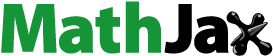
ABSTRACT
Coating materials and fluctuations in inlet temperature are crucial components of the encapsulation process, with both factors affecting the physical, chemical, and functional qualities. Therefore, this study aimed to determine the optimal properties of Jack Bean tempeh hydrolyzate using maltodextrin and Gum Arabic coating materials at a specific spray drying temperature. The experimental method consisted of 80% (w/w) maltodextrin, 20% (w/w) Gum Arabic, and a combination of both, with variations in inlet temperature at 140°C, 150°C, and 160°C. The results showed that 20% Gum Arabic treatment at a spray drying temperature of 150°C produced the best hydrolyzate characteristics of Jack Bean tempeh. Based on the SEM analysis, the morphology of the hydrolyzate particle had a spherical and wrinkled shape. Additionally, FTIR results showed no significant structural difference between the sample treatment and the uncoated control.
Introduction
The high protein content in Jack beans is a potential substitute for soy in the manufacture of tempeh. The digestibility value is significantly higher compared to that of soybeans as a raw material due to the ability of the body to easily digest and absorb the protein content.[Citation1] Starch and protein hydrolyzates have been used as an ingredient to improve food product quality and functionality. Specifically, protein hydrolyzate is widely used in the bakery industry, as a thickener, emulsion stabilizer, and gel-forming agent.[Citation2] The ability of proteins to be used naturally is limited by their sensitivity to environmental factors such as temperature and acidic conditions, solubility, limited digestion, and the presence of certain allergenic compounds.[Citation3] To address this issue, encapsulation has been proposed as a practical solution.[Citation4] Microencapsulation is a process used to encase particles or droplets, protecting the core material from the environment, and controlling the release. Encapsulated materials are protected from adverse reactions, including lipid oxidation, as well as nutrient degradation or loss of volatiles during production, storage, and handling. Furthermore, the final spray-dried product has high durability, reducing the possibility of damage caused by microbes or chemicals. These advantages make the spray drying process an effective means to encapsulate concentrates.[Citation3] The method is particularly valuable in extending the shelf-life of protein hydrolyzate, which is transformed into a stable, dehydrated form.[Citation5]
The most common coating materials used to encapsulate protein include maltodextrin and Gum Arabic due to their excellent barrier properties, high water solubility, neutral taste, smell, and color. Maltodextrin has weaknesses, including low emulsion ability, and poor film formation, showing the need to be combined with other materials to achieve stable results. On the other hand, Gum Arabic has a superior ability to hydrate and interact with water, resulting in enhanced emulsion stability.[Citation6] The combination of maltodextrin and Gum Arabic can offer a balance between encapsulation efficiency (EE), protection, cost, and product quality. Maltodextrin provides the bulk of the encapsulation matrix, while Gum Arabic enhances the stability and emulsification, leading to better encapsulation quality. The 80% maltodextrin and 20% Gum Arabic formulation may balance the strengths of both materials, providing an effective and economical solution for microencapsulation. The exact ratios can vary based on the specific requirements of the encapsulated material and the desired characteristics of the final product.
The amount of water present may vary depending on the inlet temperature during spray drying, the higher the amount present, the lower the yield generated. Furthermore, the inlet temperature can affect the structural, physical, chemical, and functional properties of the encapsulated material.[Citation7] This study explored the optimization of encapsulating Jack Bean tempeh hydrolyzate, focusing on the efficacy of different coating materials and the impact of varying inlet temperatures during the spray drying process. The primary objective was to enhance the physical, chemical, and functional attributes of hydrolyzate through effective microencapsulation. Maltodextrin and Gum Arabic were used as coating materials, examining their influence when used in different proportions. The experimental framework entailed using 80% (w/w) maltodextrin, 20% (w/w) Gum Arabic, as well as a blend of the two, under varying inlet temperatures set at 140°C, 150°C, and 160°C.
Materials and Methods
The materials used included Jack bean (Canavalia ensiformis L) obtained from farmers in Agromulyo, Salatiga, Central Java, as well as tempeh yeast, maltodextrin, Gum Arabic, distilled water, NaOH, HCl, alcohol, Kjeldahl tablets, concentrated sulfuric acid (H2SO4), boric acid (H3BO3), HCl, and the indicator methyl red. This study was carried out at the Padjadjaran University, Sumedang, Indonesia, from January to June 2023.
Hydrolysate encapsulation process
Jack Bean Tempeh
The Jack beans were sorted first by selecting those that were large, white in color, and had no impurities. The sorted samples were washed thoroughly and boiled for 30 minutes. This was followed by soaking for 24 hours, then the seeds were cut into small pieces and steamed for 45 minutes. The steamed Jack beans were inoculated with yeast at a concentration of 1.5% and subsequently wrapped in Polypropylene plastic which had been previously perforated. Furthermore, fermentation was carried out for 36 hours at room temperature.[Citation8]
Jack Bean Tempeh Flour
The Jack bean tempeh was thinly sliced with a maximum thickness of 1.5 cm and placed in an oven set to ± 50°C for 24 hours. To create fine flour, the samples were crushed in a grinder and filtered through an 80 mesh.
Hydrolysate Jack Bean Tempeh
Jack bean tempeh flour was dissolved in water (1:10, w/v), and added with 2N NaOH followed by stirring with a magnetic stirrer for 30 minutes at 30°C, adjusting the suspension to a pH of 10. The supernatant was collected after centrifugation at 4000 rpm for 15 minutes. By adding 2N HCl and stirring on a magnetic stirrer for 10 minutes at 30°C, the supernatant was adjusted to an isoelectric pH of 4.2. The precipitate was collected after centrifugation at 4000 rpm for 15 minutes. Furthermore, the defatting procedure was carried out by diluting 70% alcohol in a ratio of 1:3 with wet protein precipitate followed by homogenization for an hour with a magnetic stirrer. The suspension was centrifuged at 4°C for 10 minutes at 4000 rpm. The protein precipitate or hydrolyzate was then weighed and encapsulated using the spray drying method.[Citation9]
Encapsulation of Jack Bean Tempeh Hydrolysate
Encapsulation referred to Burger et al.[Citation9] where Maltodextrin (80%) and Gum Arabic (20%) were prepared in the ratio 80: 20, followed by stirring for ±15 minutes. This encapsulant was mixed with the sample and dissolved in a ratio of 2:1. The sample and encapsulant combination was homogenized for one minute at 10,000 rpm. Spray drying was used to encapsulate the combined solution, with inlet temperatures of 140°C, 150°C, and 160°C.
Chemical analysis of jack bean tempeh hydrolysate
Protein Content
Protein content was determined using the Kjeldahl method, in which about 0.5 g of hydrolyzate powder was weighed into the Kjeldahl flask, followed by the addition of the tablets. Furthermore, 10 mL of concentrated sulfuric acid (H2SO4) was added and this mixed solution was destroyed for 2 hours. The solution was diluted with 25 mL of distilled water and 3% boric acid (H3BO3) was added up to 30 mL with 2–3 drops of indicator methyl red. This was followed by distillation and titration with 0,02 N HCl, and the conversion factor of % Beans * 6,25 was used to obtain the % Protein.[Citation10]
Physical analysis of jack bean tempeh hydrolysate
EE
EE was determined according to Kumari et al.[Citation11] The hydrolyzate powder was prepared with a 5 mg/mL concentration, centrifuged for 5 minutes at 3000×g, and the supernatant (1 mL) was used to determine protein concentration through the Bradford method at a wavelength of 595 nm. EE was calculated by the formula:
Mass Yield
Mass Yield was determined in line with Pratiwi and Purwantini[Citation12] based on the ratio of the mass encapsulated product to the total solids mass before encapsulation.
Scanning electron microscopy (SEM)
SEM Analysis was conducted according to Bittencourt et al.[Citation13] The samples were placed on the carbon conductive tape affixed to an aluminum SEM stub. Subsequently, a thin layer of gold was applied using the gold-sputtering method and SEM was operated at 15 kV.
Fourier transformed infrared (FTIR) analysis
FTIR Analysis was carried out according to Andrade et al.[Citation14] FTIR waves were read in the mid-infrared region of the spectrum at a wavelength range of 400–4000 cm−1.
Statistical analysis
Data analysis was performed using one-way analysis of variance (ANOVA) to assess significant differences between samples. Statistical analysis was performed with SPSS software, and a p-value <.05 showed significant differences.
Results and discussion
Protein content
The results for Hydrolysate tempeh protein content in Jack beans are illustrated in . The protein content for the 80% maltodextrin − 20% Gum Arabic at 150°C (22,67 ± 0,10a) and 140°C (22,76 ± 0,08a) samples was not significantly different. Other treatments with a different notation showed significant differences at a 95% significance level. This suggested that the encapsulant prepared with 80% maltodextrin and 20% Gum Arabic, along with variations in inlet temperature affected the protein content. The addition of high maltodextrin had no significant effect on the protein content due to polysaccharide carbohydrate composition.[Citation15] Based on the results, the addition of Gum Arabic increased the protein value of the Jack Bean Tempeh Hydrolysate attributed to the composition of the arabinogalactan protein (AGP) and glycoprotein (GP) groups. The non-covalent linkages between these polypeptides which acted as emulsifiers and thickeners helped bind the protein extract.[Citation16] The amount of protein increased with higher concentrations of Gum Arabic. The combination treatment showed the lowest protein content compared to other treatments. This could be attributed to the higher ratio of maltodextrin, namely 80% compared to Gum Arabic, which was only 20%. Gum Arabic can influence the rehydration and dispersibility of proteins after drying. The hydrophilic properties of the structure help proteins quickly absorb water during rehydration, allowing a fast return to the original state after drying.[Citation17,Citation18]
Table 1. The Impact of Adding Maltodextrin and Gum Arabic on Hydrolysate Protein Content.
According to Abidin et al.,[Citation19] the addition of maltodextrin reduced the protein content in the final product. The results showed that at 150°C, there was an increase in protein levels but at 160°C, a decrease was observed. As stated by Emmawati et al.,[Citation20] higher temperatures can lead to denaturation and a subsequent decrease in protein levels. Besides, Gum Arabic might help maintain the protein structure in a semi-denatured state, which still allows for functionality and offers protection during the encapsulation process, resulting in higher protein retention.[Citation21]
EE
The results for Hydrolyzate tempeh EE are shown in . The protein content analysis showed that the 80% Maltodextrin (96,88 ± 0,057e)% and 20% Gum Arabic at 150°C (96,88 ± 0,05e)% samples were not significantly different. Other treatments with different notations showed significant differences at the 95% significance level. This suggested that the encapsulant prepared with 80% maltodextrin and 20% Gum Arabic, along with variations in inlet temperature affected EE. Factors affecting EE include the nature of the coating material and the ratio of material to coating. The use of 80% maltodextrin had no significant effect due to its weak emulsifying properties.[Citation22] On the other hand, the combination of 80% maltodextrin with 20% Gum Arabic resulted in the highest value. EE refers to the capacity of the microcapsules to encapsulate the maximum amount of protein hydrolyzate with minimal leakage. According to Premi and Sharma, [,Citation23] the results obtained were relatively higher for the microcapsules produced with the combination of both coating materials. The EE also improved consistently with increasing Gum Arabic content. The combination treatment led to a higher emulsion viscosity, which promoted faster drying. Gum Arabic acts as an emulsifier and stabilizer, creating an efficient protective matrix surrounding the core material, which can increase EE.[Citation24] Besides, maltodextrin helps in reducing the stickiness and improving the solubility of Gum Arabic, which can enhance the overall stability of the encapsulated compound. In the combination treatment, the EE decreased at higher temperatures, presumably due to the faster spray drying rate. A high spray dying rate shows faster evaporation, resulting in uncontrolled expansion and damage to the surface of the particles.[Citation25]
Table 2. The Impact of Adding Maltodextrin and Gum Arabic on Hydrolysate EE.
Mass yield
The results for Hydrolysate tempeh mass yield are presented in . Mass yield is a crucial factor determining economic value and effectiveness. In this study, the best mass yield of 20,67% was obtained from the combination treatment of 80% maltodextrin with 20% Gum Arabic at 160°C. The addition of 80% maltodextrin increased the volume and raised the amount of the total solids in the material, thereby improving the yield. The use of 20% Gum Arabic produced the lowest mass yield compared to other treatments. However, a previous study by Arepally and Goswami,[Citation26] stated that an increased ratio of Gum Arabic could improve mass yield. Based on the results, the total solids increased with higher maltodextrin and Gum Arabic concentrations. According to Fitriyani,[Citation10] a high amount of maltodextrin supported improved yield. Gum Arabic is also renowned for having a great capacity to create films, which can improve encapsulation outcomes.[Citation27] At lower inlet temperatures, the mass yield results appeared to decrease. Low evaporation rates due to lower inlet temperatures produced high-density membranes in the microcapsules, which had high moisture contents, poor fluidity, and prone to agglomeration. Consequently, the yield decreased because the particles adhered to the inner walls of the drying chamber. Low inlet temperatures may not provide sufficient airflow to completely dry the product, leaving some water content, which leads to adhesion and lower yield.[Citation25] On the other hand, the results showed an increase in mass yield at higher temperatures. Purnamayati et al.,[Citation28] stated that higher inlet temperatures would produce greater yield. This was attributed to the improved heat and mass transfer efficiency, quicker water drainage, and particles clinging to the dryer walls.[Citation3] As reported by Dewi and Satibi,[Citation29] higher inlet temperature results in drier encapsulation outcomes with minimal adhesion to the walls of the drying chamber, leading to greater yield. However, excessive inlet temperatures may also result in high evaporation, membrane cracking, and degradation or loss of the encapsulation core.[Citation30]
Table 3. The Impact of Adding Maltodextrin and Gum Arabic on Hydrolysate Mass Yield.
SEM
SEM conducted at a resolution of 2000× and 15 kV was carried out to investigate the external morphology and size distribution of Jack Bean Tempeh Hydrolysate particles. The results in showed a spherical and wrinkled shape, with the distance at a lower temperature of 140°C appearing more tenuous than at 160°C. In the 80% maltodextrin treatment, the morphology of the particles showed varying sizes. At 140°C, the smallest and largest particle diameters reached 3.01 µm was 6.91 µm, while at 150°C, the values were 5.33 µm and 12.13 µm respectively. At 160°C, the smallest and largest particle diameter was 2.66 µm and 9.71 µm respectively. In the 20% Gum Arabic treatment, the morphology of the particles also showed varying sizes. At inlet temperatures of 140°C, 150°C, and 160°C, the smallest and largest particle diameter was 1.93 and 9.21 µm, 2.52 µm and 13.71 µm, as well as 5.39 µm and 6.47 µm respectively. In the combination treatment of 80% maltodextrin and 20% Gum Arabic, the morphology of the particles also showed different sizes. At inlet temperatures of 140°C, 150°C, and 160°C, the smallest and largest particle diameters were 1.38 µm and 6.78 µm, 3.53 µm and 10.97 µm, as well as 2.58 µm and 5.50 µm respectively. Gum Arabic has a higher resistance to drying conditions and can affect the formation of microcapsules without cracks.[Citation31] The combination with other encapsulants produces a smoother particle surface compared to its individual use.[Citation32] In general, the shape of the microcapsules in all of these treatments was spherical and wrinkled. According to Hasna et al.,[Citation33] a good microcapsule with a round shape shows that the active ingredient is well encapsulated. The wrinkled structure on the surface signifies a decrease in efficiency encapsulation. The microcapsules ruptured due to the excessive temperature, leading to a reduction in efficiency. Based on the observations, the rate of shrinkage at lower inlet temperatures was greater compared to higher levels. As stated by Correâ-Filho et al.,[Citation34] lower inlet temperatures lead to a slower drying rate, resulting in even particle shrinkage and reduced size. On the other hand, higher inlet temperatures increase the drying rate, causing the particles to form a hard crust that impedes further contraction, leading to larger particles.
Figure 1. Morphology of Hydrolysate Jack Bean Tempeh at 2000x p1=80% Maltodextrin 140°C; p2= 80% Maltodextrin 150°C Resolution; p3= 80% Maltodextrin 160°C; p4= 20% Gum Arabic 140°C; p5= 20% Gum Arabic 150°C; p6= 20% Gum Arabic 160°C; p7= 80% Maltodextrin-20% Gum Arabic 140°C; p8= 80% Maltodextrin-20%Gum Arabic 150°C; p9= 80% Maltodextrin − 20% Gum Arabic 160°C.

Ftir
The De Garmo method, which groups parameters and assigns weights or scores, was used to determine the best course of action for the final results. The weight assigned to each parameter was based on its importance or priority in influencing the results. The best treatment observed by FTIR was 20% Gum Arabic at 150°C. FTIR readings were taken in the 400–4000 cm−1 region of the mid-infrared spectrum and the results were shown in .
The FTIR results of the Jack bean tempeh hydrolyzate treated with 20% Gum Arabic at 150°C are shown in , along with a control sample without encapsulation. In the single bond stretch area between 2500–4000 cm−1, the sample absorption band was observed at wave 3270.42 cm−1 while the control absorption band was at 3271.91 cm−1. The widened wave peaks showed the presence of O-H from hydrogen bonds and carboxylic acids. In the triple bond area between 2000–2500 cm−1, there was a difference in numbers, namely the sample absorption band was observed at wave 2160.20 cm−1 while the control absorption band was at 2161.20 cm−1. The wave appeared widened showing the presence of a C≡C bond, and in the double bonds area between 1500–2000 cm−1, the sample absorption band was observed at 1625.71 cm−1 while the control absorption band was at 1623.12 cm−1. Furthermore, the waves appeared sharper downwards with narrower intensities showing the N-H bond. In the fingerprint area between 400–1500 cm−1, the sample absorption band was observed at 1032.05 cm−1 and the control absorption band was at 1039.17 cm−1. The visible wave had a strong and widened bond showing the presence of C-O. Compared to the control, the two FTIR spectra appeared similar with no significant difference in the numbers. Therefore, there was no significant structural difference between the control and the 20% Gum Arabic 150°C treatment.
Conclusion
In conclusion, the combination of maltodextrin and Gum Arabic offered a balance between EE, protection, cost, and product quality. The best Jack Bean tempeh hydrolyzate features were generated by the treatment with 20% Gum Arabic at 150°C spray drying temperature. This treatment was characterized by protein content of 47,37 ± 0.19%, EE of 96,88 ± 0.05%, and mass yield of 9,47%. Several hydrolyzate parameters were affected by encapsulation at different spray drying temperatures. This specific treatment resulted in enhanced quality and integrity of the hydrolyzate. SEM analysis of the encapsulated hydrolyzate showed particles with a distinct spherical and wrinkled morphology, signifying effective encapsulation. Furthermore, FTIR spectroscopy analysis showed no significant structural alterations between the treated samples and the uncoated control, suggesting that the encapsulation process preserved the inherent properties of the hydrolyzate. These insights provide valuable directions for the effective microencapsulation of protein hydrolyzate, underscoring the importance of selecting suitable coating materials and optimal processing temperatures.
Acknowledgments
The author is grateful to the Rector of Universitas Padjadjaran for Riset Disertasi Doktor UNPAD (RDDU) with research grant 1932/UN6.N/LT/2019.
Disclosure statement
The author(s) did not report any potential conflicts of interest and This article does not include any additional data or supplementary materials.
Additional information
Funding
References
- Roni, K. A. The Effect of Adding Pineapple Peel and Core Juice in the Tempeh Making Process. Berk Tek. 2013, 3(2), 573–585. https://jurnal.um-palembang.ac.id/index.php/berkalateknik/article/view/362/0.
- Nurhaeni; Kencana, F. S.; Ajeng, A. T.; Khairuddin; Prismawiryanti; Syamsuddin. Manufacturing of Tofu Pulp Protein Concentrate Using Ammonium Sulphate. KOVALEN J. Ris. Kim. 2022, 8(1), 67–73. DOI: 10.22487/kovalen.2022.v8.i1.15844.
- Sarabandi, K.; Gharehbeglou, P.; Jafari, S. M. Spray-Drying Encapsulation of Protein Hydrolysates and Bioactive Peptides: Opportunities and Challenges. Dry. Technol. 2019, 38(5–6), 577–595. DOI: 10.1080/07373937.2019.1689399.
- Liu, Y.; Cao, A. Encapsulating Proteins in Nanoparticles: Batch by Batch or One by One. 1stVol. 590, Methods in Enzymology. Elsevier Inc.; 2017pp. 1–3110.1016/bs.mie.2016.12.001.
- Nguyen, P. M. Combination of Maltodextrin and Gum Arabic as Wall Materials in Proficiency of Microencapsulated Durian. Biosci. Res. 2020, 17(3), 2127–2133.
- Rosland Abel, S. E.; Yusof, Y. A.; Chin, N. L.; Chang, L. S.; Mohd Ghazali, H.; Manaf, Y. N. Characterisation of Physicochemical Properties of Gum Arabic Powder at Various Particle Sizes. Food Res. 2020, 4(S1), 107–115. DOI: 10.26656/fr.2017.4(S1).S32.
- Sun, X.; Cameron, R. G.; Bai, J. Effect of Spray-Drying Temperature on Physicochemical, Antioxidant and Antimicrobial Properties of Pectin/Sodium Alginate Microencapsulated Carvacrol. Food Hydrocoll. 2020, 100(August 2019), 1–10. DOI: 10.1016/j.foodhyd.2019.105420.
- Romulo, A.; Surya, R. Tempe: A Traditional Fermented Food of Indonesia and Its Health Benefits. Int. J. Gastron. Food Sci. 2021, 26(December 2021), 100413. DOI: 10.1016/j.ijgfs.2021.100413.
- Burger, T. G.; Singh, I.; Mayfield, C.; Baumert, J. L.; Zhang, Y. The Impact of Spray Drying Conditions on the Physicochemical and Emulsification Properties of Pea Protein Isolate. LWT. 2022, 153(September 2021), 112495. DOI: 10.1016/j.lwt.2021.112495.
- Fitriyani, E.; Devina, A.; Aulia, L.; Annisa, S. Microencapsulation of Garlic Oil with Gelatin and Maltodextrin Encapsulant Using the Coacervation Method. Equilib. J. Chem. Eng. 2023, 7(1), 47–52. DOI: 10.20961/equilibrium.v7i1.64248.
- Kumari, A.; Kaushik, N.; Slizyte, R.; Khushboo. Production and Microencapsulation of Protein Hydrolysate of Pink Perch (Nemipterus Japonicus) by ‑ Products Obtained from Surimi Industry for Its Sustainable Utilization. Waste Biomass. Valor. 2022, 14(1), 209–226. Published online. DOI: 10.1007/s12649-022-01853-3.
- Pratiwi, D. I.; Purwantini, A. Production of Chitosan from Fish Scale Waste (Temperature Extraction and NaOH Volume Variables). J. Inov. Proses. 2021, 6(1), 13–19.
- de Bittencourt, L. L. A.; Pedrosa, C.; Sousa, V. P. D.; Pierucci, A. P. T.; Citelli, M. Pea Protein Provides a Promising Matrix for Microencapsulating Iron. Plant Foods Hum. Nutr. 2014, 68(4), 333–339. DOI: 10.1007/s11130-013-0383-8.
- Andrade, J.; Pereira, C. G.; de Almeida Junior, J. C.; Viana, C. C. R.; de Neves, L. N. O.; Silva da, P. H. F.; de Anjos, V. C. FTIR-ATR Determination of Protein Content to Evaluate Whey Protein Concentrate Adulteration. LWT. 2019, 99, 166–172. DOI: 10.1016/j.lwt.2018.09.079.
- Rasyid, R. P.; Suprayitno, E.; Sulistiyati, T. D. The Effect of Addition of Arabic Gum to Amino Acid Profile and Fatty Acid Profile of Albumin Powder Cork Fisk (Channa Striata). Int. J. Sci. Res. Publ. 2019, 9(5), 657–662. DOI: 10.29322/ijsrp.9.05.2019.p8979.
- Susianti; Amalia, U.; Rianingsih, L. Addition of Arabic Gum with Different Concentrations to the Content of Volatile Compounds Rusip Anchovy Powder (Stolephorus sp.). J. Ilmu. dan Teknol Perikan. 2020, 2(1), 10–19. DOI: 10.14710/jitpi.2020.8083.
- Ibanoǧlu, E. Rheological Behaviour of Whey Protein Stabilized Emulsions in the Presence of Gum Arabic. J. Food Eng. 2002, 52(3), 273–277. DOI: 10.1016/S0260-8774(01)00115-7.
- Karrar, E.; Mahdi, A. A.; Sheth, S.; Ahmed, I. A. M.; Manzoor, M.; Wei, W.; Wang, X. Effect of Maltodextrin Combination with Gum Arabic and Whey Protein Isolate on the Microencapsulation of Gurum Seed Oil Using a Spray-Drying Method. Int J Biol Macromol. 2020, 171, 1–35. Published online. DOI: 10.1016/j.ijbiomac.2020.12.045.
- Abidin, A. F.; Yuwono, S. S.; Maligan, J. M. The Effect of Maltodextrin and Egg White on Characteristic of White Oyster Mushroom Broth Powder. J. Pangan. dan Agroindustr. 2019, 7(4), 53–61. DOI: 10.21776/ub.jpa.2019.007.04.6.
- Emmawati, A.; Salman, S.; Rachmawati, M. The Effect of Temperature and Drying Time on the Chemical Characteristics of Yogurt Chips Durian (Durio Zibethinus) Aswita. J. Trop. AgriFood. 2022, 3(2), 86–92. DOI: 10.35941/jtaf.3.2.2021.6199.86-92.
- Hassani, A.; Azarian, M. M. S.; Ibrahim, W. N.; Hussain, S. A. Preparation, Characterization and Therapeutic Properties of Gum Arabic-Stabilized Gallic Acid Nanoparticles. Sci. Rep. 2020, 10(1), 1–18. DOI: 10.1038/s41598-020-71175-8.
- Mehrad, B.; Shabanpour, B.; Jafari, S. M.; Pourashouri, P. Characterization of Dried Fish Oil from Menhaden Encapsulated by Spray Drying. AACL. Bioflux. 2015, 8(1), 57–69.
- Premi, M.; Sharma, H. K. Effect of Different Combinations of Maltodextrin, Gum Arabic and Whey Protein Concentrate on the Encapsulation Behavior and Oxidative Stability of Spray Dried Drumstick (Moringa Oleifera) Oil. Int J Biol Macromol. 2017, 105, 1232–1240. DOI: 10.1016/j.ijbiomac.2017.07.160.
- Akdeniz, B.; Sumnu, G.; Sahin, S. The Effects of Maltodextrin and Gum Arabic on Encapsulation of Onion Skin Phenolic Compounds. Chem. Eng. Trans. 2017, 57, 1891–1896. DOI: 10.3303/CET1757316.
- Kunarto, B.; Iswoyo, I. Nanoencapsulation of Red Melinjo Peel Extract (Gnetum Gnemon L.) at Various Inlet Temperatures and Flow Rates of the Spray Dryer. J. Teknol. Pertan. 2021, 22(3), 211–220. DOI: 10.21776/ub.jtp.2021.022.03.6.
- Arepally, D.; Goswami, T. K. Effect of Inlet Air Temperature and Gum Arabic Concentration on Encapsulation of Probiotics by Spray Drying. LWT. 2019, 99(May 2018), 583–593. DOI: 10.1016/j.lwt.2018.10.022.
- Mahdi, A. A.; Mohammed, J. K.; Al-Ansi, W.; Ghaleb, A. D. S.; Al-Maqtari, Q. A.; Ma, M. Microencapsulation of Fingered Citron Extract with Gum Arabic, Modified Starch, Whey Protein, and Maltodextrin Using Spray Drying. Int J Biol Macromol. 2020, 152, 1125–1134. DOI: 10.1016/j.ijbiomac.2019.10.201.
- Purnamayati, L.; Dewi, E. N.; Kurniasih, R. A. Phycocyanin Stability in Microcapsules Processed by Spray Drying Method Using Different Inlet Temperature. IOP Conf. Ser. Earth Environ. Sci. 2018, 116(1), 1–6. DOI: 10.1088/1755-1315/116/1/012076.
- Dewi, A. K.; Satibi, L. Study of the Effect of Spray Drying Temperature on Drying Time and Yield of Coconut Milk Powder. Konversi. 2015, 4(1), 25–31.
- Mohammed, N. K.; Tan, C. P.; Manap, Y. A.; Muhialdin, B. J.; Hussin, A. S. M. Spray Drying for the Encapsulation of Oils—A Review. Molecules. 2020, 25(17), 1–16. DOI: 10.3390/molecules25173873.
- Al-Ismail, K. M.; Mehyar, G.; Al-Khatib, H. S.; Al-Dabbas, M. Effect of Microencapsulation of Cardamom’s Essential Oil in Gum Arabic and Whey Protein Isolate Using Spray Drying on Its Stability During Storage. Qual. Assur. Saf. Crop Foods. 2015, 7(5), 613–620. DOI: 10.3920/QAS2014.0422.
- Wang, F.; Mutukumira, A. N. Microencapsulation of Limosilactobacillus reuteri DPC16 by Spray Drying Using Different Encapsulation Wall Materials. J. Food Process Preserv. 2022, 46(10), 1–9. DOI: 10.1111/jfpp.16880.
- Hasna, T.; Anandito, B. K.; Khasanah, L. U.; Utami, R.; Manuhara, G. J. Effect of Maltodextrin and Whey Combination as Wall Material on the Characteristics of Cinnamon (Cinnamomum Burmanii) Oleoresin Microencapsule Tanalyna. agriTECH. 2019, 38(3), 259–264. DOI: 10.22146/agritech.12725.
- Correâ-Filho, L. C.; Lourenço, M. M.; Moldaõ-Martins, M.; Alves, V. D. Microencapsulation of β-Carotene by Spray Drying: Effect of Wall Material Concentration and Drying Inlet Temperature. Int. J. Food Sci. 2019, 2019, 1–12. DOI: 10.1155/2019/8914852.