinant for evaluating the quality of the raw material. For the proximate analysis of the non-germinated and germinated chickpea flours, the moisture, ash, protein, fat, and total carbohydrate content of the samples were assessed.
Moisture content
The mean squares for the moisture content depicted highly significant (p < .01) differences among the treatments. The non-germinated and germinated chickpeas showed variations with respect to germination time as indicated in , where the non-germinated unsoaked chickpea (BG1) represented a moisture content of 11.38 ± 0.27%, BG2 that had only been soaked, and AG1 with a germination time of 24 h, showed moisture contents of 10.06 ± 0.22% and 9.93 ± 0.21%, accordingly. However, black chickpea AG2 with a germination time of 48 h, and AG3 with a germination time of 72 h, showed slightly higher moisture contents, i.e., 10.60 ± 0.35% and 12.02 ± 0.40%, respectively. The moisture content for AG4 slightly decreased (11.50 ± 0.12%) and AG5 showed an increase in moisture content, i.e., 13.31 ± 0.04%. The trend depicted an overall increase in the moisture content from the non-germinated sample BG1 to AG5 with a germination time of 120 h i.e., 11.38 ± 0.27% of BG1 to 13.31 ± 0.04% of AG5.
Table 3. Proximate composition (%) of germinated black chickpea.
Ash content
The statistical analysis of the ash content of the samples depicted non-significant differences (p > .05) among treatments. The non-germinated and germinated chickpeas showed inconsequential variations with respect to germination time as indicated in . The trend depicted an overall decrease in the ash content. The mean ash content for BG1 is 3.22 ± 2.18% which decreased for BG2 having an ash content of 1.68 ± 0.09%. The ash content for AG1 and AG2 increased with a value of 2.83 ± 0.22% and 3.03 ± 0.05%. The ash content of AG3, AG4, and AG5 decreased continuously as shown by their respective values of 2.65 ± 0.07%, 2.90 ± 0.31%, and 2.71 ± 0.11%, respectively.
Crude fat content
The statistical analysis of the fat content of the non-germinated and germinated chickpea samples is non-significant (p > .05). The non-germinated and germinated chickpeas showed inconsequential variations with respect to germination time as indicated in ; where the percentage crude fat was 4.00 ± 0.21% for BG1, 3.99 ± 0.30% for BG2, 4.22 ± 0.40% for AG1, 4.12 ± 0.64% for AG2, 4.21 ± 0.72% for AG3, 4.69 ± 0.75% for AG4, and 3.49 ± 0.21% for AG5, correspondingly. The sample with the highest fat content is AG4 with 92 h of germination, while the lowest fat content is present in AG5. The trend overall depicted an increase in fat content for germinated samples when compared with non-germinated variants.
Crude protein content
The mean squares for crude protein content depicted highly significant differences (p < .01) among the treatments. The non-germinated and germinated chickpeas showed variations with respect to germination time as indicated in . The trend followed for the non-germinated and germinated chickpeas shows an increase, where BG1, with no treatment had a percentage protein content of 14.80 ± 1.25%, BG2, which had undergone soaking for 12 h, has a protein content of 14.87 ± 0.46% ensuing which AG1, after being germinated for 24 h, had a protein content of 13.93 ± 2.50%, showing a slight decrease. The proceeding samples; AG2, AG3, AG4, and AG5 showed a significant increase with respective values of 14.27 ± 2.44%, 19.87 ± 1.21%, 16.33 ± 1.70%, and 20.93 ± 0.81% therefore displaying an increase in their crude protein content with an increase in germination time. The protein content for AG4 with a germination time of 96 h, was slightly lower as compared to the rest, following which AG5 with 120 h of germination, displayed the highest protein content in all the samples. The trend depicted an overall increase in the crude protein content after germination, from the first sample to the last i.e. 14.80 ± 1.25% of BG1 to 20.93 ± 0.81% of AG5. The values sharing similar alphabetical letters are statistically alike.
Total carbohydrate content
The statistical analysis of the total carbohydrate content depicted highly significant differences (p < .01) among the treatments. The non-germinated and germinated chickpeas showed variations with respect to germination time as indicated in . BG1, which underwent no treatment, had a percentage carbohydrate content of 66.59 ± 1.58%. BG2, after 12 h of soaking, had a content of 69.41 ± 0.95% and AG1, after 24 h of germination, had a carbohydrate content of 69.08 ± 3.12%. AG2, AG3, AG4, and AG5 with germination times of 48, 72, 96, and 120 h, respectively, showed a significant decrease in their carbohydrate content as 67.99 ± 2.63%, 61.26 ± 0.64%, 64.58 ± 1.74%, and 59.56 ± 0.99%. The carbohydrate content for BG2, after soaking and AG1, after 24 h of germination was slightly higher as compared to the rest, this can be explained by the interconversion of molecules at this stage, following which AG5 displayed the lowest carbohydrate content in all the samples. The trend depicted an overall decrease in the total carbohydrate content from BG1 to AG5 suggesting that carbohydrates were being broken down for the synthesis of other macromolecules such as proteins and fats which are required during germination.
Phytochemical profiling of Non-germinated and Germinated chickpea
Extract yield
The statistical analysis of the extract yield depicted highly significant differences (p < .01) among the treatments. The non-germinated and germinated chickpeas showed variations with respect to germination time as indicated in . A successive increase in their percentage yield is observed; from BG1 with a yield of 3.23 ± 0.06%, following which BG2 has a yield of 3.42 ± 0.09%, AG1, after 24 h of germination, had a yield of 3.87 ± 0.08%, AG2, after 48 h of germination, had a yield of 4.02 ± 0.08%, and AG3, after 72 h of germination, that shows the highest yield of 4.30 ± 0.09%. Samples with 96 and 120 h of germination, i.e., AG4 (3.83 ± 0.08%) and AG5 (3.96 ± 3.96%.) showed a slight decrease in their percentage yield, as compared to AG3 but still had a higher percentage yield when compared with the initial sample BG1. The trend therefore depicted an increase in percentage yields subsequently.
Table 4. Means for Total polyphenols (mg GAE/g) and Total flavonoids (µg QE/mL) of germinated black chickpea extracts.
Total polyphenols
The statistical analysis of the total polyphenols depicted highly significant differences (p < .01) among the treatments. The non-germinated and germinated chickpeas showed variations with respect to germination time as indicated in . A successive increase in phenolic content was observed with an increase in germination time; from BG1, having undergone no treatment, with a value of 42.15 ± 0.49 mg GAE/g, following which BG2 after 12 h of soaking has a phenolic content of 45.93 ± 0.41 mg GAE/g, AG1, after 24 h of germination, displays a total polyphenol value of 49.27 ± 1.81 mg GAE/g, AG2, after 48 h of germination had a content of 57.11 ± 0.81 mg GAE/g and AG3, after 72 h of germination showed a phenolic content of 59.92 ± 0.52 mg GAE/g. AG4, with a germination time of 96 h, carries forward the increase in the trend with a phenolic content of 66.72 ± 1.18 mg GAE/g. AG5 with the highest germination time showed the highest polyphenolic content as compared to the other samples, i.e., 77.76 ± 2.05 mg GAE/g. This suggests that with an increase in germination time, the polyphenolic content of the chickpeas increases.
Total flavonoids
The statistical analysis of the total flavonoids depicted highly significant differences (p < .01) among the treatments. The non-germinated and germinated chickpeas showed variations with respect to germination time as indicated in . The flavonoid content showed fluctuation with an initial decrease for the samples from BG1 with a flavonoid content of 193.89 ± 09.80 µg QE/mL, BG2 having 184.00 ± 16.82 µg QE/mL and AG1 with a corresponding flavonoid content of 156.89 ± 21.69 µg QE/mL which was the lowest one recorded. The total flavonoid content of AG2 increased significantly to 236.56 ± 18.08 µg QE/mL which again decreased for AG3 with a content of 203.56 ± 09.00 µg QE/mL. Both AG4 and AG5 later showed an increase for their flavonoid content with values of 258.55 ± 19.35 and 272.11 ± 14.69 µg QE/mL, respectively. According to the data obtained in this trial, AG5 represented the highest flavonoid content of all samples, meaning that a germination time for 120 h was optimal for ensuring high flavonoid contents. Overall, the trend showed an increase in total flavonoid content after germination. Further analysis of individual flavonoids present in Cicer arietinum was carried out, and their quantities before and after germination were quantified. The main flavonoids found in notable quantities included Rutin, Isorhamnetin, Luteolin, Kaempferol, and Quercetin.
Rutin
The statistical analysis of the flavonoid Rutin depicted highly significant differences (p < .01) among the treatments. The non-germinated and germinated chickpeas showed variations with respect to germination time as indicated in . According to data obtained from this analysis, the rutin content in BG1 was the highest with 1.26 ± 0.02 mg/mL. This successively decreased for each sample where BG2 had a rutin content of 1.24 ± 0.01 mg/mL, AG1 with a content of 1.22 ± 0.01 mg/mL, AG2 with a rutin content of 1.21 ± 0.01 mg/mL, AG3 with a content of 1.20 ± 0.02 mg/mL, AG4 with 1.19 ± 0.01 mg/mL and AG5, with a germination time of 120 h having the lowest rutin content of 1.18 ± 0.01 mg/mL. The trend therefore depicts a decrease in rutin content after germination.
Table 5. Means for certain flavonoids found in germinated black chickpea extracts.
Isorhamnetin
The statistical analysis of the flavonoid Isorhamnetin depicted highly significant differences (p < .01) among the treatments. The non-germinated and germinated chickpeas showed variations with respect to germination time as indicated in . The content of isorhamnetin initially decreased for samples, namely; BG1 with 0.29 ± 0.02 mg/mL, BG2 with 0.27 ± 0.03 mg/mL and AG1 with 0.23 ± 0.03 mg/mL which was the lowest content of isorhamnetin documented in all the samples. The samples following these, i.e. AG2, with 0.35 ± 0.03 mg/mL, AG3 with 0.30 ± 0.01 mg/mL, AG4 with 0.38 ± 0.03 mg/mL and AG5 with 0.40 ± 0.02 mg/mL all displayed an increase in isorhamnetin content with AG5, after 120 h of germination having the highest quantity of it, therefore signifying an overall increase in isorhamnetin content after germination.
Luteolin
The statistical analysis of the flavonoid luteolin depicted highly significant differences (p < .01) among the treatments. The non-germinated and germinated chickpeas showed variations with respect to germination time as indicated in . The content of luteolin showed a decrease for all the samples, namely; BG1 with a content of 0.27 ± 0.01 mg/mL, BG2, and AG1 with a value of 0.26 ± 0.01 mg/mL and AG2, AG3, AG4, and AG5 with similar values of 0.25 ± 0.01 mg/mL.
Kaempferol
The statistical analysis of the flavonoid Kaempferol depicted highly significant differences (p < .01) among the treatments. The non-germinated and germinated chickpeas showed variations with respect to germination time as indicated in indicating that BG1 has the highest kaempferol content of 0.46 ± 0.01 mg/mL among all the samples, BG2 and AG1 have a similar kaempferol content with the content 0.45 ± 0.01 mg/mL. AG2 and AG3 have a further decreased content of kaempferol with a content of 0.44 ± 0.01 mg/mL. The lowest quantity of this flavonoid was observed in AG4 and AG5 with a content of 0.43 ± 0.01 mg/mL, signifying an overall decrease in kaempferol content after germination.
Quercetin
The statistical analysis of the flavonoid quercetin depicted highly significant differences (p < .01) among the treatments. The non-germinated and germinated chickpeas showed variations with respect to germination time as indicated in Table 4.6, according to which the quercetin content is decreasing successively as the germination time increases. BG1 has a quercetin content of 16.46 ± 0.41 mg/mL, which decreases for BG2 with a content of 16.09 ± 0.27 mg/mL. AG1, after 24 h of germination has a quercetin content of 15.96 ± 0.17 mg/mL and this further decreases for AG2, after being germinated for 48 h, at 15.73 ± 0.41 mg/mL. AG3 has an even greater decrease with a content of 15.47 ± 0.08 mg/mL. AG4 has a quercetin content of 15.17 ± 0.22 mg/mL, and AG5, after 120 h of germination, has the lowest quantity of this flavonoid with a value of 15.08 ± 0.12 mg/mL indicating an overall decrease in quercetin content after germination.
Antioxidant activities
DPPH radical scavenging activity
The statistical analysis of the antioxidant capacity for DPPH depicted highly significant differences (p < .01) among the treatments. The non-germinated and germinated chickpeas showed variations with respect to germination time as indicated in . According to the data recorded, the percentage of DPPH shows an overall increase post-germination when compared with non-germinated samples. BG1 shows a DPPH content of 75.30 ± 0.53% which increases after soaking as observed in BG2 which has a reading of 75.83 ± 2.16%. The percentage of DPPH decreases after 24 and 48 h of germination which is supported by AG1 and AG2 having reduced contents of 72.43 ± 0.35% and 71.97 ± 0.57%, respectively. AG2 documents the lowest quantity observed. The highest amount of DPPH observed was in sample AG3 which was 79.09 ± 1.04%. After 96 and 120 h of germination, the DPPH contents showed a slight decrease with values of 76.52 ± 0.35% for AG4 and 76.44 ± 0.13% for AG5, respectively.
Table 6. Means for antioxidant activities of germinated black chickpea extracts.
ABTS scavenging activity
The statistical analysis of the antioxidant capacity for ABTS+ depicted highly significant differences (p < .01) among the treatments. The non-germinated and germinated chickpeas showed variations with respect to germination time as indicated in . According to this, the content of ABTS+ shows an increase post-germination when compared with non-germinated samples. The lowest amount of ABTS+ was observed in BG1, i.e.; before soaking or germination, with 26.87 ± 0.49 µg TE/mL. After soaking the samples for 12 h, as in BG2 the amount of ABTS+ increased, with a content of 27.60 ± 0.70 µg TE/mL. This again slightly decreased at 24 h of germination for AG1 with an ABTS+ content of 27.07 ± 0.23 µg TE/mL. For the samples AG2, AG3, and AG4 the ABTS+ content increased significantly with respective contents of 27.97 ± 0.57 µg TE/mL for 48 h of germination, 28.13 ± 0.35 µg TE/mL for 72 h of germination and 28.47 ± 0.49 µg TE/mL for 96 h of germination, which was also the highest amount observed in this trial. The amount again decreased slightly for AG5 with a content of 27.90 ± 0.80 µg TE/mL, suggesting that 4th day germination would result in optimal ABTS+ quantity.
Color profile of non-germinated and germinated chickpea
L value
The statistical analysis of color analysis in terms of the lightness depicted highly significant differences (p < .01) among the treatments. The non-germinated and germinated chickpeas showed variations with respect to germination time as indicated in . According to the data recorded, the values for lightness show a decrease for samples post-germination when compared with their non-germinated variants. BG1 is observed to have the highest content of 81.33 ± 0.99, for the lightness parameter, showing that it is the lightest among all samples. BG2 records a decrease with a content of 78.57 ± 0.55. As the samples achieve higher germination times, their lightness values decrease. At 24 h of germination, the sample AG1 has a lightness value of 78.37 ± 0.38, this further decreases at 48 h of germination all the way to 120 h of germination which shows the lowest content for this trial. The samples corresponding to their decrease in the lightness parameter are AG2 with a content of 75.93 ± 1.1, AG3 with a value of 75.8 ± 0.5, AG4 with a value of 74.9 ± 0.2, and finally, AG5 with a value of 74.83 ± 0.57, hence showing that AG5 at 120 h of germination was the darkest sample.
Table 7. Means for color profile of germinated and non-germinated black chickpea powders.
a* value
The statistical analysis of color analysis in terms of the redness (+a*) or greenness (-a*) depicted highly significant differences (p < .01) among the treatments. The non-germinated and germinated chickpeas showed variations with respect to germination time as indicated in . According to the data recorded, the values for this color parameter show fluctuation with an initial decrease in redness and later an increase in redness for the chickpea samples. The sample BG1, without any treatment, has a redness value of 2.73 ± 0.12, which decreases for the sample BG2, which has been soaked only and has a value of 2.7 ± 0.26 in terms of its redness. After 24 h of germination, AG1 has the lowest redness value of 2.37 ± 0.25. The other samples showed increase in their redness measure with AG2 having a value of 2.4 ± 0.17, AG3 reports a higher increase in redness with a value of 3.23 ± 0.35 which slightly decreases at 4 days of germination for AG4 which corresponds to 3.13 ± 0.32. The highest redness value was observed for AG5, after 120 h of germination with a value of 4 ± 0.17. The overall trend depicted an increase in the redness when the non-germinated samples were compared with the germinated ones.
b* value
The statistical analysis of color analysis in terms of the yellowness (+b*) or blueness (-b*) depicted highly significant differences (p < .01) among the treatments. The non-germinated and germinated chickpeas showed variations with respect to germination time as indicated in . According to the data recorded, the values for this color parameter showed fluctuation with the yellowness of the non-treated sample BG1 being the highest with a value of 21.17 ± 0.76. The amount of yellowness decreases for the samples BG2 and AG1 with values of 17.7 ± 0.52 and 17.5 ± 0.36, respectively. The yellowness for the sample AG2, after 48 h of germination, was observed to have the lowest value of 15.57 ± 0.31. AG3 after 72 h of germination reported a slight increase in yellowness with a value of 18.33 ± 0.49 after which AG4 and AG5 had decreased values of 16.77 ± 0.32 and 17.27 ± 0.47. The overall trend depicted a decrease in the yellowness when the non-germinated samples were compared with the germinated ones.
Chroma
The statistical analysis of color analysis in terms of the chroma parameter depicted highly significant differences (p < .01) among the treatments. The non-germinated and germinated chickpeas showed variations with respect to germination time as indicated in . According to the data recorded, the values for this color parameter showed fluctuation with BG1 having the highest value of 21.37 ± 0.76. This decreased notably for BG2 and AG1 with values of 17.93 ± 0.58 and 17.67 ± 0.35, respectively. The sample after 2 days of germination, i.e.; AG2 reported the lowest value for this parameter at 15.77 ± 0.21. AG3 reported the highest content of 18.57 ± 0.55 for chroma of the chickpea samples, while AG4 and AG5 presented a slight decrease at 17.1 ± 0.36 and 17.73 ± 0.51, respectively. The trend overall depicted a decrease in the chroma of the samples after germination, when compared to the non-germinated variants.
Hue angle
The statistical analysis of color analysis in terms of the hue angle depicted highly significant differences (p < .01) among the treatments. The non-germinated and germinated chickpeas showed variations with respect to germination time as indicated in . According to the data recorded, the hue angle of the samples showed fluctuation initially with BG1 having the highest reading of 82.67 ± 0.06. BG2, after soaking, reported a decrease with a value of 81.33 ± 0.47. AG1 after 24 h of germination showed a higher hue angle of 82.3 ± 0.72. The other samples with 48 (AG2), 72 (AG3), 96 (AG4) and 120 (AG5) h of germination all reported a continuous reduction of 81.3 ± 0.87, 79.97 ± 0.75, 79.37 ± 0.91, and 77.03 ± 0.40, respectively. Overall, the trend depicted a decrease in the hue angle of chickpeas after germination when compared to those that were not treated or soaked only.
Discussion
Functional foods are gaining much popularity due to their additional health benefits beyond basic nutrition.[Citation24] With a significant increase in population and a deterioration in the quality of life and diets of people, it is becoming progressively important to develop convenience foods that are readily available but also fulfill the dietary requirements of a population. The increasing demand and commercial viability of such foods makes their production not only accomplishable but also imperative. Secondary and tertiary processed chickpea edibles, including frozen, dehydrated, microwavable, and extruded products, can be used as a food therapy to eradicate nutritional deficiencies. The results obtained in this study can be used for many advanced opportunities for the production of legume formulations and products.
Germination is a gradual but natural biochemical process that takes place at low temperatures and suitable moisture contents to alter the metabolic activity and the breakdown of carbohydrates, thereby forming an array of other useful macro and micro nutrients.[Citation25,Citation26] This phenomenon augments the protein content, antioxidant activity, and nutritional bioavailability.[Citation27]
The moisture content of each flour is important in predicting its shelf life. The moisture contents obtained for this study were between 9.93 and 13.31 which is significant for microbial growth to develop. This higher moisture content could be due to increased soaking and germination times which were required to conduct metabolic activities needed for germination; however, no microbial growth was observed in any sample due to extraction in acidified methanol. The protein content of germinated samples when compared to their non-germinated variants showed an increase. This increase in protein content could be a result of amino acid production during germination, a similar trend was also reported by Tarasevičienė et al.[Citation28] and Kumar et al..[Citation29]
The carbohydrate content showed a decrease in trend from BG1 to AG5, so the non-germinated chickpea samples have a higher carbohydrate content as compared to the germinated samples. This can be explained by the utilization of carbohydrates to form amino acids which later formed proteins through polymerization and molecular conversion. The carbohydrate content observed in the samples was lower, which was not characterized between soluble and insoluble fractions. The decreased ash content can be explained by soaking and germination as this trend has also been observed before in previous studies done on soaked legumes like Labla purpureaus, Cejanus cajan, and Vignia ungulicalata as well as in chickpeas.[Citation30,Citation31] This reduction in ash content can be attributed to it being an energy source to start the process of germination.
The increase in the total phenolic content after germination could be accredited to the enzymatic degradation of the kernel structure.[Citation32] The results of this study are also similar to the change observed for the total phenolic content observed in the germination of moong.[Citation33] Another study reported the highest quantity of phenolic compounds in chickpeas after 4 days of germination. This is because phenolics that are previously bound to cell wall substances such as pectin, lignin, cellulose, hemicellulose, proteins, and carbohydrates, are made free by the breakage of covalent bonds.[Citation34] The percentage yield of the samples also increased with an increase in germination time.
The flavonoid content of the germinated samples also varied from their non-germinated alternatives. In general, the flavonoid content increased with germination time, with the highest value being observed at fifth day of germination. Between the five particular flavonoids studied, the only flavonoid that showed an increase was isorhamnetin, while rutin, luteolin, kaempferol, and quercetin all decreased continuously with an increase in germination time. One possible explanation for this is the transformation or metamorphosis of one flavonoid into another. The antioxidant capacities of the black chickpea also increased with an increase in germination time with the highest content observed at the third and fourth day of germination. Studies carried out in the past support the increase of antioxidant activity with an increase in germination time, this trend was also observed by López-Amorós et al.[Citation35] Similarly, research carried out by Saleh et al.[Citation36] supported that the antioxidant activity increased as the germination time increased. This increase in antioxidant capacity is due to the active macro and micro elements that are already present in the seed. Since chickpeas are a rich source of these nutrients, they are also a useful source of antioxidants. The highest antioxidant compounds are reportedly found in germinated chickpeas.
Color is an important parameter which is a strong determinant of the qualitative analysis of germinated and non-germinated chickpea samples. In this study, the lightness of the samples decreased with an increase in germination time and the redness and greenness of the samples increased as the samples underwent germination. The saturation and the hue angle of the samples also decreased with an increase in germination time. The same trends were also observed by Kumar et al.[Citation29] Concisely, the overall quality of the black chickpea is influenced by germination, where extended germination may cause deleterious effects on the quality of the commodity.
Conclusion
Germination of legumes is considered a potential process to increase the functional worth of this commodity. However, the extent of germination is important to obtain promising functional properties for product development with additional benefits. In general, crude fat and as contents of black chickpea remain unchanged; however, significant differences in protein and carbohydrate contents were seen as the germination progressed. It was noted that germination improved the bioactive potential of the black chickpea. However, extended germination for 120 h can negatively influence the phytochemical and antioxidant profile of black chickpea. In this milieu, optimum germination with improved bioactive profiles was observed till 72 h. Conclusively, black chickpea with up to 72 h of germination can be considered for further investigation on their potential use in different culinary items.
Acknowledgments
This project was supported by Researchers Supporting Project number (RSP2024R5) King Saud University, Riyadh, Saudi Arabia.
Disclosure statement
No potential conflict of interest was reported by the author(s).
References
- Raju, J.; Mehta, R. Cancer Chemopreventive and Therapeutic Effects of Diosgenin, a Food Saponin. Nutr. Cancer. 2008, 61, 27–35. DOI: 10.1080/01635580802357352.
- Maphosa, Y.; Jideani, V. A. The Role of Legumes in Human Nutrition. Functional Food-Improve Health Through Adequate Food. 2017, 1, 13.
- Siddiq, M.; Uebersax, M. A. Dry Beans and Pulses: Production, Processing, and Nutrition; John Wiley & Sons: Ames, Iowa, USA, 2022.
- Njintang, N.; Mbofung, C.; Waldron, K. In vitro Protein Digestibility and Physicochemical Properties of Dry Red Bean (Phaseolus Vulgaris) Flour: Effect of Processing and Incorporation of Soybean and Cowpea Flour. J. Agric. Food Chem. 2001, 49(5), 2465–2471. DOI: 10.1021/jf0011992.
- FAO. Pulses are Praised for Health, Ecological and Economic Benefits. Food & Agriculture Organization - United Nations. https://www.fao.org/newsroom/detail/Pulses-are-praised-for-health-ecological-and-economic-benefits/en, (accessed 17 October 2023).
- Merga, B.; Haji, J.; Yildiz, F. Economic Importance of Chickpea: Production, Value, and World Trade. Cogent Food Agric. 2019, 5(1), 1615718. DOI: 10.1080/23311932.2019.1615718.
- OEC, Chickpeas, dried, shelled https://oec.world/en/profile/hs/chickpeas-dried-shelled, (accessed 2 September 2023).
- Jukanti, A. K.; Gaur, P. M.; Gowda, C.; Chibbar, R. N. Nutritional Quality and Health Benefits of Chickpea (Cicer Arietinum L.): A Review. Br. J. Nutr. 2012, 108(S1), S11–S26. DOI: 10.1017/S0007114512000797.
- Francis, K. R., Chickpeas: Health Benefits and Nutritional Information, 2023, https://www.medicalnewstoday.com/articles/280244#benefits, (accessed August 2023).
- Malleshi, N.; Klopfenstein, C. Proximate Composition, Amino Acid and Vitamin Contents of Malted Chickpea, Mungbean and Their Seed Coats. J. Food Sci. Technol. 1996, 33, 479–482.
- Bravo, L.; Siddhuraju, P.; Saura-Calixto, F. Composition of Underexploited Indian Pulses. Comparison with Common Legumes. Food Chem. 1999, 64(2), 185–192. DOI: 10.1016/S0308-8146(98)00140-X.
- Kon, S.; Burtea, O. Process Development Adds Scope to Bean Products. Food Product Development. 1979, 13, 48–49.
- Singh, N.; Kaur, M.; Sandhu, K. S.; Guraya, H. S. Physicochemical, Thermal, Morphological and Pasting Properties of Starches from Some Indian Black Gram (Phaseolus Mungo L.) Cultivars. Starch‐Stärke. 2004, 56, 535–544. DOI: 10.1002/star.200400290.
- Walker, A. F.; Kochhar, N. Effect of Processing Including Domestic Cooking on Nutritional Quality of Legumes. Proc. Nutr. Soc. 1982, 41, 41–51. DOI: 10.1079/PNS19820006.
- Salunkhe, D. Legumes in Human Nutrition: Current Status and Future Research Needs. Current Science. 1982, 51, 387–394.
- Perez-Perez, L. M.; Huerta-Ocampo, J. Á.; Ruiz-Cruz, S.; Cinco-Moroyoqui, F. J.; Wong-Corral, F. J.; Rascón-Valenzuela, L. A.; Robles-García, M. A.; González-Vega, R. I.; Rosas-Burgos, E. C.; Corella-Madueño, M. A. G. Evaluation of Quality, Antioxidant Capacity, and Digestibility of Chickpea (Cicer Arietinum L. Cv Blanoro) Stored Under N2 and CO2 Atmospheres. Molecules. 2021, 26, 2773. DOI: 10.3390/molecules26092773.
- AOAC. Official Methods of Analysis of Association of Official Analytical Chemists International, 18th ed.; AOAC Press: VA, USA, 2006.
- Filgueiras, M. F.; Borges, E. M. Quick and cheap colorimetric quantification of proteins using 96-well-plate images. J. Chem. Educ. 2022, 99, 1778–1787. DOI: 10.1021/acs.jchemed.1c00756.
- Straus, S.; Bavec, F.; Turinek, M.; Slatnar, A.; Rozman, C.; Bavec, M. Nutritional Value and Economic Feasibility of Red Beetroot (Beta Vulgaris L. Ssp. Vulgaris Rote Kugel) from Different Production Systems. Afr. J. Agric. Res. 2012, 7, 5653–5660. DOI: 10.5897/AJAR12.1519.
- Heimler, D.; Vignolini, P.; Dini, M. G.; Romani, A. Rapid Tests to Assess the Antioxidant Activity of Phaseolus Vulgaris L. Dry Beans. J. Agric. Food Chem. 2005, 53(8), 3053–3056. DOI: 10.1021/jf049001r.
- Haq I.U.; Butt, M. S.; Randhawa, M. A.; Shahid, M. Extraction, Characterization and Optimization of Betalains from Red Beetroot Using Response Surface Methodology. Pak. J. Agric. Sci. 2020, 57(2), 535–545.
- Vulić, J. J.; Ćebović, T. N.; Čanadanović-Brunet, J. M.; Ćetković, G. S.; Čanadanović, V. M.; Djilas, S. M.; Šaponjac, V. T. T. In vivo and in vitro Antioxidant Effects of Beetroot Pomace Extracts. J. Funct. Foods. 2014, 6, 168–175. DOI: 10.1016/j.jff.2013.10.003.
- Raupp, D. D. S.; Rodrigues, E.; Rockenbach, I. I.; Carbonar, A.; Campos, P. F. D.; Borsato, A. V.; Fett, R. Effect of Processing on Antioxidant Potential and Total Phenolics Content in Beet (Beta Vulgaris L. Food Sci. Technol. 2011, 31, 688–693. DOI: 10.1590/S0101-20612011000300021.
- Butt, M. S.; Tariq, U.; Lahtisham, U.-H.; Naz, A.; Rizwan, M. Neuroprotective effects of oleuropein: Recent developments and contemporary research. J. Food Biochem. 2021, 45, e13967. DOI: 10.1111/jfbc.13967.
- Duenas, M.; Sarmento, T.; Aguilera, Y.; Benitez, V.; Molla, E.; Esteban, R. M.; Martín-Cabrejas, M. A. Impact of Cooking and Germination on Phenolic Composition and Dietary Fibre Fractions in Dark Beans (Phaseolus Vulgaris L.) and Lentils (Lens Culinaris L. LWT Food Sci. Technol. 2016, 66, 72–78. DOI: 10.1016/j.lwt.2015.10.025.
- Wu, F.; Yang, N.; Touré, A.; Jin, Z.; Xu, X. Germinated Brown Rice and Its Role in Human Health. Crit. Rev. Food Sci. Nutr. 2013, 53(5), 451–463. DOI: 10.1080/10408398.2010.542259.
- Singh, A.; Sharma, S.; Singh, B. Effect of Germination Time and Temperature on the Functionality and Protein Solubility of Sorghum Flour. J. Cereal Sci. 2017, 76, 131–139. DOI: 10.1016/j.jcs.2017.06.003.
- Tarasevičienė, Ž.; Danilčenko, H.; Jariene, E.; Paulauskienė, A.; Gajewski, M. Changes in Some Chemical Components During Germination of Broccoli Seeds. Notulae Botanicae Horti Agrobotanici Cluj-Napoca. 2009, 37, 173–176.
- Kumar, Y.; Sharanagat, V. S.; Singh, L.; Mani, S. Effect of Germination and Roasting on the Proximate Composition, Total Phenolics, and Functional Properties of Black Chickpea (Cicer Arietinum). Legume Sci. 2020, 2(1), e20. DOI: 10.1002/leg3.20.
- Dida Bulbula, D.; Urga, K. Study on the effect of traditional processing methods on nutritional composition and anti nutritional factors in chickpea (Cicer arietinum). Cogent Food Agric. 2018, 4(1), 1422370. DOI: 10.1080/23311932.2017.1422370.
- Ragab, H.; Kijora, C.; Ati, K. A.; Danier, J. Effect of Traditional Processing on the Nutritional Value of Some Legumes Seeds Produced in Sudan for Poultry Feeding. International J. Of Poultry Science. 2010, 9, 198–204. DOI: 10.3923/ijps.2010.198.204.
- Tian, B.; Xie, B.; Shi, J.; Wu, J.; Cai, Y.; Xu, T.; Xue, S.; Deng, Q. Physicochemical Changes of Oat Seeds During Germination. Food Chem. 2010, 119(3), 1195–1200. DOI: 10.1016/j.foodchem.2009.08.035.
- Sharanagat, V. S.; Kumar, P.; Patro, S.; Ghule, P. D.; Naryal, S.; Meena, S.; Singh, L.; Kumar, Y.; Gundev, P.; Nagar, M. Influence of Germination on Physicochemical, Thermo‐Pasting, and Antioxidant Properties of Moong Grain (Vigna Radiata. J. Food Process. Preserv. 2019, 43, e13922. DOI: 10.1111/jfpp.13922.
- Bhinder, S.; Kumari, S.; Singh, B.; Kaur, A.; Singh, N. Impact of Germination on Phenolic Composition, Antioxidant Properties, Antinutritional Factors, Mineral Content and Maillard Reaction Products of Malted Quinoa Flour. Food Chem. 2021, 346, 128915. DOI: 10.1016/j.foodchem.2020.128915.
- López-Amorós, M.; Hernández, T.; Estrella, I. Effect of Germination on Legume Phenolic Compounds and Their Antioxidant Activity. J. Food Compost. Anal. 2006, 19(4), 277–283. DOI: 10.1016/j.jfca.2004.06.012.
- Saleh, H. M.; Hassan, A. A.; Mansour, E. H.; Fahmy, H. A.; El-Bedawey, A. E.-F. A. Melatonin, Phenolics Content and Antioxidant Activity of Germinated Selected Legumes and Their Fractions. J. Saudi Soc. Agric. Sci. 2019, 18, 294–301. DOI: 10.1016/j.jssas.2017.09.001.