ABSTRACT
In this report, we investigated physiological and genetic aspect of RG5, a locus increasing the ratio of filled grain in rice (Oryza sativa L). RG5 increased the accumulation of non-structural carbohydrates (NSCs) in the body, and NSCs might contribute to the development of grains after heading. With a chromosome segment substitution line containing ‘Kasalath’ RG5 in the genetic background of the japonica cultivar ‘Koshihikari’, RG5 might enlarge lower leaves and improve NSC supply to the sheath. Through the fine mapping using data plural mutans, we identified NBS-LRR protein (Os05g0380300) as a casual gene for expanding of lower leaves area by RG5. The single nucleotide polymorphism (SNP) of Kasalath caused a premature stop codon and lost function might increase the ratio of filled grains.
KEYWORDS:
The ratio of filled grains, the main factor of grain yield in rice (Oryza sativa L.), is determined by the relative ability of source to sink size. In rice, more than 30 genes related to sink size, such as grain number or weight, have been identified (Fan et al., Citation2006; Song et al., Citation2007; Qi et al., Citation2012; Mao et al., Citation2010; Ishimaru et al., Citation2013). The expansion in sink size has been achieved with genetic marker selection or genome editing methods. In most modern cultivars, source ability is equal to, or rather lower than, sink size. We must increase rice production under low inputs of fertilizer to meet the increasing demand in view of the future population growth and achieve the United Nations’ sustainable development goals (SDGs); therefore, the improvement of source ability has been the main target of research aiming to enhance yield, especially under low inputs.
In addition to photo-assimilate after heading, rice has another source, which originates from the non-structural carbohydrates (NSCs) mainly starch accumulated in leaf sheaths and culms prior to heading (Ishimaru, Citation2003). With the functional differentiation in a sheath, the lower part accumulated most starch and upper part-functioned translocation of carbohydrates from the connected leaf blade to the lower part.
The protein level of branching enzyme I (BEI, EC 2.4.1.18) and sucrose synthase (SuS, EC 2.4.1.13) were consistent with the content of starch in a leaf sheath (Ishimaru et al., Citation2004), and their activities were regulated at the transcriptional level (Hirose et al., Citation1999, Citation2006). In rice leaf sheaths, SuS played a central role in the degradation of sucrose to hexoses. Among the enzymes involved in starch synthesis, BEI enzymes were shown to have the highest positive correlation with starch content and controlled activities at the transcriptional level.
In a previous report, we identified a locus for the higher ratio of filled grains (RG5) with positive allele from aus rice, ‘Kasalath’ in ‘Nipponbare’ background (Ishimaru et al., Citation2005). This allele increased starch content in a body before heading at 30% and, consequently, increased yield at 15%. Additionally, RG5 could function across genetic background. On the Koshihikari background, the ratio of filled grain was higher than that of the control without negative effect on other yield component, grain number, grain size and weight.
Increasing the amount of NSCs in leaf sheaths before heading has been important for improving yield. Some genes related to starch accumulation in rice were identified by Hirose et al. (Citation1999, Citation2006), but the genetic targets for increasing accumulation remained unknown. As a first step, this study analyzed the physiological factors that increased NSCs accumulation by RG5 and attempted to identify the genes involved.
Materials and methods
Plant materials
We used SLRG5, (CSSL212), which carries a 20 cM chromosomal segment derived from Kasalath around RG5 in the ‘Koshihikari’ genetic background. The seedlings used in this study were sown in a greenhouse on 27 April 2017, and were transplanted to paddy fields in Tsukuba, Japan (36.05’140.055”), on 27 May 2017; one plant per hill at a spacing of 18 × 30 cm. Twenty-five plants were grown for each strain. Compound fertilizer (N: P2O5: K2O = 14: 14: 14%) and molten phosphoric acid (P2O5 = 20%) were applied as base dressing. Insertion mutants of Tos17 were selected by BLAST searches against a dataset of Tos17 flanking sequences in the rice genome (Miyao et al., Citation2003; Mutant panel (affrc.go.jp). The length and leaf area of lower (third) leaf blade of SLRG5 was measured in 5 plants in each line. At just before heading, a third of leaf sheathes were sampled in liquid nitrogen after separated into 5 parts and used for measurements.
Determination of starch content and real-time PCR
Starch content was measured enzymatically based on the method by Ono et al. (Citation1999). The extract of genome DNA or mRNA was performed with DNeasy Plant Mini Kit (Qiagen, USA) and RNeasy Plant Mini Kit, respectively. Similarly, the real-time PCR method was operated using gene specific primers. In real-time PCR, the relative expression levels were calculated using the 2−ΔΔCT method (Livak and Schmitthen, Citation2001). The used primers were listed in supplemental Table 3.
Fine mapping of RG5 and haplotype analysis
DNA markers were created at both ends of the RG5 candidate region, and lines with recombination within the region were selected from the 1,920 individuals. Homozygous lines were selected from the progeny of these lines by self-fertilization and cross-fertilization; they were grown in paddy fields in the region where RG5 was present based on the results of phenotype and genotype. Additionally, previous studies and databases were used to narrow down the candidate genes to one. Haplotype of RG5 was analyzed with rice varmap (http://ricevarmap.ncpgr.cn). The primers were listed in supplemental table 3.
Results and discussion
We analyzed physiological function for higher starch accumulation by RG5. Two hypotheses were considered regarding the function of RG5 in increasing the starch content of leaf sheaths: (1) the function of the upper part might be altered to store starch and (2) the content of the lower part might be increased without any functional change (). We divided the third leaf sheath of ‘Koshihikari’ and SLRG5 plants into five parts and measured each accumulation (). In both plants, the content consistently increased from top to bottom. The slope of the regression line of the SLRG5 was larger than that of the control, Koshihikari. This result implied increased accumulation in every part of the leaf sheath. The rate of increase in starch content due to RG5 increas showed from the bottom to the top, with a 2.3-fold increase at the bottom, but a 3.0-fold increase at the 3rd, 6.3-fold increase at the 2nd, and 11.2-fold increase at the top of the sheath (dnata not show). On the other hand, the lower part of the leaf sheath still accumulated more and the upper part less; in fact, the accumulation in the upper part was 20% of that in the lower part in the SLRG5.
Figure 1. (a) Two hypothetical models for high starch content by RG5. Two hypotheses were consideredregarding the function of RG5 (1) the upper part might be altered to be sink, and (b) the sink capacity of thelower part might be improved. (a) and the manner of accumulated starch in Koshihikari (white box) andSLRG5 (black box) (b). Third leaf sheath was separated into 5 parts and used. The contents of starch weresignificant different in all parts between them (p <0.001).
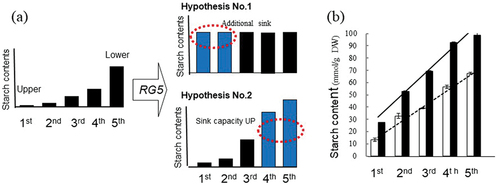
The expression level of BEI and SuS were consistent with the content of starch in a leaf sheath (Hirose et al., Citation1999, Citation2006), and their activities were regulated at the transcriptional level. In third leaf sheath of SLRG5 plants, the expression levels of these two genes were similar to those in the control (). Thus, the increasing accumulation associated with RG5 was not due to a functional change of the upper non-accumulating site to an accumulating site, but to an increasing accumulation of starch in each site. These results suggested that the increase in NSC accumulation associated with RG5 was not due to an increasing accumulation capacity but to other factors, such as increased supply ability. In fact, the area of the leaf blade connected to the leaf sheath in SLRG5 was significantly larger than that in Koshihikari, by more than 30% ().
Figure 2. (a) Relative expression of key genes for starch synthase in 5th (lower) part of third sheath and (b)third leaf area of Koshihikari (white box) and SLRG5 (black box). BE1:branching enzyme 1; SuS: sucrose synthase. *means P<0.05
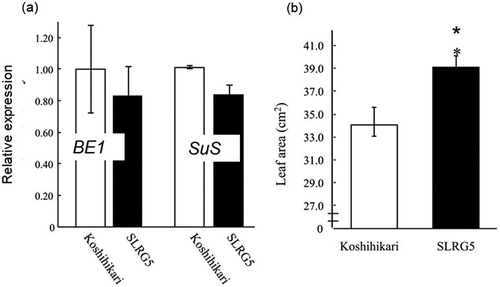
Based on the ratio of filled grains and genotypes, the chromosomal region containing RG5 was narrowed down to 250 kb at 18.15–18.49 Mb on chromosome No. 5 (). DNA markers (No. 902, 834) were made at both ends of the RG5 candidate region (410Kb), and seven lines with recombination in the region were selected from 1,920 individuals (). The grain number per a panicle as sink capacity affected on the ratio of filled grain. This ratio of 4-D-6 was significantly higher than that of Koshihikari was caused by lower grain number per a panicle.
Figure 3. Fine mapping of RG5 in rice. Black and white line mean chromosome of Kasalath andKoshihikari, respectively. In phenotype data, blue box indicated grain number per a panicle.Gray box did the ratio of filled grain (%) without 3-E-6.All markers were listed in supplemental table 3.
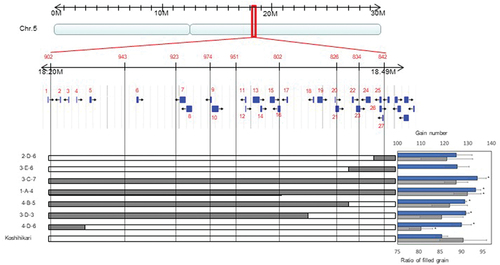
The annotation data of the rice genome suggested that 27 genes (0s05g0377150~Os05g0382550) were present in the candidate region for RG5 (Supplemental table 3). By the comparison of annotation data of the Koshihikari and Kasalath, single nucleotide deletion caused stop codons (non-sense) in Os05g0380300 (similar to the NBS-LRR protein). According to the expression database (https://ricexpro.dna.affrc.go.jp), the expression of Os05g0380300 (Transcript ID: Os05g31570-1) was high in early developing stage of leave. To clarify this point, we measured leaf area of pickup NILs (Supplemental table 2). In two knockout lines (NC3065, ND6035) with Tos in the Nipponbare background, the 3rd leaves were larger than the control (Supplemental table 2). Therefore, Os05g0380300 should be the causal gene at least for expanding in larger lower leaf area.
Overall, we decided that Os05g0380300 was the casual gene for expanding in lower leaf and principal candidate gene for RG5 and that the loss of function due to the SNP might be the determining factor (FNP) for the allele. Jun et al. (Citation2019) reported that the knockout line with Tos17 inserted on the exon of Os05g0380300 had 56% higher grain weight than the control, Korean variety ‘Ilmibyo’ (japonica). They measured grain weight without sorting, so the data are probably given by a higher proportion of filled grains, as in Kasalath allele RG5. On the contrary, the overexpression of Pi-d2 and Pi-d3 genes, which belong to the NBS-LRR protein reduced the grain weight per plant was reduced by 7.7% and 29.6%, respectively (Hao et al., Citation2009). While further study is needed with transgenic plants overexpressed of RG5 in Kasalath to clarify this point.
Haplotype analysis with rice varmap indicated that the distribution of SNPs in 4, 726 cultivars that confer the allelic properties of RG5 possessed by Kasalath allele. Rice (O. sativa) can be divided into five genetically differentiated subgroups based on genome sequence: indica, aus, aromatic, temperate japonica, and tropical japonica (Song et al., Citation2007). The FNP for Kasalath allele RG5 is cassalate in all subgroups except for aus. Less than 10% of the varieties contain Kasalath allele RG5 (), while 82.2% of the aus 276 varieties had this FNP. This rice variety was originally grown under rainfed conditions during short summers in Bangladesh. As NSCs accumulate over a long period and were less sensitive to environmental factors, their contribution might become more important under unfavorable conditions such as short summers.
Table 1. Population of Koshihikari and Kasalath type allele in rice.
In conclusion, the results in this study indicated that improving source ability not only of the uppermost flag leaves, but also of the canopy, might be effective in achieving higher ratio of filled grain and increasing yields. One of the strategies was to increase the size and photosynthetic ability of the lower leaves, but it remains unknown how the mechanism of this gene (NBS-LRR protein) might be associated with leaf elongation and the ratio of filled grain. In addition to expanding the area of third leaves, which accumulated the maximal amount of carbohydrates, maintaining the photosynthetic ability (i.e. improving light-receiving conditions) was considered as a target to improve accumulation of NSCs and source ability.
Supplemental Material
Download Zip (26.1 KB)Disclosure statement
No potential conflict of interest was reported by the author(s).
Supplementary material
Supplemental data for this article can be accessed online at https://doi.org/10.1080/1343943X.2024.2330729
Correction Statement
This article has been republished with minor changes. These changes do not impact the academic content of the article.
Additional information
Funding
References
- Fan, C., Xing, Y., Mao, H., Tingting, L., Han, B., Caiguo, X., Xianghua, L., & Zhang, Q. (2006). GS3, a major QTL for grain length and weight and minor QTL for grain width and thickness in rice, encodes a putative transmembrane protein. Theoretical and Applied Genetics, 112, 1164–1171.
- Hao, Z., Wang, J., Wang, L., & Tao, R. (2009). Influences of the disease resistance conferred by the individual transgenes, pi-d2, pi-d3 and Xa21, on the transgenic rice plants in yield and grain quality. African Journal of Biotechnolgy, 8, 4845–4848.
- Hirose, T., Endler, A., & Ohsugi, R. (1999). Gene expression of enzymes for starch and sucrose metabolism and transport in leaf sheaths of rice (Oryza sativa L.) during the heading period in relation to the sink to source transition. Plant Production Science, 2, 178–183.
- Hirose, T., Ohdan, T., Nakamura, Y., & Terao, T. (2006). Expression profiling of genes related to starch synthesis in rice leaf sheaths during the heading perio. Physiologia plantarum, 128(3), 425–435. https://doi.org/10.1111/j.1399-3054.2006.00758.x
- Ishimaru, K. (2003). Identification of a locus increasing rice yield and physiological analysis of its function. Plant Physiology, 133(3), 1083–1090. https://doi.org/10.1104/pp.103.027607
- Ishimaru, K., et al. (2005). Identification and physiological analyses of a locus for rice yield potential across the genetic background. Journal of Experimental Botany, 56(420), 2745–2753. https://doi.org/10.1093/jxb/eri267
- Ishimaru, K., Hirotsu, N., Madoka, Y., Murakami, N., Hara, N., Onodera, H., Kashiwagi, T., Ujiie, K., Shimizu, B.-I., Onishi, A., Miyagawa, H., & Katoh, E. (2013). Nature Genet. Nature Genetics, 45(6), 707–711. https://doi.org/10.1038/ng.2612
- Ishimaru, K., Kosone, M., Sasaki, H., & Kashiwagi, T. (2004). Leaf contents differ depending on the position in a rice leaf sheath during sink–source transition. Plant Physiology & Biochemistry, 42(11), 855–860. https://doi.org/10.1016/j.plaphy.2004.10.008
- Jun, K. M., et al. (2019). App. Biological Chemistry, 62(1), 31. https://doi.org/10.1186/s13765-019-0439-z
- Livok, K., & Schnittogen, T. D. S. (2001). Analysis of Relative Gene Expression Data Using Real-Time Quantitative PCR and the 2‒ΔΔCT Method. Methods, 25(4), 402–408. https://doi.org/10.1006/meth.2001.1262
- Mao, H., Sun, S., Yao, J., Wang, C., Yu, S., Xu, C., Li, X., & Zhang, Q. (2010). Linking differential domain functions of the GS3 protein to natural variation of grain size in rice. Proceedings of the National Academy of Sciences, 107(45), 19579–19584. https://doi.org/10.1073/pnas.1014419107
- Miyao, A., Tanaka, K., Murata, K., Sawaki, H., Takeda, S., Abe, K., Shinozuka, Y., Onosato, K., & Hirochika, H. (2003). Target site specificity of the Tos17 retrotransposon shows a preference for insertion within genes and against insertion in retrotransposon-rich regions of the genome. The Plant Cell, 15(8), 1771–1780.
- Ono, K.,Ishimaru, K., Aoki, N., Takahashi, S., Ozawa, K., Okawa, Y., & Ohsugi, R. (1999). Characterization of a maize sucrose–phosphate synthase protein and its effect on carbon partitioning in transgenic rice plants. Plant Production Science, 2, 172–177. https://doi.org/10.1626/pps.2.172
- Qi, P., Lin, Y.-S., Song, X.-J., Shen, J.-B., Huang, W., Shan, J.-X., Zhu, M.-Z., Jiang, L., Gao, J.-P., & Lin, H.-X. (2012). The novel quantitative trait locus GL3.1 controls rice grain size and yield by regulating Cyclin-T1;3. Cell Research, 22(12), 1666–1680. https://doi.org/10.1038/cr.2012.151
- Song, X. J., Huang, W., Shi, M., Zhu, M.-Z., Lin, H.-X. (2007). A QTL for rice grain width and weight encodes a previously unknown RING-type E3 ubiquitin ligase. Nature Genetics, 39(5), 623–630. https://doi.org/10.1038/ng2014