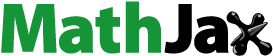
ABSTRACT
Brown rice and whole grains are vital nutrient sources for humans; however, they usually require a long cooking period and are difficult to cook uniformly. This study investigated electrical conductivities of brown rice (KDML 105, Riceberry, and red cargo rice) and whole grains (lotus seed, red kidney bean, and Job’s tear) under various conditions. Furthermore, ohmic heating was applied in cooking the mixtures of brown rice and whole grains and the results were compared with conventional heating. The key objective was to apply ohmic heating in cooking the mixtures of brown rice and whole grains to improve the retention of nutrients in cooked products and to improve process efficiency. It appeared that electrical conductivities of all samples were sufficiently high for applying ohmic heating. Electrical conductivities of lotus seed mixed with water were between 0.061 and 0.402 S/m at 30–100°C that were higher than red kidney bean (0.016–0.256 S/m) and Job’s tears (0.018–0.148 S/m). Brown rice of Riceberry variety had the highest electrical conductivities (0.067–0.204 S/m) followed by red cargo rice (0.067–0.173 S/m) and KDML 105 (0.048–0.130 S/m). Sample mixtures cooked using ohmic method had significantly higher antioxidant activities and the calcium, phosphorus, and potassium contents than those cooked using conventional method. Precooking steps for lotus seed, red kidney bean, and Job’s tears were needed with the conventional cooking approach; however, these were unnecessary with ohmic cooking. Ohmic cooking apparatus in this study consumed approximately 2% more energy than electric rice cooker.
Introduction
Brown rice is a beneficial component of a healthy diet for individuals looking to manage obesity and reduce the risk of chronic diet-related diseases. Brown rice has higher fiber and nutrients and a lower glycemic index compared to white rice.[Citation1] However, consuming only brown rice cannot provide sufficient nutrition. Therefore, it is recommended to supplement brown rice with whole grains to ensure a balanced and nutritious meal. Some kinds of whole grains, such as lotus seed, red kidney bean, and Job’s tear, offer abundant nutritional benefits and these grains are broadly recognized for their rich nutrient profiles and health-promoting properties.[Citation2] Lotus seed is a good source of protein, fiber, and essential minerals, such as magnesium and potassium.[Citation3] Red kidney bean contains high protein, dietary fiber, iron, and antioxidants.[Citation4] Job’s tear, also known as coix seed, is rich in dietary fiber, antioxidants, and minerals, such as calcium and phosphorus.[Citation5] Including these ingredients in the brown rice meals provides an interesting approach to enhance nutritional value and health benefits.
In general, mixtures of brown rice and whole grains are prepared using a rice cooker or stove. These cooking methods rely on conventional heating mechanisms, namely heat conduction and convection, which typically require long cooking periods leading to nutrient loss compared to the raw brown rice and whole grains. In addition, different sizes and heating characteristics between brown rice and each type of whole grain lead to non-uniformity of cooking levels among different compositions. Furthermore, a rice cooker would automatically shift from cooking mode to the warming mode when reaching the set temperature; as a result, the whole grains having a larger size than rice tend to be undercooked. Therefore, an innovation is required that can shorten the cooking process and provide superior cooked brown rice and whole grain mixture.
Ohmic heating is an alternative method to conventional heating that has potential to be applied in cooking a mixture of brown rice and whole grains. This technique involves generating heat directly inside the food material by passing an electrical current through it.[Citation6] The amount of heat produced depends on the quantity of electrical power, which, in turn, is related to the applied voltage gradient and the electrical conductivity of the food material. Studies[Citation7–12] have reported the application of ohmic heating in rice cooking, resulting in significantly different properties for the cooked rice compared to the conventional method. Variation in the quality of rice cooked using ohmic and conventional methods depends on the type and variety of rice sample.[Citation8,Citation9] Furthermore, ohmic cooking systems usually have a noticeable reduction in energy consumption compared to conventional rice cookers.[Citation9–11] However, ohmic heating is constrained by some factors like electrical conductivity, electrical field distribution, fouling deposits on electrodes, equipment design, and cost. To date, only limited information has been published regarding the application of ohmic heating to cook brown rice mixed with whole grains, which is more complicated than cooking brown rice alone. More information that is scientific are still needed for the successful utilization of ohmic heating technology for this kind of food product. In order to fulfill this research gap, in the present study, the brown rice of three varieties (KDML 105, Riceberry, and red cargo rice) and three kinds of whole grains (lotus seeds, red kidney beans, and Job’s tears) were measured for electrical conductivity and cooked using ohmic method. The objectives were: (1) to investigate the possibility of applying ohmic heating for cooking brown rice mixed with whole grains; and (2) to compare the total phenolic content, antioxidant activities, vitamin B1, some minerals, and energy consumption between cooking products by ohmic and conventional methods.
Materials and methods
Raw materials
The raw materials consisted of brown rice of three varieties: KDML 105, Riceberry, and red cargo rice. These rice varieties have exceptional characteristics. KDML 105 is famous for its unique aroma, flavor, and slightly sticky texture when cooked. Riceberry and red cargo rice are well-known for their high levels of antioxidants due to the anthocyanin and proanthocyanidins contained in their bran. They are also rich in various vitamins and minerals, especially zinc and iron. These brown rice samples were purchased from a supermarket in Thailand. All brown rice samples were placed in polyethylene plastic bags and stored in a refrigerator at around 2–4°C to retard rancidity. Three types of whole grains comprising lotus (Nelumbo nucifera) seed, red kidney bean (Phaseolus vulgaris), and glutinous-type Job’s tear (Coix lacryma-jobi) were selected for this study due to their nutrient contents and health benefits, as previously mentioned. The lotus seed was supplied by Kopongkimyong shop, Kim Yong Market, Songkhla, Thailand while the red kidney bean and Job’s tear were manufactured by Thanya Farm Co., Ltd., Nonthaburi, Thailand. The varieties of all these samples were not declared by the manufacturers. All the whole grain samples were placed in separate polyethylene plastic bags and stored at room temperature.
Electrical conductivity measurement
The electrical conductivity of each sample of the mixtures of all brown rice varieties and whole grains was measured under the different preparation processes as categorized into three cases.
Case 1 lotus seed, red kidney bean, and job’s tears
Each whole grain was soaked in a sodium chloride solution at a concentration of 0.005 M with a grain-to-sodium chloride solution ratio of 1:3 by weight for 12 h to let the whole grain absorb moisture and to increase the electrical conductivity of the whole grain. After that, the sodium chloride solution was drained and replaced with distilled water at a grain-to-distilled water ratio of 1:3 by weight. Notably, the sodium chloride solution was drained to remove some microorganisms that might have grown during the long soaking period. The electrical conductivity or each sample was measured in a temperature range of 30–100°C. A magnetic stirrer was operated during the electrical conductivity measurement to ensure proper mixing. The data on temperature, electrical current, and voltage were recorded every 1 minute.
Case 2 brown rice
Brown rice of each variety was soaked in a sodium chloride solution at a concentration of 0.005 M with a rice-to-sodium chloride solution ratio of 1:3 by weight for 30 minutes. The soaking period of brown rice was much shorter than that for the lotus seed, red kidney bean, and Job’s tears because of their different sizes and moisture absorption characteristics. It must be noted that the soaking time selected for the whole grains (12 h) was specified from the preliminary study whereas that of the brown rice samples (30 minutes) was recommended in the cooking guideline of the brown rice product manufacturer. The brown rice, together with the soaking solution, was used for measuring the electrical conductivity in a temperature range of 30–100°C. Similar to the lotus seed, red kidney bean, and Job’s tears, the samples were stirred using a magnetic stirrer during the electrical conductivity measurement.
Case 3 combinations of lotus seed, red kidney bean, job’s tears, and brown rice
First, the whole grains of lotus seed, red kidney bean, and Job’s tears were soaked in a 0.005 M sodium chloride solution with a grain-to-sodium chloride solution ratio of 1:3 by weight for 12 h. After finishing the soaking period, the sodium chloride solution was drained. The soaked lotus seed, red kidney bean, and Job’s tears were mixed with the soaked brown rice with a whole grains-to-brown rice ratio of 1:9 by weight. For soaking, the brown rice samples of KDML 105, Riceberry, and red cargo rice were blended at three different ratios (1:1:1, 1:2:1, and 1:1:2 by weight) prior to steeping in a 0.005 M sodium chloride solution for 30 minutes. The combination of soaked lotus seed, red kidney bean, Job’s tears, and brown rice was covered with the sodium chloride solution that was formerly used in the brown rice soaking process at a soaked grains-to-solution ratio of 1:3 by weight. The combinations of lotus seed, red kidney bean, Job’s tears, and brown rice, together with the soaking solution, were measured for their electrical conductivities in a temperature range of 30–100°C. A magnetic stirrer was applied during the electrical conductivity measurement.
The electrical conductivity values of all samples were examined using a small ohmic heating system, as shown in . The system consisted of a cylindrical ohmic cell made from acrylic pipe with an inside diameter of 0.045 m. The electrodes were made of stainless-steel grade 316 L with an identical diameter size to the inside diameter of ohmic cell and were located at both ends of the ohmic cell, with a gap of 0.09 m between the electrodes. During measurement, the ohmic cell was placed on a magnetic hot plate stirrer (model C-MAG HS 4; IKA Corporation; NC, USA), with a magnetic bar rotating inside the cell to ensure a uniform sample temperature, with the heating mode of the magnetic hot plate stirrer turned off. The electrical field strength applied in the measurement was in the range 13.3–44.4 V/cm at a frequency of 50 Hz. A type-T thermocouple was positioned at the center of the ohmic cell to monitor the sample temperature. The temperature data were recorded at 1 minute intervals using a data logger (model DX 1012; Yokogawa Electric Corporation; Tokyo, Japan). The applied voltage and current were measured using a digital multimeter (model 8808A; Fluke Corporation; WA, USA). The experiments were conducted in triplicate. The electrical conductivity of each sample was calculated using EquationEquation (1)(1)
(1) :
Figure 1. The schematic diagram of the small ohmic heating circuit used for measuring electrical conductivity.

where is the electrical conductivity (S/m),
is the cross-sectional area of the electrode (m2),
is the electrical current (A),
is the distance between the electrodes (m), and
is the applied voltage (V).
Development of empirical models for predicting electrical conductivity of samples
The experimental data from the electrical conductivity measurements were fitted to a linear model to represent the relationship between the electrical conductivity of a sample (solid-liquid phase mixture) and temperature. The models developed in the present study was used to estimate the electrical conductivities of samples during the ohmic heating process.
Cooking of mixtures of brown rice and whole grains
Conventional method
First, the whole grains of lotus seed, red kidney bean, and Job’s tears were separately soaked in a 0.005 M sodium chloride solution with a grain-to-sodium chloride solution ratio of 1:3 by weight for 12 h. After soaking, the sodium chloride solution was drained and replaced with distilled water. Then, the samples were precooked before being combined with the brown rice. Notably, the precooking was conducted by placing the sample in boiling water for 15 minutes for the lotus seed and the Job’s tears and for 17 minutes and 30 seconds for the red kidney bean. The precooking step for the whole grains was necessary because the whole grains needed a much longer cooking time in the electric rice cooker than the brown rice. These precooking times were determined in the preliminary study. They provided the partly-cooked whole grains after precooking step and the well-cooked samples with good characteristics at the end of cooking process by the electric rice cooker. The brown rice samples were blended at KDML 105-to-Riceberry-to-red cargo rice ratios of 1:1:1, 1:2:1, and 1:1:2 by weight before soaking in the sodium chloride solution at the same concentration and grain-to-sodium chloride solution ratio for 30 minutes. The precooked whole grains were mixed with the soaked brown rice with a whole grains-to-brown rice ratio of 1:9 by weight. The combination of soaked lotus seed, red kidney bean, Job’s tears, and brown rice was covered with the sodium chloride solution that had been used in the brown rice soaking process at a soaked grains-to-solution ratio of 1:3 by weight. The size of sample mixture for each cooking batch was 600 g. An electric rice cooker (model CR-110 T; OTTO Kingglass Co., Ltd.; Bangkok, Thailand) was used to cook the samples. During the cooking process, the temperature was monitored using a type-T thermocouple probe placed in the liquid at the midpoint of the sample mixture. The sample was heated from around 30°C to 100°C during the cooking mode of the electric rice cooker before switching to the warm mode to maintain the temperature at 100.0 ± 0.5°C for 10 minutes. The conventional cooking experiments were conducted in three replicates.
Ohmic heating method
The samples were prepared applying the identical procedure to that of the conventional cooking method, except that the lotus seed, red kidney bean, and Job’s tears were not precooked after soaking. These whole grains were not precooked because the ohmic heating method, due to its method of heating, could heat the mixtures of whole grains, brown rice, and solution simultaneously and more uniformly than the conventional cooking method. The sample size for each cooking batch was same as that for the conventional method. The schematic diagram of the ohmic heating system used for cooking is depicted in . The ohmic cell of cubic shape with 100 mm dimensions was constructed using Teflon plate material with 10 mm thickness. Titanium grade Gr-2 electrodes (5 mm thickness) were located at both the left and right walls of the ohmic cell, with a gap of 80 mm between them. An agitator was mounted on the cover of the ohmic cell to stir the sample during cooking. The agitator was directly driven using a gear motor with an electrical voltage of 12 V DC and a maximum current of 3 A. The agitator shaft and the paddle were made of Teflon. During the cooking process, the sample temperatures were measured and recorded using a type-T thermocouple linked to a data logger. The temperature profiles of the samples during ohmic cooking were controlled to be analogous to those of the conventional cooking by manually regulating the applied electrical voltage supplied from the variable-voltage transformer. The transformers in the ohmic cooking system consisted of a variable transformer with input/output voltages of 220 V AC/0–250 V AC, a power capacity of 5 KVA, and a maximum electric current of 20 A (model TSGC2–5 K; Stable Transformer Co., Ltd.; Pathum Thani, Thailand), and an invariable transformer with input/output voltages of 220 V AC/350 V AC, a power capacity of 7 KVA, and a maximum electric current of 20 A (model STB 301; Stable Transformer Co., Ltd.; Pathum Thani, Thailand). The ohmic cooking experiments were conducted in three replicates.
Quality determination
The mixtures of brown rice and whole grains using different KDML 105-to-Riceberry-to-red cargo rice ratios were examined for their total phenolic contents and antioxidant activities after cooking whereas the vitamin B1, calcium, phosphorus, and potassium contents were determined only for the cooked samples using the ratio of KDML 105-to-Riceberry-to-red cargo rice of 1:2:1 since this condition provided the cooked samples with the significantly higher total phenolic contents and antioxidant activities than their counterparts.
Total phenolic content
The cooked samples were determined for their total phenolic contents by applying Folin-Ciocalteu reagent according to the method modified from Wolfe et al.[Citation13] For measurements, gallic acid was used as the standard for all phenolic compounds. A standard curve was created at concentrations of gallic acid between 10 and 50 µM. A methanolic extract sample (0.2 ml) was diluted to the concentration range of the standard curve prior to adding 0.2 ml of Folin-Ciocalteu solution. After 6 min, 2 ml of saturated sodium carbonate solution was added and thoroughly mixed. Then, the sample was stored for 90 minutes in the dark at room temperature before measuring its absorbance at a wavelength of 750 nm using a UV-visible spectrophotometer (Genesys 10S; Thermo Fisher Scientific; Waltham, MA, USA). The total phenolic content values of the samples were calculated and presented as milligrams of gallic acid equivalent per gram of dry sample (mg GAE/gdry sample). The total phenolic content was determined for three replicates of each sample.
Antioxidant activity
The antioxidant activity values of cooked samples were determined based on the scavenging activity of the stable 1,1-diphenyl-2-picrylhydrazyl (DPPH) free radical, using the method modified from Tachibana et al.[Citation14] Trolox was used as an external standard to generate the standard curve, with its concentrations ranging from 10 to 50 µM. For the sample analysis, 1 ml of the methanolic extract sample was diluted to the concentration range of the standard curve before adding 1 ml of DPPH (dissolved in 95% methanol) at a concentration of 200 µM. The mixture was mixed and then kept in the dark at room temperature for 30 minutes prior to measuring the absorbance at a wavelength of 515 nm using the UV-visible spectrophotometer. The results were reported in milligrams of Trolox per gram of dry sample to quantify the antioxidant activity. The antioxidant activity was determined for three replicates of each sample.
Vitamin B1, calcium, phosphorus, and potassium
The Vitamin B1 (thiamin), calcium, phosphorus, and potassium contents of the cooked samples were determined using AOAC method no. 942.23 for vitamin B1 and no. 984.27 for calcium, phosphorus, and potassium.[Citation15,Citation16]
Energy consumption
The energy consumption values for cooking the mixtures of brown rice and whole grains using the conventional and ohmic cooking methods were measured using a digital Watt-hour meter (model MPH-W1-29A-0NNNB; Axe Technology Corporation; Taiwan).
Statistical analysis
Statistical analysis was conducted using the SPSS software package (version 16.0; IBM Inc.; Chicago, IL, USA). Model fitting was performed based on the least square method. Additionally, the software was utilized for analysis of variance and Duncan’s multiple range testing to compare means and to identify significant differences among groups at the p ≤ .05 level.
Results and discussion
Electrical conductivity of samples
Case 1 lotus seed, red kidney bean, and job’s tears
The results of electrical conductivity measurement for the lotus seed, red kidney bean, and Job’s tears are presented in , with lotus seed mixed with water having the highest values, in the range 0.061–0.402 S/m at temperatures in the range 30–100°C, followed by red kidney bean with water (0.016–0.256 S/m) and Job’s tears with water (0.018–0.148 S/m). Although all three of these samples had low electrical conductivity values, especially at 30°C, the values were sufficiently high for ohmic heating. Piette et al.[Citation17] pointed out that food materials could be efficiently heated using the ohmic method if their electrical conductivity fell in the range 0.01–10 S/m. The electrical conductivity values of these three samples were low chiefly due to the fact that the sodium chloride solution that was applied for soaking the whole grain was drained and replaced with distilled water which is non-conductive for electricity. The electrical-conductive constituents contained in the whole grains during soaking would be diluted by the distilled water. In addition, the results demonstrated linear relationships for all samples between electrical conductivity and temperature, which were consistent with other published research, such as Icier and Ilicali[Citation18] and Tumpanuvatr & Jittanit.[Citation8,Citation19] Typically, the electrical conductivity values of food materials are strongly related to their ionic content, ionic movement, and physical structure.[Citation20] Thus, the electrical conductivity of a sample increased with temperature, since ions in the sample moved more rapidly at a higher temperature. The electrical conductivity values for the lotus seed, red kidney bean, and Job’s tears were different because of their dissimilarities in composition, physical structure, moisture content, and sodium chloride absorptivity. Tulsiyan et al.[Citation21] reported significant differences in electrical conductivity among various components in their multicomponent food systems, with sauces having the highest electrical conductivity, followed by vegetables and chicken. They claimed that the differences in electrical conductivity could be attributed to the varying composition of the food components. For example, bean sprouts and shiitake mushrooms had low electrical conductivity values due to air cavities in their internal structures. Chicken had a lower conductivity attributed to its non-conductive fat content. However, the inclusion of salted soy sauce in their multicomponent food systems enhanced the ion contents and subsequently elevated their electrical conductivities.
Case 2 brown rice
The electrical conductivity values of the brown rice samples mixed with sodium chloride solution are presented in . The brown rice of the Riceberry variety had the highest electrical conductivity, in the range 0.067–0.204 S/m, followed by the red cargo rice and KDML 105 varieties, with their electrical conductivity ranges being 0.067–0.173 and 0.048–0.130 S/m, respectively. Similar to the lotus seed, red kidney bean, and Job’s tears, these brown rice samples had adequately high electrical conductivity values for ohmic heating. In addition, these results were consistent with the research conducted by Tumpanuvatr & Jittanit,[Citation8] who reported that the electrical conductivities of four varieties of brown rice, mixed with 0.005 M sodium chloride solutions at different rice-to-sodium chloride solution ratios depending on the rice variety, were in the range 0.05–0.25 S/m. The Riceberry sample had a higher electrical conductivity than the other two rice varieties, most likely due to its greater contents of phytochemical components and minerals, as reported by Chiang et al.[Citation22] These phytochemical components and minerals contributed to an increased presence of ions in the mixture sample. Similar to the previous section, there was an increase in the electrical conductivity for the brown rice samples with temperature. However, after reaching temperatures in the range 60–80°C, there was a noticeably slower increase in electrical conductivity that could be attributed to starch gelatinization in the brown rice during measurement, as according to Juliano & Bechtel[Citation23] and Roy et al.,[Citation24] starch gelatinization in rice typically occurs at temperatures in the range 55–79°C, depending on the variety of rice. Starch gelatinization could result in a slower increase in the electrical conductivity of a sample because the starch granules adsorbed water and became swollen when gelatinized, leading to a reduction in the amount of free water. In addition, a larger starch granule size reduces the gap between granules; consequently, there is diminished space for movement of ionic particles in the sample system.[Citation9,Citation25] Nonetheless, Li et al.[Citation25] reported that the electrical conductivity value of starch would rapidly rise once more with an the increase in temperatures after the end of the gelatinization process.
Case 3 combinations of lotus seed, red kidney bean, job’s tears, and brown rice
The combinations of soaked lotus seed, red kidney bean, Job’s tears, and brown rice mixed with sodium chloride solution were determined for their electrical conductivity values and the results are illustrated in . The blend of KDML 105, Riceberry, and red cargo brown rice at the ratio of 1:2:1 had the highest electrical conductivity compared to those for 1:1:1 and 1:1:2. The electrical conductivity values in the blended brown rice samples at the ratios of 1:2:1, 1:1:2, and 1:1:1 were in the ranges 0.079–0.286, 0.077–0.274, and 0.056–0.265 S/m, respectively. It was not surprising that a greater proportion of Riceberry brown rice resulted in a higher electrical conductivity because of its higher content of ionic constituents, as described in the previous section. The combination of various grains caused the disparity in electrical conductivity values within the mixtures due to the different electrical conductivities of the grains. These variations could result in dissimilar ohmic heating rates across different regions in the mixture, especially if no agitator was used during ohmic heating. The discrepancies in the electrical conductivity values of the various grains within the mixture that led to the non-uniformity of ohmic heating rates could also have been due to the electrical conductivity difference between the grains and the surrounding solution, resulting in a non-uniform temperature distribution in the mixture.
Figure 5. Electrical conductivities of the whole grain and brown rice in case 3 (▲, 1:1:1 (KDML 105:Riceberry:Red cargo rice) experiment; ●, 1:2:1 (KDML 105:Riceberry:Red cargo rice) experiment; ■, 1:1:2 (KDML 105:Riceberry:Red cargo rice) experiment; —, 1:1:1 (KDML 105:Riceberry:Red cargo rice) model prediction; ‐‐‐‐, 1:2:1 (KDML 105:Riceberry:Red cargo rice) model prediction; ⋅⋅⋅⋅⋅, 1:1:2 (KDML 105:Riceberry:Red cargo rice) model prediction).

Empirical models for predicting electrical conductivity of samples
The results of fitting the experimental data obtained from the electrical conductivity measurements in the previous section to linear models are shown in . These models represent the relationships between the electrical conductivities of samples and temperatures and can be applied for estimating the electrical conductivities of samples during the ohmic heating process. It appeared that the values for the coefficient of determination (R2) of these equations were high while the values for root mean square error (RMSE) were low, indicating good fits to the respective experimental data. The comparisons between the experimental data and model predictions are illustrated in .
Table 1. Empirical equations representing correlations between electrical conductivities of samples and temperatures.
Cooking of mixtures of brown rice and whole grains
The temperature profiles of the combination of soaked lotus seed, red kidney bean, Job’s tears, brown rice, and sodium chloride solution during cooking using conventional and ohmic methods were controlled to be similar, as illustrated in . The samples started boiling at around 100°C after cooking for approximately 14 minutes, whereas the total cooking time for both the cooking and warming modes was around 48 minutes. Notably, the lotus seed, red kidney bean, and Job’s tears were precooked in boiling water before mixing them with the brown rice and sodium chloride solution and cooking in the electric rice cooker; otherwise, these whole grains would not be well-cooked. The whole grains required a much longer cooking time in the electric rice cooker than the brown rice because of their larger particle sizes (≥8 times), dissimilar compositions (especially in the skin layers), and gelatinization temperatures. The gelatinization temperature of red kidney bean was reported to be in the range 63.62–81.72°C,[Citation26] while being greater than 70°C for lotus seeds[Citation27] and in the range 68.28–80.94°C for white Job’s tear starch.[Citation28] Tumpanuvatr et al.[Citation29] reported that the gelatinization temperature of brown rice of the KDML 105 variety was in the range 62.90–77.62°C, whereas Kraithong et al.[Citation30] reported it was in the range 69.57–82.41°C for Riceberry flour. The longer cooking time required for the whole grains compared to the brown rice typically is problematic in the preparation and cooking processes of these whole grain mixtures. For example, in the current study, the lotus seed, Job’s tears, and red kidney bean had to be precooked in boiling water for 15, 15, and 17.5 minutes, respectively, before mixing with the soaked brown rice and cooking in an the electric rice cooker. The current application of ohmic heating for cooking these mixtures overcame this issue because this method generated internal heat that differed from the conventional heat transfer mechanisms (conduction and convection) that can be retarded by low thermal conductivity of food grain and a larger grain size, together with the low convective heat transfer coefficients between the surrounding liquid and the grains. In addition, the applied electric field during ohmic heating typically causes the formation of pores in cell membranes which is known as electroporation,[Citation31] which can facilitate moisture diffusion via the grain surface into the internal structure, resulting in the grains being cooked more rapidly than using the conventional cooking method. With ohmic heating, the soaked lotus seed, red kidney bean, and Job’s tears were not precooked before mixing with the soaked brown rice; nonetheless, the mixtures were well-cooked after ohmic cooking for approximately 48 minutes. In the current study, the well-cooked grains were detected using two steps: (1) visual inspection, involving examination of the grains for their plumpness, tenderness, and center color (when fully cooked, they would not have any opaque spots at the center of grains)[Citation32–34]; and (2) taste, involving evaluation of their textural characteristics (not overly starchy and not having a grainy texture). The results from this part of the current study were notable because they indicated an apparent advantage of ohmic heating in this cooking application.
Figure 6. Temperature profiles of the combination of soaked lotus seed, red kidney bean, job’s tears, brown rice (KDML 105:Riceberry:Red Cargo rice = 1:1:1), and sodium chloride solution during cooking; ■, conventional method; ×, ohmic method.

Antioxidant activity and nutritional quality
Total phenolic content and antioxidant activity
The results of the total phenolic content and antioxidant activity determinations for the cooked samples of the different whole grains and brown rice are presented in . It was clear that increasing either the Riceberry or red cargo rice proportions in the mixture increased the total phenolic content and antioxidant activity values; however, the increases were greater for the Riceberry because the brown rice Riceberry variety has components rich in antioxidants, such as vitamin E (tocotrienol), anthocyanins (cyanidin-3-glucoside and peonidin-3-glucoside), and phytosterols (gamma oryzanol, beta-sitosterol, and triterpenes).[Citation35] Comparing the conventional and ohmic cooking methods, there was no significant difference between the cooked samples of the same combination of grains in their total phenolic contents, except for the combination of KDML 105:Riceberry:red cargo rice of 1:2:1. The ohmic heating method produced a higher total phenolic content compared to the conventional method for this ratio probably because it contained twice the amount of Riceberry compared to the other varieties of brown rice, which increased the amount of total phenolic compounds in the sample mixture. These phenolic compounds were dissolved into the surrounding liquid during heating and then absorbed into the grain structure during starch gelatinization. In addition, electroporation occurring during ohmic heating allowed the electrical charges to build up and form pores across cell walls resulting in the greater amount of phenolic compounds that could be absorbed in the grain microstructure. Compounds within the grain structure would be more tolerant to heat damage and oxidation than those on the surface and in the surrounding liquid. Therefore, the remaining phenolic compounds in the ohmically cooked sample were significantly higher than those conventionally cooked samples in this case. Aamir and Jittanit[Citation36] pointed out that the electroporation was responsible for breakdown of cell membranes during ohmic heating, which enabled the movement of bioactive compounds across cell membrane of Gac aril such as β-carotene and lycopene much easier when compared to the conventional heating. They also showed the scanning electron micrograph of the Gac aril powder residue obtained from ohmic heating treatment which was more porous microstructure than that of the conventional heating treatment as the evidence of the disruption of the cell membrane structure as a consequence of electroporation.”
Table 2. Total phenolic content and antioxidant activity of cooked samples.
The trends for the antioxidant activity values were similar to those for the total phenolic contents because phenolic compounds are the key constituents that contribute to the total antioxidant activity of rice.[Citation37] Nonetheless, the values of antioxidant activity in demonstrated that the ohmic cooking method had significantly greater antioxidant activity levels than those using the conventional method in all cases. This result was slightly different from the total phenolic contents since the antioxidants in the samples were not only phenolic compounds but also other substances such as flavonoids, vitamin E, phytosterols, and pigments.[Citation22,Citation35,Citation37] Therefore, the effect of different cooking methods on these antioxidants could be dissimilar to that regarding the phenolic compounds. Furthermore, the lotus seed, red kidney bean, and Job’s tears contain high antioxidants[Citation3–5]; thus, the precooking step applied in the conventional cooking approach would produce lower antioxidant activity values compared to the ohmic cooking that did not precook these whole grains.
Vitamin B1, calcium, phosphorus, and potassium
The vitamin B1, calcium, phosphorus, and potassium contents of the cooked samples using the ratio of KDML 105-to-Riceberry-to-red cargo rice of 1:2:1 are presented in . These samples were selected for this analysis because they had significantly higher values for total phenolic content and antioxidant activity than their counterparts, implying that they should be preferable for the health-conscious consumer. The vitamin B1, calcium, phosphorus, and potassium contents were examined because they are the key vitamin and minerals found in lotus seed, red kidney bean, Job’s tears, and brown rice. The results indicated that the sample cooked using the ohmic method contained significantly higher amounts of calcium, phosphorus, and potassium than that cooked by conventional method. On the other hand, the vitamin B1 contents were not significantly different. In addition, the amounts of vitamin B1 were much lower than for calcium, phosphorus, and potassium because the contents of these minerals in the whole grains and brown rice are usually much higher than the vitamin B1 amount. In addition, the water-soluble nature of vitamin B1 contributed to this result as a substantial reduction in the vitamin B1 content probably occurred during the soaking period. Despite reusing the solution from soaking the brown rice when cooking the samples, any vitamin B1 leaching into the solution would be simply lost during the cooking process since it is highly sensitive to damage by heat. In contrast, these minerals did not easily dissolved in water; therefore, their losses during soaking period would be expected to be less than for vitamin B1. Furthermore, these minerals are typically heat tolerant, resulting in lower losses after cooking. Kennedy & Burlingame[Citation38] reported that the vitamin B1 contents in raw, unpolished samples of 79 diverse rice cultivars were in the range 0.117–1.74 mg/100 g, whereas Villareal & Juliano[Citation39] stated that the vitamin B1 contents in the raw unpolished rice samples of five pigmented traditional Philippine rice varieties were in the range 0.33–0.46 mg/100 g. In comparison, the current results regarding vitamin B1 contents were rather low because these samples had been exposed to soaking and cooking processes.
Table 3. Vitamin B1, calcium, phosphorus, and potassium contents of cooked samples.
Notably, ohmic cooking provided significantly higher contents of calcium, phosphorus, and potassium than the conventional cooking approach by around 13, 8, and 21% respectively. The greater remaining amounts of these minerals following cooking using the ohmic method could have been mainly caused by the exclusion of precooking process for the whole grains because for the conventional cooking approach, the lotus seed and Job’s tears were placed in boiling water for 15 minutes while it was 17 minutes and 30 seconds for the red kidney bean.
Energy consumption
The energy consumption values for cooking the mixtures of brown rice and whole grains using the conventional or ohmic cooking method are presented in . The results revealed that ohmic cooking consumed more energy than using the electric rice cooker which contrasted with the studies of other researchers, such as Jittanit et al.,[Citation9] Kanjanapongkul,[Citation10] and Gavahian et al.[Citation11] who reported that cooking rice using an ohmic heating apparatus needed significantly less electrical energy than the commercial electric rice cooker. Normally, it is recognized that ohmic heating method is a more energy-efficient heating technique than the conventional heating method because ohmic heating is based on the direct heating concept.[Citation40] Nevertheless, the energy consumption for the ohmic cooking apparatus in the current study was approximately 2% higher energy than for the electric rice cooker because this ohmic cooking system utilized two transformers () to supply a sufficient strong electrical field in the ohmic cell, resulting in higher losses, comprising hysteresis loss, stray loss, eddy current loss, dielectric loss, and copper loss, with a subsequent decline in the power factor for the whole system.[Citation41–43] Notably, the power factor for using each of these transformers was approximately 0.8, resulting in an overall power factor of about 0.64 when connected in series. Apart from the losses at the transformers, there were heat losses to the surroundings, including from the electrodes, agitator, and ohmic cell, leading to the slightly greater energy consumption for cooking the grain samples than from using the electric rice cooker. Tumpanuvatr & Jittanit[Citation8] used a similar ohmic heating apparatus to that in the current study, except that their ohmic cell was a cube with sides of 200 mm (double that in the current study) while their sample size in each cooking batch was about triple (≈1,800 g of mixture, comprising 600 g brown rice and approximately 1,200 g solution). Tumpanuvatr & Jittanit[Citation8] reported that their ohmic system used to cook brown rice consumed more electrical energy than their electric rice cooker by 69–162%, depending on the types of brown rice samples. This result indicated that the energy efficiency of ohmic cooking strongly depends on the engineering design of the process. For example, if the number of connecting transformers were increased or the size of ohmic cell were not matched with the volume of sample, the efficiency would decline. Another issue that must be considered in the design of an ohmic heating apparatus should bear is that the energy consumption for cooking food grains can be reduced if the heating rate is accelerated to be greater than that of the electric cooker to lessen the total energy loss during cooking. The heating rate of ohmic method can be raised by increasing either the electrical field strength or the electrical conductivity of sample.
Table 4. Electrical energy consumption values measured during cooking process of samples.
Conclusion
The electrical conductivities of all mixture samples of food grains and liquids in this study were high enough for ohmic heating. The electrical conductivities of lotus seed mixed with water were greater than those of similarly prepared samples of red kidney bean and Job’s tears respectively. The Riceberry brown rice variety had the highest electrical conductivity, followed by the brown rice of the red cargo rice and KDML 105 varieties, respectively. The linear models representing the relationships between the measured electrical conductivities of samples and temperatures established in this study could be applied to estimate the electrical conductivities of samples during the ohmic heating process. When cooking the mixtures of brown rice and whole grains using the conventional method, the lotus seed, red kidney bean, and Job’s tears must be precooked in boiling water before cooking them together with the brown rice while the precooking step was unnecessary using ohmic cooking, making ohmic cooking more convenient. In addition, ohmic cooking provided significantly better retention of antioxidant activity and calcium, phosphorus, and potassium contents in the cooked samples than from conventional cooking. This finding should appeal to the health-conscious consumer. The current study demonstrated that ohmic cooking consumed slightly more energy than from using the electric rice cooker, even though the temperature profile for all samples during cooking by either method were the same. This result confirmed that ohmic heating was not always more energy efficient than conventional heating, as would be generally expected.
Acknowledgments
This work was supported by the Kasetsart University Research and Development Institute (KURDI), Bangkok, Thailand under Grant [R-M 38.64].
Disclosure statement
No potential conflict of interest was reported by the author(s).
Additional information
Funding
References
- Wu, X.; Guo, T.; Luo, F.; Lin, Q. Brown Rice: A Missing Nutrient-Rich Health Food. Food Sci. Hum. Wellness. 2023, 12(5), 1458–1470. DOI: 10.1016/j.fshw.2023.02.010.
- Chen, C.; Li, G.; Zhu, F. A. Novel Starch from Lotus (Nelumbo nucifera) Seeds: Composition, Structure, Properties and Modifications. Food Hydrocoll. 2021. 120,106899. DOI: 10.1016/j.foodhyd.2021.106899.
- Bangar, S. P.; Dunno, K.; Kumar, M.; Mostafa, H.; Maqsood, S. A Comprehensive Review on Lotus Seeds (Nelumbo Nucifera Gaertn.): Nutritional Composition, Health-Related Bioactive Properties, and Industrial Applications. J. Funct. Foods. 2022, 89, 104937. DOI: 10.1016/j.jff.2022.104937.
- Link, R. Kidney Beans Nutrition Helps Fight Diabetes, Heart Disease & Even Some Cancers. 2019. Website DrAxe.Com: Dr. Axe Co-Founders of Ancient Nutrition. https://draxe.com/nutrition/kidney-beans-nutrition/ (accessed Feb 19, 2024).
- Devaraj, R. D.; Jeepipalli, S. P. K.; Xu, B. Phytochemistry and Health Promoting Effects of Job’s Tears (Coix Lacryma-Jobi) - A Critical Review, Food Biosci. 2020, 34 100537. DOI:10.1016/j.fbio.2020.100537
- Palaniappan, S.; Sastry, S. K. Electrical Conductivity of Selected Juices: Influences of Temperature, Solids Content, Applied Voltage, and Particle Size 1. J. Food Process. Eng. 1991, 14(4), 247–260. DOI: 10.1111/j.1745-4530.1991.tb00135.x.
- Sastry, S. K.; Li, Q. Modelling the Ohmic Heating of Foods. Food Technol. 1996. 50(5), 246–248.
- Tumpanuvatr, T.; Jittanit, W. Quality Improvement of Refrigerated Ready-To-Eat Cooked Brown Rice by Adding Gellan Gum and Trehalose with Ohmic Heating Compared to Conventional Cooking Method. J. Food Process Preserv. 2022, 46(4), e16443. DOI: 10.1111/jfpp.16443.
- Jittanit, W.; Khuenpet, K.; Kaewsri, P.; Dumrongpongpaiboon, N.; Hayamin, P.; Jantarangsri, K. Ohmic Heating for Cooking Rice: Electrical Conductivity Measurements, Textural Quality Determination and Energy Analysis. Innov. Food Sci. Emerg. Technol. 2017, 42, 16–24. DOI: 10.1016/j.ifset.2017.05.008.
- Kanjanapongkul, K. Rice Cooking Using Ohmic Heating: Determination of Electrical Conductivity, Water Diffusion and Cooking Energy. J. Food Eng. 2017, 192, 1–10. DOI: 10.1016/j.jfoodeng.2016.07.014.
- Gavahian, M.; Chu, Y. H.; Farahnaky, A. Effects of Ohmic and Microwave Cooking on Textural Softening and Physical Properties of Rice. J. Food Eng. 2019, 243, 114–124. DOI: 10.1016/j.jfoodeng.2018.09.010.
- Tumpanuvatr, T.; Jittanit, W. Physical Properties, Total Phenolic Contents, and Antioxidant Activities of Refrigerated Ready-To-Eat Brown Rice Cooked by Ohmic and Conventional Methods: Effect of Gellan Gum. Int. J. Food. Prop. 2022, 25(1), 2381–2395. DOI: 10.1080/10942912.2022.2135538.
- Wolfe, K.; Wu, X.; Liu, R. H. Antioxidant Activity of Apple Peels. J. Agr. Food. Chem. 2003, 51(3), 609–614. DOI: 10.1021/jf020782a.
- Tachibana, Y.; Kikuzaki, H.; Lajis, N. H.; Nakatani, N. Antioxidative Activity of Carbazoles from Murraya koenigii Leaves. J. Agric. Food. Chem. 2001. 49 11, 5589–5594. DOI: 10.1021/jf010621r.
- AOAC. In-House Method Thiamine (Vitamin B1). The Association of Official Analytical Chemists, Gaithersburg, MD, USA, 2000. Method 942.23.
- AOAC. In-House Method Calcium, Copper, Iron, Magnesium, Manganese, Phosphorus, Potassium, Sodium, and Zinc in Infant Formula. The Association of Official Analytical Chemists, Gaithersburg, MD, USA, 1986. Method 984.27.
- Piette, G.; Dostie, M.; Ramaswamy, H. Ohmic Cooking of Processed Meats - State of the Art and Prospects. 47th International Congress of Meat Science and Technology. 2001; 62–67.
- Icier, F.; Ilicali, C. Temperature Dependent Electrical Conductivities of Fruit Purees During Ohmic Heating, Food Res. Food Res. Int. 2005. 38 10, 1135–1142. DOI:10.1016/j.foodres.2005.04.003.
- Tumpanuvatr, T.; Jittanit, W. The Mathematical Models for Electrical Conductivities of Fresh Juices, Concentrated Juices and Purees During Ohmic Heating. Thai J. Agric. Sci. 2011. 44(5), 312–318.
- Zareifard, M. R.; Marcotte, M.; Ramaswamy, H. S.; Karimi, Y. Chapter 6: Modeling of Electrical Conductivity in the Context of Ohmic Heating. In Ohmic Heating in Food Processing Ramaswamy, H. S., Marcotte, M., Sastry, S., and Abdelrahim, K. (Eds.); CRC Press, Taylor & Francis Group: Boca Raton, FL, 2014; pp. 67–89.
- Tulsiyan, P.; Sarang, S.; Sastry, S. K. Electrical Conductivity of Multicomponent Systems During Ohmic Heating. Int. J. Food. Prop. 2008, 11(1), 233–241. DOI: 10.1080/10942910701302580.
- Chiang, A. N.; Wu, H. L.; Yeh, H. I.; Chu, C. S.; Lin, H. C.; Lee, W. C. Antioxidant Effects of Black Rice Extract Through the Induction of Superoxide Dismutase and Catalase Activities. Lipids. 2006, 41(8), 797–803. DOI: 10.1007/s11745-006-5033-6.
- Juliano, B. O.; Bechtel, D. B. Criteria and Tests for Rice Grain Qualities. In Rice Chemistry and Technology. St Juliano, B. O. (Ed.), Paul, Minnesota: American Association of Cereal Chemists, Inc. 1985; pp 17–57.
- Roy, P.; Nei, D.; Orikasa, T.; Okadome, H.; Thammawong, M.; Nakamura, N.; Shiina, T. Cooking Properties of Different Forms of Rice Cooked with an Automatic Induction Heating System Rice Cooker. Asian J. Food Agro-Ind. 2010. 3(4), 373–388.
- Li, F. D.; Li, L. T.; Li, Z.; Tatsumi, E. Determination of Starch Gelatinization Temperature by Ohmic Heating. J. Food Eng. 2004, 62(2), 113–120. DOI: 10.1016/s0260-8774(03)00199-7.
- Li, M.; Wang, B.; LV, W.; Lin, R.; Zhao, D.; Characterization of Pre-Gelatinized Kidney Bean (Phaseolus Vulgaris L.) Produced Using Microwave Hot-Air Flow Rolling Drying Technique. LWT - Food Sci. Technol. 2022.154, 112673. DOI: 10.1016/j.lwt.2021.112673.
- Dhull, S. B.; Chandak, A.; Collins, M. N.; Bangar, S. P.; Chawla, P.; Singh, A. Lotus Seed Starch: A Novel Functional Ingredient with Promising Properties and Applications in Food—A Review. Starch Stärke. 2022 74 9–10. DOI: 10.1002/star.202200064.
- Chaisiricharoenkul, J.; Tongta, S.; Intarapichet, K. Structure and Chemical and Physicochemical Properties of job’s Tear (Coix Lacryma-Jobi L.) Kernels and Flours. Suranaree J. Sci. Technol. 2011. 18(2), 109–122.
- Tumpanuvatr, T.; Jittanit, W.; Surojanametakul, V. Effects of Drying Conditions in Hybrid Dryer on the GABA Rice Properties. J. Stored Prod. Res. 2018, 77, 177–188. DOI: 10.1016/j.jspr.2018.04.009.
- Kraithong, S.; Lee, S.; Rawdkuen, S. Physicochemical and Functional Properties of Thai Organic Rice Flour. J. Cereal Sci. 2018, 79, 259–266. DOI: 10.1016/j.jcs.2017.10.015.
- An, H. J.; King, J. M. Thermal Characteristics of Ohmically Heated Rice Starch and Rice Flours. J. Food Sci. 2007, 72(1), C084–C088. DOI: 10.1111/j.1750-3841.2006.00239.x.
- Siqueira, B. D. S.; Vianello, R. P.; Fernandes, K. F.; Bassinello, P. Z. Hardness of Carioca Beans (Phaseolus Vulgaris L.) As Affected by Cooking Methods. LWT - Food Sci. Technol. 2013. 54 (1), 13–17. DOI: 10.1016/j.lwt.2013.05.019.
- Chanu, C. S.; Shivaleela, H. B.; Ravindra, U. Physicochemical and Cooking Properties of Rice (Sambha masuri) Individually Fortified with Iron, Zinc and Calcium. Int. J. Curr. Microbiol. App. Sci. 2020, 9(1), 315–327. DOI: 10.20546/ijcmas.2020.901.036.
- Svarna, M.; Mavromatis, A.; Vlachostergios, D. N.; Gerasopoulos, D. Modeling the Effects of Seed Maturity on Cooking Time of ‘Dimitra’ Lentils. Foods. 2023. 12 (1), 42. DOI: 10.3390/foods12010042.
- Suttiarporn, P.; Sookwong, P.; Mahatheeranont, S. Fractionation and Identification of Antioxidant Compounds from Bran of Thai Black Rice Cv. Riceberry. Int J Chem Eng Appl. 2016. 7, 109–114. DOI: 10.7763/ijcea.2016.v7.552.
- Aamir, M.; Jittanit, W. Ohmic Heating Treatment for Gac Aril Oil Extraction: Effects on Extraction Efficiency, Physical Properties and Some Bioactive Compounds. Innov. Food Sci. Emerg. Technol. 2017, 41, 224–234. DOI: 10.1016/j.ifset.2017.03.013.
- Goufo, P.; Trindade, H. Rice Antioxidants: Phenolic Acids, Flavonoids, Anthocyanins, Proanthocyanidins, Tocopherols, Tocotrienols, C-Oryzanol, and Phytic Acid. Food Sci. & Nutr. 2014. 2(2), 75–104. DOI: 10.1002/fsn3.86
- Kennedy, G.; Burlingame, B. Analysis of Food Composition Data on Rice from a Plant Genetic Resources Perspective. Food Chem. 2003, 80(4), 589–596. DOI: 10.1016/S0308-8146(02)00507-1.
- Villareal, C.; Juliano, B. Variability in Contents of Thiamin and Riboflavin in Brown Rice, Crude Oil in Brown Rice and Bran-Polish, and Silicon in Hull of IR Rices. Plant Foods Hum. Nutr. 1989. 39 (3), 287–297. DOI: 10.1007/BF01091939.
- Dias-Martins, A. M.; Cappato, L. P.; Mattos, M. D. C.; Rodrigues, F. N.; Pacheco, S.; Carvalho, C. W. P. Impacts of Ohmic Heating on Decorticated and Whole Pearl Millet Grains Compared to Open-Pan Cooking. J. Cereal Sci. 2019. 85, 120–129. DOI: 10.1016/j.jcs.2018.11.007.
- Demirdelen, T. Optimal Design and Experimental Validation Long-Lasting, Low Loss Transformer for Low Power Renewable Energy System. Energy Source Part A: Recovery Util. And Environ. Eff. 2019. 41(20), 2534–2548. DOI: 10.1080/15567036.2019.1637973.
- Galvan, J. C. O.; Georgilakis, P. S.; Valdez, R. O. A review of transformer losses. Electr. Power Compon. Syst. 2009. 37, 1046–1062. DOI: 10.1080/15325000902918990
- Kulkarni, V. A.; Katti, P. K. Estimation of distribution transformer losses in feeder circuit. Int. J. Electr. Comput. Eng. 2011. 3(5), 659–662. DOI: 10.7763/IJCEE.2011.V3.399.