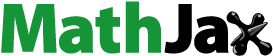
Abstract
We applied a modified assay for the measurement of the intracellular ATP content as an alternative biochemical method for estimating the viability of the BCG vaccine instead of the standard cultural microbiological assay. Eight lyophilized BCG ampoule samples (Lot 287-2 and Lot 254-2, Bulgarian BCG strain SL222 Sofia) were tested on different days. Each calibration curve was measured for the following range of ATP concentrations: 100, 10, 5, 2.5, 1.25, 0.625, 0.3125, and 0.1562 pmol. The obtained results were compared to the traditional cultural colony counting method for evaluating the viability of the BCG vaccine. The NCP of Lot 287-2 (min 2.325–max 3.300) was higher in comparison to Lot 254-2 (min 1.438–max 3.330). That result corresponded to higher ATP-content registered for Lot 287-2 (min 0.409–max 1.338) than Lot 254-2 (min 0.18–max 1.104). Our results confirmed the observation made by other researchers that the modified ATP assay is a reliable, simple, and quick method for the evaluation of the viability of the BCG vaccine. Additional compiled data about all BCG strains are needed to perform studies on the correlation between the luciferin-luciferase bioluminescent ATP assay and the cultural method for viability and enumeration of the units in the lyophilized BCG vaccine.
Introduction
Tuberculosis has evolved and developed among humans since their initial migration from Africa, before ∼70,000 years [Citation1]. More than a billion people have died from tuberculosis during the last 200 years, more than from any other infectious disease in the history of mankind [Citation2]. The impact of tuberculosis epidemics has been mitigated by improved living conditions, the introduction of the Bacille Calmette-Guérin (BCG) vaccination, and the development of new antibiotics. However, in certain regions, the disease still has a significant impact. There are an estimated 10.7 million new cases and 1.6 million deaths in 2021 [Citation3].
In the era of integrative systems biology, the BCG vaccination stands as a sentinel, prompting us to delve into the intricate cellular choreography within our immune systems. As we unravel these complexities, we gain insight not only into how vaccines protect against disease but also into the fundamental workings of our bodies at a cellular level.
The only possible tuberculosis protection is the vaccination with the attenuated strain of Mycobacterium bovis—BCG (Bacillus Calmette-Guérin). The BCG vaccination is considered to have been administered to about 4 billion individuals in the world, more than a century after its discovery. BCG vaccinations are given more frequently than any other worldwide. The live mycobacteria used to manufacture the freeze-dried BCG vaccinе are derived from a culture of the Bacillus Calmette-Guérin substrain. The viability of the vaccine, which is a marker for the efficacy of BCG, is crucial for inducing the protective immune response. For this reason, measuring the viable count is essential to quality control. The colony-forming unit (CFU) assay is currently the gold standard that the World Health Organization (WHO) recommends for determining the potency of the BCG vaccine. This direct approach counts the quantity of microorganisms when cultured on a solid Löwenstein-Jensen medium; the test sample is later serially diluted. However, the variation of the CFU assay can vary a lot (5–50%) due to dilution errors, medium modifications made during cultivation, and counting errors because of mycobacterial clumping. Furthermore, due to the very slow growth rate, colony formation takes between four and five weeks. As a result, vaccine production and control take a very long time. The laboriousness of the culture method, the long duration of the test, as well as the many other factors influencing the consistency of the results, have always been a great challenge for BCG vaccine manufacturers and control laboratories [Citation4]. For this reason, the search, validation, and implementation of more rapid and reproducible alternative methods for viability control is a priority, which has also been included at WHO consultative meetings for the characterization and improvement of BCG vaccine quality control [Citation5].
Consequently, the development of an easily performed, rapid, and accurate method to quantify the viability of BCG lots is strongly advisable. For example, the evaluation of adenosine triphosphate (ATP) is an alternative practical method for the express detection of the viability of the BCG vaccine [Citation6]. It is part of the WHO’s initiative to modernize the methods and assays for BCG vaccine viability evaluation [Citation7].
Measuring the amount of ATP in a sample can yield accurate estimates of the number of living cells because ATP is a main metabolite of living cells and is rapidly lost in dead cells. The Statens Serum Institute (SSI) has created a modified ATP bioluminescence assay as a quick substitute for the viable count of the BCG vaccine [Citation8]. This ATP assay is based on the bioluminescent product that firefly luciferase produces when it reacts with ATP. Light is released as a result of the reaction [Citation9]. When ATP standards are used for calibration, it is possible to determine the ATP content of the sample, which is directly proportional to the intensity of the detected light emission. There is a strong relationship between the amount of live BCG bacilli in various vaccine formulations and the intracellular ATP concentrations. Additionally, the results of a global collaborative investigation initiated by the WHO showed that the ATP bioluminescence test is a reliable, robust, repeatable method that may be regularly employed as a quality control tool in the production of the BCG vaccine [Citation7]. This method’s ability to assess incredibly low metabolic concentrations (0.01 pmol/L) is another key benefit.
The ATP assay is based on a luminescent reaction. The majority of bioluminescent processes are initiated by oxidation or oxygenation. The exerted energy is spent on the transfer of the molecules from basic, low-energy to excited, high-energy levels. The reverse transfer into the basic state is accompanied by luminescent energy emissions. Different biological species possess various luminescent mechanisms, but common substrates and corresponding enzymes. For example, the luciferins are oxidized by the luciferase enzyme, producing oxyluciferin and light energy [Citation10]:
Luciferase catalyzes the interaction of luciferin and ATP in the presence of divalent metal ions (Mg2+, Fe2+, Co2+, Zn2+, or Mn2+). Luciferyl adenylate and pyrophosphate are produced. Luciferyl adenylate is oxidized by molecular oxygen, producing oxyluciferin and AMP, accompanied by light emission [Citation9,Citation11,Citation12]. The total quantum yield from the reaction is about 0.9 photons per oxidized luciferin molecule. The final product of this reaction—oxyluciferin, is formed in a single excited state as a result of the intermolecular annihilation. Yellow-green light in the range of 550–570 nm is emitted when oxyluciferin transfers into a basic state. This phenomenon is called the ‘lantern effect’. The key point is that the bioluminescent effect cannot be observed in the absence of ATP. These are the basics for the most sensitive method for analyses of ATP [Citation13]. The method is based on measuring the ATP content in various samples by measuring the level of luminescence. As long as every living cell contains a specific concentration of ATP, the evaluation of the total amount of ATP is a direct parameter for the number of living cells in the volume of the tested sample.
The standard test applies a natural firefly or synthetic luciferin-luciferase mixture:
where: AMP—adenosine monophosphate, PP—pyrophosphate, E—luciferase, LH2—luciferin, P* and P—product (oxyluciferin) in excited and basic state, respectively. The presence of divalent metal ions is obligatory for the production of light emissions. The reaction rate is proportional to the ATP content. The concentration range of 10−12–10−6 mol/L appears to be the practical limitation for this assay. ATP estimations up to
mol/L or smaller concentrations are also possible under refined conditions. This method is widely applied for the evaluation of bacterial contamination, blood cell viability, antibiotic resistance research, etc. The contemporary methods for bioluminescent registration afford direct counting of the emitted quanta or measurement of the intensity of the light emission (I). The registered intensity is proportional to the rate of light emission (
) or the electronically excited production rate (
). The bioluminescent assay affords the evaluation of extremely low metabolic concentrations, which is the main advantage of this method. The ATP content is proportional to the number of living cells or the living biomass [Citation8,Citation14–16]. Results are given according to the calibration curve: cell number or biomass/intensity of bioluminescence. The enumeration error that appears in a single microbial count is about 10–15%. The average light signal from a single cell corresponds to the standard ATP concentration in the pro- or eukaryotic cell [Citation7,Citation17].
This paper describes our work to establish the suitability of the alternative ATP potency assay for use as routine viability testing in the production of the BCG vaccine in Bulgaria.
The aim of the study was to
utilize the ATP assay for the evaluation of the viability of the lyophilized BCG vaccine based on the method introduced by the Statens Serum Institute (SSI), Copenhagen [Citation8]
to evaluate the ATP content in ampoule samples from the lyophilized BCG vaccine produced from the Bulgarian BCG strain SL222 Sofia (originating from the Moscow BCG strain BCG I) manufactured by BB-NCIPD Ltd., Sofia, BG by the modified ATP assay.
Materials and methods
ATP assay
Reagents
BCG samples
The samples from BCG Lot 254-2 and BCG Lot 287-2 (both originating from the Bulgarian BCG substrain SL222 Sofia) were produced and supplied by BB-NCIPD Ltd. The ampoules were stored at 2–8 °C for 25 and 21 months, respectively. ATP Standard, 1 × 10−5 mol/L: Stock ATP Standard was aliquoted and stored at −20 °C until use. Dilutions were prepared in TE buffer. Stock ATP standard = 1000 pmol/100 µL. The recommended working ATP standards were prepared as 1:2 serial dilutions from 10 to 0.3125 pmol/100 µL.
Key materials required:
Dubos medium (supplemented with 0.045% Tween 80 and 0.5% bovine albumin; 4347, SSI Diagnostika, Hillerød, Denmark)
ATP Kit SL (144-041, BioThema, Haninge, Sweden) included Tris-EDTA (TE) buffer (0.1 mol/L) with 2 mmol/L EDTA, pH 7.75 adjusted with acetic acid, and stored at 4 °C until use; ATP Reagent SL—it was reconstituted with 10 mL Diluent C. Aliquots of reconstituted ATP reagent were stored at −20 °C until use; ATP Standard, 1 × 10−5 mol/L
2 mL sterile screw-cap tubes;
Heat block: to heat the buffer up to 96–98 °C.
Procedure
Three 1:2 serial dilutions were prepared from each ampoule, and each dilution was tested in triplicate, yielding three luminescent measurements about each dilution or nine luminescent readings per ampoule. Each ampoule was reconstituted with 1.0 mL Dubos medium, mixed well, and transferred to sterile screw-capped tubes. The reconstituted BCG suspensions were incubated at 37 °C for 22–26 h. Enough time was allowed for the dried heat block to heat up as the temperature was measured with a thermometer inside the heat block. 500 µL TE buffer was put in 2 mL screw top tubes. The TE buffer temperature was kept between 96 and 98 °C; evaporation was avoided. Then, 100 µL of BCG suspension was added directly to the preheated buffer. The BCG-buffer mixture was returned to the heat block and kept there for exactly 6 min. The negative control was prepared as samples using the Dubos medium without BCG. After heat treatment, the resulting extracts were allowed to cool to room temperature before the addition of the ATP reagent. ATP standards were prepared in triplicate as mentioned before, and 100 µL of each standard was added into 500 µL of TE buffer, but no heating was required. To each 600 µL ATP extract/standard, 100 µL ATP reagent was added, and the luminescence of the sample was measured on a luminescence counter. Samples and ATP standards were read exactly 5 min after the addition of the ATP reagent, and readings followed fixed time intervals [Citation8].
Culturable particle counting of the BCG vaccine
The traditional method for evaluating BCG potency is usually performed by counting the culturable particles in a selective medium. This method is based on the recommendations of WHO (WHO/TB/Techn.Guide/77.9) [Citation18]. In order to confirm ampoule integrity, each ampoule of BCG vaccine was checked for vacuum before starting the test. The content of one ampoule was reconstituted thoroughly in 1 mL saline. Three dilution series were prepared. For each dilution series, equal volumes of the diluted vaccine (0.1 mL) were inoculated on a Löwenstein-Jensen medium (BB-NCIPD Ltd.) surface, using altogether 16 containers. The three serial dilutions were inoculated onto four, four, and eight tubes with Löwenstein-Jensen medium, respectively. The tubes were appropriately randomized. The inoculum was spread carefully on the medium surface, followed by incubation at 37 °C for 35 days before counting the number of colonies.
Data analysis
Data are shown as mean values with standard deviation (±SD). The statistical analysis was performed using Microsoft Excel, Origin Pro 6.1, and the Student’s t-test.
Results
In the present study, we applied a modified assay for the measurement of the intracellular ATP content as an alternative biochemical method for the estimation of the viability of the BCG vaccine strain SL222 Sofia, instead of the standard cultural microbiological assay.
Eight lyophilized BCG ampoule samples (Lot 287-2 and Lot 254-2, Bulgarian BCG strain SL222 Sofia) were tested on different days. Separate calibration curves, applied in the calculations of the results, were prepared on each experimental day (). Each curve was measured for the following range of ATP concentrations: 100, 10, 5, 2.5, 1.25, 0.625, 0.3125, and 0.1562 pmol. For better accuracy, the value for each result was calculated according to the curve given by the closest concentrations in the range (). Having separate controls on each experimental day is extremely important as the luciferin-luciferase system changes its enzyme activity in hours, even when stored at proper conditions (−20 °C). It should be noted that the blank control samples always gave signals that were quite close but not equal: the luminescence achieved from the one prepared with TE buffer, Dubos medium, and ATP reagent was lower (∼1.1 mV) than the signal from the blank sample not containing Dubos medium (∼1.4 mV). This can be explained by a higher medium density in the control sample containing Dubos medium, leading to slightly lower signal emission.
Figure 1. Chemiluminescent calibration curve in the range 10, 5, 2.5, 1.25, 0.625, 0.3125 pmol ATP (A) and 1.25, 0.625, 0.3125 pmol ATP and the calculation of the results according to the linear equation (B).

After all these considerations, the following results about the undiluted and two 1:2 serial dilutions were obtained ().
Table 1. Concentrations of ATP [pmol] in different ampoules of two BCG vaccine samples.
The observed differences in the ATP content between the two vaccine lots could be explained by the influence of the lyophilization process on the proportion of live/dead organisms in each lot. The data show the expected higher ATP content in the undiluted samples in comparison to the concentrations of the diluted ones. The registered ATP content in the 1:2 and 1:4 serial dilutions was lower but did not change in a linear double reduction sequence.
The following results () were obtained by applying the traditional cultural colony counting method for the evaluation of the viability of the BCG vaccine in the BCG Laboratory, BB-NCIPD Ltd.:
Table 2. Number of culturable particles (NCP) × 106/mL.
Discussion
The cultural method is based on counting the Number of Culturable Particles (NCP) determined after cultivation at 37 °C on a solid egg Löwenstein-Jensen medium. It is slow and labour-intensive. There are numerous sources of errors that can influence the results, such as the type and conditions in the medium and the diluent, the type of flasks used, the dilution series, the ‘individual factor’, etc. One of the main features of BCG organisms is their hydrophobicity and tendency to clump. Due to the various extents of bacilli clumping, the consecutive lots of one and the same production series, as well as the different ampoules of one and the same lot, never contain exactly the same NCP.
The NCP of Lot 287-2 (min 2.325–max 3.300) was higher in comparison to Lot 254-2 (min 1.438–max 3.330). That result corresponded to a higher ATP content, registered for Lot 287-2 (). However, additional testing, data, and statistics of the BCG samples are needed to calculate the correlation between the NCP and ATP content.
ATP is the universal energy carrier of every living cell, carrying out intracellular energy transfer and all metabolic processes necessary for the existence, growth, and replication of living organisms. This nucleotide is synonymous with life as it is the basis of all living processes in prokaryotic and eukaryotic cells. ATP is considered one of the most important indicators of cell viability, and one convenient way to measure it is by high-sensitivity luciferase-based bioluminescence assays. ATP bioluminescence assays are suitable for a variety of cell types and are currently applied in a wide range of studies [Citation19]. Recently, the test for the evaluation of intracellular ATP has been developed as an alternative and faster possibility to assess the viability of the BCG vaccine, avoiding many of the disadvantages of the classical culture method, which are related to its subjectivity, slowness, and variability of results [Citation20–22]. Some of these issues are associated with the clumping of mycobacteria, and it is known that batch-to-batch and vendor-to-vendor differences in vaccine and nutrient media, respectively, can generate 1- to 2-log variance for vaccine samples [Citation23]. In 2017, Gweon et al. [Citation24] demonstrated that ‘the CV% between different laboratories can be as high as 95% (with an average of 49%) when using the CFU assay for analysis’ [Citation24,Citation25].
The data that we present describe the first application of this method for the Bulgarian BCG vaccine (Bulgarian BCG strain SL222 Sofia) and confirm the results of other authors for the applicability of the method in assessing BCG vaccine viability [Citation26–28]. To introduce the method into the routine practice, it is necessary to accumulate additional data on the correlation between the calculated amount of ATP and the CFU in the BCG vaccine from which it was extracted. It is also necessary to validate the already established parameters, methods, and conditions of testing according to the following indicators: linearity, accuracy, precision, repeatability, and robustness.
The ATP bioluminescence assay itself could also be subject to additional processing steps, such as improving the extraction of intracellular ATP to correct some critical points leading to poor correlation in results [Citation25]. The extreme sensitivity of all luciferase systems (up to 0.01 pmol/L) is defined by the high quantum yield of bioluminescence. Each reacting luciferin molecule corresponds to 0.3–1 light quantum. The bioluminescent assay has a far better sensitivity than conventional spectrophotometric or fluorometric methods [Citation29]. Another feature of that method is its absolute specificity to ATP and NAD.H, which is of great practical advantage. The registered signal values and the evaluated substance concentration exhibit an exceptionally wide range of linearity. Other advantages of this method include the need for and usage of small volumes from the examined sample, as well as the simple and not very expensive equipment.
The suitability of this modified ATP assay, as proposed by the SSI, Copenhagen was applied in a collaborative study with the participation of seven laboratories and four manufacturers [Citation7]. Our results confirm the observation made by other researchers that the modified ATP assay is a reliable, simple, and quick method for the evaluation of the viability of the BCG vaccine. Additional investigations can be advanced by a detailed correlation analysis between the cultural viable counts and ATP contents of the BCG vaccine produced by various (sub)strains. Our research was part of a collaborative project when the WHO Requirements for the BCG vaccine were revised and updated with this new quality control test to monitor the BCG viability, providing better characterization of the BCG vaccine [Citation3,Citation30–36].
Conclusions
The modified ATP assay is a very reliable and quick method for evaluating the viability of the BCG vaccine. The reproducibility of the obtained results was also validated by other researchers and reference laboratories. Additional compiled data about all BCG strains is needed to perform studies on the correlation between the luciferin-luciferase bioluminescent ATP assay and the cultural method for viability and enumeration of the units in the lyophilized BCG vaccine.
Author contributions
The authors have contributed substantially to the work reported. E.P.: conceptualization and design of the study, performance of the ATP luminescent assays, calculations and data analysis, visualization, conclusions, and writing the major part of the draft manuscript. A.M.: performance of the microbiological assays and calculations. T.S.: conceptualization and design of the study, writing–review and editing; funding acquisition. All three authors discussed together the obtained results and conclusions and made corrections to the text. All authors have read and approved the final version of the paper.
Disclosure statement
No potential conflict of interest was reported by the author(s).
Data availability statement
The data are available on request from the corresponding author [E.P.] upon reasonable request.
Additional information
Funding
References
- Comas I, Coscolla M, Luo T, et al. Out-of-Africa migration and neolithic coexpansion of Mycobacterium tuberculosis with modern humans. Nat Genet. 2013;45(10):1–8. doi: 10.1038/ng.2744.
- Paulson T. Epidemiology: a mortal foe. Nature. 2013;502(7470):S2–S3. doi: 10.1038/502S2a.
- World Health Organization. WHO report, 2022, global tuberculosis control. 2022.
- Stefanova T. Quality control and safety assessment of BCG vaccines in the post-genomic era. Biotechnol Biotechnol Equip. 2014;28(3):387–391. doi: 10.1080/13102818.2014.927200.
- WHO Expert Committee on Biological Standardization. Recommendations to assure the quality, safety and efficacy of BCG vaccines. In: World Health Organisation technical report series 2011, No. 979, Annex 3; 2011. p. 137–185.
- Crispen RG. Rapid testing of freeze-dried BCG vaccine for stability and viability. Symp Ser Immunobiol Stand. 1971;17:205–210.
- Ho MM, Markey K, Rigsby P, et al. Report of an international collaborative study to establish the suitability of using modified ATP assay for viable count of BCG vaccine. Vaccine. 2008;26(36):4754–4757. doi: 10.1016/j.vaccine.2008.06.026.
- Jensen SE, Hubrechts P, Klein B, et al. Development and validation of an ATP method for rapid estimation of viable units in lyophilized BCG danish 1331 vaccine. Biologicals. 2008;36(5):308–314. doi: 10.1016/j.biologicals.2008.05.001.
- Allen RC. In: DeLuca M, McElroy WD, editors. Bioluminescence and chemiluminescence. Chapter 4: "Bioluminescence Assays: Fundamentals and Applications". New York (NY): Academic Press; 1981. p. 63–73.
- Savov VM, Ismailov AD. [Biokhemiyluminestsentsiya]. Prikladniye vozmozhnosti v nauke i tekhnologii. Moskva: MGU; 1987.
- Askgaard DS, Gottschau A, Knudsen K, et al. Firefly luciferase assay of adenosine triphosphate as a tool of quantitation of viability of BCG vaccines. Biologicals. 1995;23(1):55–60. doi: 10.1016/1045-1056(95)90012-8.
- De Luca M, Mc Elroy WD. In: Colowick SP, Kaplan NO, editors. Chapter 18: "Bioluminescence and Chemiluminescence", Methods in enzymology. Vol. LVII, 3. New York (NY): Acаdemic Press; 1978.
- Gheoghiu M, Lagranderie M. Measure rapid de la viabilite’ du BCG par dosage de l’ATP. Ann Microbiol. 1979;130(2):11–19.
- Hoffner S, Jimenez-Misas C, Lundin A. Improved extraction and assay of mycobacterial ATP for rapid drug susceptibility testing. Luminescence. 1999;14(5):255–261. doi: 10.1002/(SICI)1522-7243(199909/10)14:5<255::AID-BIO543>3.0.CO;2-W.
- Janaszek W, Aleksandrowicz J, Sitkiewicz D. The use of the firefly bioluminescent reaction for rapid detection and counting of mycobacterium BCG. J Biol Stand. 1987;15(1):11–16. doi: 10.1016/0092-1157(87)90012-6.
- Kuzikov AN, Bondarenko VM, Latkin AT. Use of the bioluminescent method for the determination of bacterial adenosinetriphosphate (ATP-metry) in microbiology. Zh Microbiol Epidemiol Immunobiol. 2003;1:80–89.
- Maeda M. Determination of biological substances using bioluminescent reaction based on luciferin-luciferase. Rinsho Byori. 2004;52(7):595–603.
- WHO/TB/Technical Guide/77/9. In vitro assay of BCG products; 1977.
- Gu Q-S, Li T, Liu T, et al. Recent advances in design strategies and imaging applications of fluorescent probes for ATP. Chemosensors. 2023;11(7):417. doi: 10.3390/chemosensors11070417.
- Kolibab K, Derrick SC, Jacobs WR, et al. Characterization of an intracellular ATP assay for evaluating the viability of live attenuated mycobacterial vaccine preparations. J Microbiol Methods. 2012;90(3):245–249. doi: 10.1016/j.mimet.2012.05.015.
- Ugarova NN, Lomakina GY, Perevyshina TA, et al. Controlling BCG vaccine’s cell viability in the process of its production by an bioluminescent ATP assay. Moscow Univ Chem Bull. 2019;74(4):191–197. doi: 10.3103/S0027131419040084.
- Ugarova NN, Ugarova NN, Lomakina GY. Bioluminescence methods for the rapid assay of the specific activity of lyophilized BCG vaccine. Adv Tech Biol Med. 2017;5(4):243. doi: 10.4172/2379-1764.1000243.
- Jin TH, Qu T, Raina A, et al. Improvements of ATP assay as a substitute for the CFU method in estimating viable cell count for BCG/rBCG vaccine preparations. J Vaccines Vaccin. 2016;7(1):309. doi: 10.4172/2157-7560.1000309.
- Gweon E, Choi C, Kim J, et al. Development of a new approach to determine the potency of bacille Calmette-Guérin vaccines using flow cytometry. Osong Public Health Res Perspect. 2017;8(6):389–396. doi: 10.24171/j.phrp.2017.8.6.06.
- Moghawry HM, Rashed ME, Gomaa K, et al. Development of a fast and precise potency test for BCG vaccine viability using flow cytometry compared to MTT and colony-forming unit assays. Sci Rep. 2023;13(1):11606. doi: 10.1038/s41598-023-38657-x.
- Nafchi MZ, Hassanzadeh SM, Kaghazian H, et al. A modified ATP assay for test the potency of BCG vaccine. Pharma Pract. 2019;10(2):20–23.
- Ugarova NN, Lomakina GY, Modestova Y, et al. A simplified ATP method for the rapid control of cell viability in a freeze-dried BCG vaccine. J Microbiol Methods. 2016;130:48–53. doi: 10.1016/j.mimet.2016.08.027.
- Supaporn P, Sompong S, Sukanlayanee C. Suitability of alternative adenosine triphosphate potency assay for lot release of Tokyo bacilli Calmette-Guerin – 172-1 vaccines in Thailand. TJPS. 2018;42(4):203–207.
- Popescu C, Balazs D, Tasca G, et al. The preservation of viability of BCG vaccine freeze-dried in a new extracellular protector, evaluated by ATP-dependent bioluminescence assay. Cryo-letters. 1999;2:71–76.
- Savov VM. In: Willson B, Tuchin V, editors. Chapter 19: "Bioluminescence and Chemiluminescence in Early Warning Systems". Bioluminescence and chemiluminescence: mechanisms, fundamental principles and applications, advances in biophotonics, NATO science series; series I. Life and behavioral sciences. Vol. 369. Amsterdam; Berlin; Tokyo; Washington, DC: IOS Press; 2005. p. 228–241.
- Shi MF, Klegerman ME, Groves MJ. Viability of freeze-dried tice-substrain BCG by bioluminescent measurement of adenosine triphosphate. Microbios. 1989;59(240–241):145–155.
- Simpson WJ, Hammond JR. The effect of detergents on firefly luciferase reactions. J Biolumin Chemilumin. 1991;6(2):97–106. doi: 10.1002/bio.1170060207.
- Thore A, Anséhn S, Lundin A, et al. Detection of bacteriuria by luciferase assay of ATP. J Clin Microbiol. 1975;1(1):1–8. doi: 10.1128/jcm.1.1.1-8.1975.
- Wulff K. In: Bergmeyer HU, editor. Mehods of enzymatic analysis. 3rd ed. Vol. 1. Weinheim: Verlag Chemie; 1983. p. 340–368.
- WHO. Requirements for dried BCG vaccine. In: WHO Expert Committee on Biological Standardization, Annex 2. WHO technical report series 1987, No. 745; 1987. p. 60–92.
- Markey K, Ho MM, Rigsby P, et al. International collaborative study to evaluate and establish WHO reference reagents for BCG vaccine of three different substrains. Expert Committee on Biological Standardization; 2009 October 19–23; Geneva; WHO/BS/09.2114. Available from: https://iris.who.int/bitstream/handle/10665/70155/WHO_BS_09.2114_eng.pdf;jsessionid=D539401D08C3A41EEA8277AB3A1EDBA0?sequence=1