ABSTRACT
CO2-Responsive CCT Protein (CRCT) is a transcription factor regulating the expression of starch synthesis-related genes in the vegetative organs of rice. Among six rice cultivars (Nipponbare, Koshihikari, Habataki, Takanari, Momiroman, Leafstar), the expression of CRCT was highly correlated with the starch content of leaf sheath in Nipponbare, but not in Koshihikari, Takanari and Momiroman during plant development. Meanwhile, the expressions of starch synthesis-related genes such as ADP-glucose pyrophosphorylase large subunit 1 was correlated with CRCT expression in Nipponbare, Momiroman, and Leafstar, but the correlations were ambiguous in Koshihikari, Habataki, and Takanari. In addition, the slopes of its regression lines in Nipponbare were larger than those cultivars. These results suggest that the influence of CRCT on starch synthesis varies among these cultivars. Additionally, the similar cultivar differences were observed when these gene expressions were analyzed after sucrose treatment in leaf blade. Some mutations in the promoter regions of the starch synthesis-related genes were identified among these cultivars. There were cultivars in which the presence of mutations correlated with decreased expression of starch synthesis genes in response to CRCT. Thus, these mutations can partially explain the responsiveness of the starch synthesis-related genes to CRCT, and difference in starch content of vegetative organs among rice cultivars.
1. Introduction
Starch is a major photosynthetic product and utilized for transient carbon storage in vegetative organs such as a leaf blade and a leaf sheath. At the end of the vegetative stage, starch is highly accumulated in the leaf sheath of rice. During the ripening stage, the carbohydrate stored as this starch is translocated to grains. As much as 30% of grain carbon is derived from this carbon translocation (Cock & Yoshida, Citation1972), which is hugely important for normal ripening under low irradiance (Okamura et al., Citation2013). Thus, improving starch accumulation in the leaf sheath is important to save grain yield and quality in rice.
Previously, we identified CO2-responsive CCT Protein (CRCT) through screening CO2-responsive genes by microarray (Fukayama et al., Citation2009; Morita et al., Citation2015). In the leaf sheath, the starch content of CRCT overexpression lines was markedly higher than that of wild-type while the starch content of CRCT knock-down lines and CRCT knock-out lines was significantly lower (Miyagawa et al., Citation2023; Morita et al., Citation2015). The expressions of several starch synthesis-related genes such as ADP glucose pyrophosphorylase large subunit 1 (OsAGPL1) was highly correlated with the expression of CRCT. CRCT can binds to the promoter regions of these starch synthesis-related genes (Fukayama et al., Citation2021). These results indicated that CRCT is a transcription factor regulating starch synthesis by promoting the expressions of starch synthesis-related genes. CRCT is significantly upregulated under elevated CO2 condition and also responds to sucrose to promote the expressions of starch synthesis-related genes (Miyagawa et al., Citation2023). Given that photosynthesis increases the accumulation of sucrose under elevated CO2 condition, CRCT is considered to be a key regulator of starch synthesis in the vegetative organs responding to the level of photosynthate.
In this way, the importance of CRCT in controlling starch synthesis has become clear using a rice cultivar, Nipponbare. It is well-known that starch accumulation in the vegetative organs differs depending on the cultivar, whereas the relationship between this phenomenon and CRCT is unknown yet. In this study, we selected six rice cultivars (Nipponbare, Koshihikari, Habataki, Takanari, Momiroman, Leafstar), which showed different patterns of starch accumulation in vegetative organs during growth, and analyzed the expressions of CRCT and starch synthesis-related genes, and starch content at various developmental stages. Also, we analyzed the cultivar differences in responsiveness of expression of CRCT and starch synthesis related genes to sucrose. These results and analysis of promoter sequence of starch synthesis related genes suggest that the responsiveness of the promoters of starch synthesis-related genes to CRCT may determine the expression levels of these genes in some cultivars, and hence it partially explains the cultivar difference in starch accumulation of vegetative organs.
2. Materials and methods
2.1. Plant materials and growth condition
The six rice cultivars (Oryza sativa L. cv., Nipponbare, Koshihikari, Habataki, Takanari, Momiroman, Leafstar) were grown in paddy soil under natural light and temperature conditions in a net house. Two rice seedlings at 4.5 leaf stage were transplanted into 1/5000 Wagner pots supplemented with 0.6 g chemical fertilizer (N:P:K = 8:8:8), 1.0 g slow-release fertilizer (LP COTE 70, JCAM AGRI, Tokyo, Japan), 0.4 g micronutrient (High green, MC FERTICOM, Tokyo, Japan) and 4.0 g silicate fertilizer (Softsilica, Tokyo, Japan) per pot. The leaf sheaths were sampled at 18:00–19:00 (photoperiod of 5:00–19:00) on sunny days from the developmental stage (8th and 9th leaf sheaths at 9.5 leaf stage) and the reproductive stages (2nd and 3rd leaf sheath from flag leaf sheaths at 0, 10 and 20 days after heading), immediately frozen in liquid nitrogen and stored at −80°C until required.
For the first sucrose treatment, the rice seedlings were transplanted into 1 L pots supplemented with 2.0 g chemical fertilizer (N:P:K = 8:8:8), 0.5 g slow-release fertilizer, 0.4 g micronutrient and 1.5 g silicate fertilizer. These plants were grown under natural light and temperature in a greenhouse. One month after transplanting, these plants were placed in darkness for one day. Then, the second leaf blades from the top of the main culms were detached at 10:00 and immediately immersed in water or 50 mM sucrose solution in the dark at 25°C. After 12 and 24 h, the treated leaf blades were frozen in liquid nitrogen and stored at −80°C until required. For the second sucrose treatment, Nipponbare and Momiroman were grown as above and placed in darkness for two days. Then, the leaf blades were sampled at 11:00 and immediately immersed in water or 50, 100, or 150 mM sucrose solution in the dark at 25°C. After 24 h, the treated leaf blades were frozen and stored as above.
2.2. Determination of starch content
The starch in the leaf sheath was extracted and solubilized as described previously (Morita et al., Citation2015). The starch content was determined by a coupled enzyme assay using an ENZYTEC Starch kit (E1268, r-biopharm, Darmstadt, Germany) as described previously (Fukayama et al., Citation2021).
2.3. qRT-PCR analysis
The total RNA was isolated from rice tissues using RNeasy Plant Mini Kit (Qiagen, Hilden, Germany) according to the manufacturer’s instructions. The first strand cDNA was synthesized from the total RNA with an oligo (dT)18 and a random hexamer as primers using PrimeScript II first Strand cDNA Synthesis Kit (Takara, Kyoto, Japan). The qRT-PCR was carried out using the gene-specific primers shown in Supplemental Table 1, TBgreen Premix Ex Tag GC (Takara), and a thermal cycler (MyGoPro, IT-IS Life Science, Dublin, Ireland). The expression of genes relative to Actin (internal control) was calculated by the delta-delta Ct method.
The priming site sequences of all primers used in this study were analysed by genome-wide variation data of 685 rice varieties, TASUKE+ for RAP-DB (https://agrigenome.dna.affrc.go.jp/tasuke/ricegenomes/). Although this database does not include Habataki, Momiroman and Leafstar, there are no mutations in the priming sites of starch synthesis related genes among the varieties in Oryza sativa. However, according to TASUKE+, the mutation was found in the priming site of forward primer of Actin (OsRac1-F2) in some varieties other than Nipponbare, Koshihikari and Takanari. Therefore, we determined the nucleotide sequence of the priming site of OsRac1-F2 in Habataki, Momiroman and Leafstar by ourselves and confirmed that there are no mutations in this region in these varieties. Therefore, we consider that PCR efficiency is unlikely to vary between six cultivars used in this study.
2.4. Analysis of nucleotide sequences of promoters
Genomic DNA was extracted from leaf blades basically as described in Liu et al. (Citation1995). As an exception, we used modified DNA extraction buffer (50 mM Tris-HCl (pH7.5), 0.3 M NaCl, 20 mM EDTA, 10 mM mercaptoethanol, 5 M urea, 0.5% (w/v) SDS, 5% (v/v) phenol). Promoter regions of starch synthesis genes were amplified by PCR using Quick Taq HS DyeMix (TOYOBO, Osaka, Japan) and the primers (listed in Supplemental ). The PCR products were purified using ISOSPIN PCR Product or ISOSPIN Agarose Gel (NIPPON GENE, Tokyo, Japan). The nucleotide sequences of these PCR products were determined by contract analysis (Azenta, Tokyo, Japan). Cis-elements were analyzed using New PLACE database (https://www.dna.affrc.go.jp/PLACE/?action=newplace).
Figure 1. Starch content and starch synthesis-related gene expressions in the leaf sheathes of six rice cultivars. (a) The starch content and starch synthesis-related gene expressions at various developmental stages. Rice leaf sheathes were sampled in the evening of vegetative stage (VGS, 8.5 leaf stage) and reproductive stages of 0, 10 and 20 days after heading (DAH). The expressions of starch synthesis related genes relative to Actin analyzed by qRT-PCR are shown. Data represent mean ± SD of four to five biological replicates. (b) The correlation between starch content and starch synthesis-related gene expressions as a function of CRCT expression. Data were obtained from the experiment of .
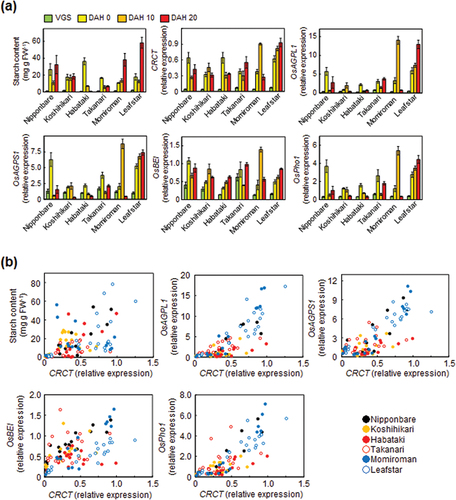
Table 1. Slopes of the liner regressions and its correlation coefficients of the relationship between the expressions of CRCT and starch synthesis related genes in leaf sheathes during development.
2.5. Statistical analysis
The significance of difference in expression between sucrose treatment and H2O treatment was analyzed by a Student’s t-test. The significance of difference in induction of starch synthesis-related genes among culativars was analyzed by Tukey’s test. The regression lines were created using the least squares method, and the correlation coefficient was calculated. The level of significance was set at for p < 0.05 for Student’s t-test and Tukey’s test, and p < 0.01 for correlation coefficient.
3. Results and discussion
3.1. Expressions of CRCT and starch synthesis-related genes in the leaf sheath
The starch content in the rice leaf sheath varies dynamically with developmental stages, and it is considerably different among rice varieties (Goda et al., Citation2016; Hirano et al., Citation2005). CRCT is a key regulator of starch accumulation by controlling the expression of starch synthesis-related genes in rice leaf sheath (Morita et al., Citation2015). From these findings, we assume that CRCT can determine changes in the starch content in rice leaf sheath throughout developmental stages, and differences in the starch contents among cultivars. To test this hypothesis, we analyzed the expressions of CRCT and starch synthesis-related genes by qRT-PCR, and measured the starch contents in the leaf sheaths of the six rice cultivars (Nipponbare, Koshihikari, Habataki, Takanari, Momiroman, Leafstar) at various developmental stages. These six rice cultivars are representative or have different starch accumulation patterns (Fukayama et al., Citation2021). Sugimura et al. (Citation2015) reported that the starch content in the leaf sheathes peaked at the heading stage in Nipponbare and Takanari. Thus, the leaf sheathes were sampled at 0, 10 and 20 days after heading in addition to vegetative stage (8.0 leaf stage).
In all the six rice cultivars, the expressions of CRCT at the reproductive stages were markedly higher than that at the vegetative stage (). Also, the expressions of starch synthesis-related genes and the starch contents after heading were higher than those at the vegetative stage. Photosynthetic products such as sucrose positively regulate CRCT and nitrogen negatively affects the expression of CRCT (Morita et al., Citation2015). At the vegetative stage, the sink strength for growth is large and nitrogen would be sufficient. After heading, the main sink gradually shifts to the panicle from vegetative organs, and nitrogen becomes limited. It is possible that physiological changes such as the balance of carbon and nitrogen may influence the differences in expression of CRCT between the reproductive stages and vegetative stage. Either way, changes in the expression of CRCT seem to affect the expression level of starch synthesis related genes and the starch accumulation during growth of rice.
In Habataki, the expressions of CRCT, OsAGPL1 and OsAGPS1 were decreased at 10 and 20 days after heading (DAH), and the starch content also decreased over the same period. In Leafstar, the starch content as well as the expressions of CRCT and starch synthesis-related genes increased during reproductive stage and reached its maximum at DAH 20. Also, in Nipponbare and Koshihikari, the patterns of change in the starch content were similar to those of CRCT expression. From these results, it is suggested that CRCT is involved in changes in the starch accumulation in the leaf sheath during plant development of these cultivars. However, the starch content of Momiroman continued to increase even at DAH 20 although the expressions of CRCT and starch synthesis-related genes were significantly decreased at that time. In contrast, the starch content of Takanari was quite low at DAH 20 although the expressions of CRCT and starch synthesis-related genes were kept at high level. These results suggest that factors other than control of starch synthesis by CRCT, possibly starch degradation can contribute to starch content in some cultivars (Sugimura et al., Citation2015).
The scatter plots showed that the starch content and the expressions of starch synthesis-related genes were basically correlated with CRCT expression, but the degree of the correlations differed among cultivars (). Overall, the slopes of the regression lines and the correlation coefficient in Koshihikari, Takanari and Habataki tended to be lower than Nipponbare (). Also, the slope in Leafstar was slightly lower than that in Nipponbare. Thus, it is assumed that there may be cultivar differences in the sensitivity of starch synthesis-related genes to CRCT.
3.2. Response of expressions of CRCT and starch synthesis-related genes to sucrose
In our previous study, it was shown that CRCT responds to sucrose to promote the expressions of starch synthesis-related genes (Miyagawa et al., Citation2023). In this experiment, we used leaf blades rather than leaf sheathes from which starch can be easily removed by dark treatment before starting sucrose treatment. Thus, sucrose dependent inductions of CRCT and starch synthesis-related genes were analyzed using the detached leaf blades of the six rice cultivars. The expression of CRCT was basically upregulated by sucrose treatment in most cultivars (). These results were largely consistent with previous reports using Nipponbare (Miyagawa et al., Citation2023; Morita et al., Citation2015). However, in Momiroman, the expression was high even without sucrose treatment, and thus the increase in expression was unclear. In order to confirm whether CRCT in Momiroman really respond to sucrose or not, we conducted the second experiment under conditions that make it more likely to respond to sucrose, namely, longer dark treatment and higher sucrose concentrations. In this experiment, the expression of CRCT was upregulated by 24 h sucrose treatment in Momiroman (Supplemental Figure 1). Overall, even without sucrose treatment, the expression of CRCT tended to be high in Momiroman, especially at 12 h. CRCT belong to CCT protein, some of which is controlled by circadian rhythm (Li & Xu, Citation2017; Valverde, Citation2011). The CRCT expression in the leaf blade of Nipponbare increased during the daytime and decreased during the night period (Fukayama et al., Citation2021). Most of these changes in expression are considered to be in response to sugar levels, but can be partially influenced by circadian rhythms. It is hypothesized that Momiroman is more susceptible to circadian rhythm than other cultivars. This cultivar difference is interesting, and its mechanism awaits clarification.
Figure 2. Effects of sucrose on the expression of starch synthesis-related genes in the leaf blades of the six rice cultivars. Seventh leaf blades at 7.2 leaf stage were immersed in water (H2O) or 50 mM sucrose solution (Suc). The expressions of CRCT and starch synthesis-related genes (OsAGPL1, OsAGPS1, OsBEI, and OsPho1) relative to Actin analysed by qRT-PCR are shown. The data represents the means ±SD of four independent samples. Asterisks indicate significant differences between a water-treated group and a sucrose-treated group for the same hour and cultivar by the Student’s t-test (*p < 0.05).
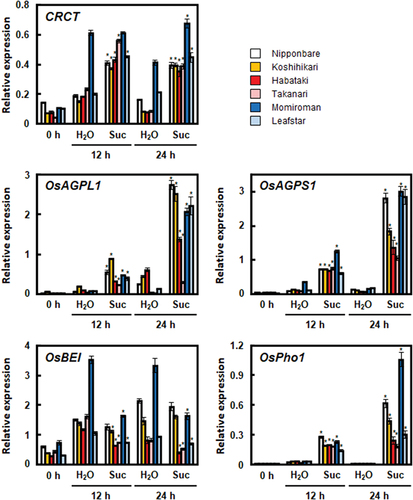
As well as CRCT, the expressions of starch synthesis-related genes except for OsBEI were basically upregulated by sucrose treatment in all cultivars (). However, we observed cultivar difference in the response of CRCT and starch synthesis-related genes to sucrose. In detail, the expressions of OsAGPL1 under sucrose treatment in Habataki and Takanari were lower than that in Nipponbare even though there were no obvious difference in the expression of CRCT among these three cultivars. The expressions of OsAGPS1 in Koshihikari, Habataki and Takanari at 24 h sucrose treatment were lower than those in the other cultivars. The expressions of OsPho1 in Koshihikari, Habataki, Takanari and Leafstar at 24 h sucrose treatment were lower than those in Nipponbare and Momiroman. Next, we compared the inductions of starch synthesis ralated genes by sucrose relative to CRCT among cultivars and tested for significant differences between cultivars (Supplemental Figure 2). The inductions of OsAGPL1, OsAGPS1 and OsPho1 in 24 h were significantly lower in Koshihikari, Habataki, Takanari than those in Nipponbare. The scatter plot highlighted that the correlations between the expressions of CRCT and starch synthesis-related genes after sucrose treatment differed among the rice cultivars although these correlations is unclear compared to (Supplemental ). Similar to , the slopes of the regression lines of Habataki and Takanari in OsAGPS1 and OsPho1 tended to be lower than that of Nipponbare (). Therefore, these cultivar differences in sucrose dependent induction of starch synthesis-related genes by CRCT may also suggest the presence of the difference in sensitivity of starch synthesis-related genes to CRCT.
Table 2. Slopes of the linear regressions and its correlation coefficients of the relationship between the expressions of CRCT and starch synthesis related genes in leaf blades after sucrose treatment.
3.3. Nucleotide sequences of the promoter regions of starch synthesis-related genes among rice cultivars
Previously, we estimated the promoter regions of starch synthesis-related genes to which CRCT directly binds by ChIP analysis in Nipponbare (Fukayama et al., Citation2021). In this study, the nucleotide sequences of these regions of the six cultivars were determined (, Supplemental Figure 4). The comparison of cis-elements in these nucleotide sequences identified 3, 9, 3 and 21 mutations in OsAGPL1, OsAGPS1, OsBEI and OsPho1 among six cultivars, respectively (, Supplemental Table 2). There were few mutations in regions other than cis-elements, with 0, 1, 1 and 7 mutations in OsAGPL1, OsAGPS1, OsBEI and OsPho1, respectively. Particularly, the mutations were found in the cis-element T1ME of TOC1 (Gendron et al., Citation2012), a representative CCT protein in the OsAGPL1 promoter of Habataki and in the OsAGPS1 promoter of Takanari (compared to Nipponbare). Also, the mutations in the cis-element of Ghd7 (G-box, Nemoto et al., Citation2016), a CCT protein, were found in the OsAGPS1 promoter of Takanari and the OsBEI promoter of Takanari, Habataki and Leafstar. Interestingly, the slopes of the regression lines of the correlations of CRCT expression to starch synthesis-related genes expressions in Habataki and Takanari were lower than that in Nipponbare, suggesting a causal relationship with these mutations. Thus, it is possible that these mutations of promoter regions of starch synthesis-related genes may relate to the sensitivity of starch synthesis-related genes to CRCT in some cultivar such as Takanari. However, it is also possible that cis-elements responsible for this sensitivity may present outside of CRCT-binding site sequenced in this study. Actually, Koshihikari is also considered to be less sensitive, but no mutations were found in this study. In addition, factors other than cis-elements of CRCT may be involved in this mechanism, which remains to be elucidated.
Figure 3. Comparison of nucleotide sequence of OsAGPL1 promoters. (a) Diagram of OsAGPL1 promoter in Nipponbare. Amplified positions in previous ChIP-qPCR analysis (Fukayama et al., Citation2021) are shown in pink and red bar. Especially, the position enriched by ChIP are shown in red bar. The position amplified by PCR for sequence analysis are shown in green bar. (b) Mutations in cis-elements of OsAGPL1 promoters among six cultivars. Cis-elements which have nucleotide mutations compared to Nipponbare are listed, and mutated nucleotide is coloured in red. The nucleotide sequences of cis-elements are underlined. Position is based on the numbers from the translation initiation site.
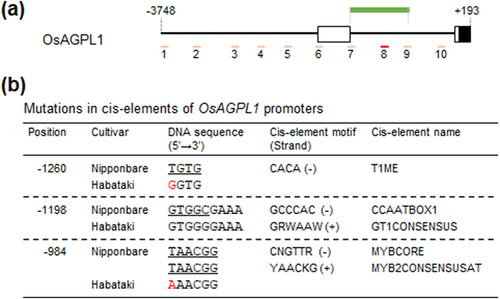
Concluding remarks
In this study, we indicated that responsiveness of expressions of starch synthesis-related genes to CRCT differed among rice cultivars during plant growth and sucrose treatment. This difference can be due to different sensitivity of starch synthesis-related genes with CRCT. The mutations found in the promoters of starch synthesis-related genes may involve in this sensitivity in some cultivars. To exploit cis-elements determining the sensitivity will help us to improve starch accumulation by genome editing and breeding, and finally productivity and quality of rice in the future.
Supplemental Material
Download MS Excel (18.6 KB)Supplemental Material
Download PDF (210.5 KB)Acknowledgments
We thank Prof. Taiichiro Ookawa (Tokyo University of Agriculture and Technology) for generous gifts of the seeds of Leafstar.
Disclosure statement
No potential conflict of interest was reported by the author(s).
Supplementary material
Supplemental data for this article can be accessed online at https://doi.org/10.1080/1343943X.2024.2344833.
Correction Statement
This article was originally published with errors, which have now been corrected in the online version. Please see Correction (http://dx.doi.org/10.1080/1343943X.2024.2354572)
Additional information
Funding
References
- Cock, J. H., & Yoshida, S. (1972). Accumulation of 14C-labelled carbohydrate before flowering and its subsequent redistribution and respiration in the rice plant. Japanese Journal of Crop Science, 41(2), 226–234.
- Fukayama, H., Fukuda, T., Masumoto, C., Taniguchi, Y., Sakai, H., Cheng, W., Hasegawa, T., & Miyao, M. (2009). Rice plant response to long term CO2 enrichment: Gene expression profiling. Plant Science, 177(3), 203–210. https://doi.org/10.1016/j.plantsci.2009.05.014
- Fukayama, H., Miyagawa, F., Shibatani, N., Koudou, A., Sasayama, D., Hatanaka, T., Azuma, T., Yamauchi, Y., Matsuoka, D., & Morita, R. (2021). CO2-responsive CCT protein interacts with 14-3-3 proteins and controls the expression of starch synthesis-related genes. Plant Cell and Environment, 44(8), 2480–2493. https://doi.org/10.1111/pce.14084
- Gendron, J. M., Pruneda-Paz, J. L., Doherty, C. J., Gross, A. M., Kang, S. E., & Kay, S. A. (2012). Arabidopsis circadian clock protein, TOC1, is a DNA-binding transcription factor. Proceedings of the National Academy of Sciences of the USA, 109(8), 3167–3172. https://doi.org/10.1073/pnas.1200355109
- Goda, T., Teramura, H., Suehiro, M., Kanamaru, K., Kawaguchi, H., Ogino, C., Kondo, A., & Yamasaki, M. (2016). Natural variation in the glucose content of dilute sulfuric acid-pretreated rice straw liquid hydrolysates: Implications for bioethanol production. Bioscience, Biotechnology, & Biochemistry, 80(5), 863–869. https://doi.org/10.1080/09168451.2015.1136882
- Hirano, T., Saito, Y., Ushimaru, H., & Michiyama, H. (2005). The effect of the amount of nitrogen fertilizer on starch metabolism in leaf sheath of japonica and indica rice varieties during the heading period. Plant Production Science, 8(2), 122–130. https://doi.org/10.1626/pps.8.122
- Liu, Y. G., Mitsukawa, N., Oosumi, T., & Whittier, R. F. (1995). Efficient isolation and mapping of arabidopsis thaliana T-DNA insert junctions by thermal asymmetric interlaced PCR. The Plant Journal: For Cell and Molecular Biology, 8(3), 457–463. https://doi.org/10.1046/j.1365-313X.1995.08030457.x
- Li, Y., & Xu, M. (2017). CCT family genes in cereal crops: A current overview. The Crop Journal, 5(6), 449–458. https://doi.org/10.1016/j.cj.2017.07.001
- Miyagawa, F., Shibatani, N., Morita, R., Sasayama, D., Hatanaka, T., Azuma, T., & Fukayama, H. (2023). Effects of knockout of CO2-responsive CCT protein, a regulator of starch synthesis on physiological characteristics of rice. Plant Production Science, 26(3), 273–286. https://doi.org/10.1080/1343943X.2023.2233161
- Morita, R., Sugino, M., Hatanaka, T., Misoo, S., & Fukayama, H. (2015). CO2-responsive CONSTANS, CONSTANS-like, and time of chlorophyll a/b binding protein Expression1 protein is a positive regulator of starch synthesis in vegetative organs of rice. Plant Physiology, 167(4), 1321–1331. https://doi.org/10.1104/pp.15.00021
- Nemoto, Y., Nonoue, Y., Yano, M., & Izawa, T. (2016). Hd. 1, a CONSTANS ortholog in rice, functions as an Ehd1 repressor through interaction with monocot-specific CCT-domain protein Ghd7. The Plant Journal: For Cell and Molecular Biology, 86(3), 221–233. https://doi.org/10.1111/tpj.13168
- Okamura, M., Hirose, T., Hashida, Y., Yamagishi, T., Ohsugi, R., & Aoki, N. (2013). Starch reduction in rice stems due to a lack of OsAGPL1 or OsAPL3 decreases grain yield under low irradiance during ripening and modifies plant architecture. Functional Plant Biology, 40(11), 1137–1146. https://doi.org/10.1071/FP13105
- Sugimura, Y., Michiyama, H., & Hirano, T. (2015). Involvement of α-amylase genes in starch degradation in rice leaf sheaths at the post-heading stage. Plant Production Science, 18(3), 277–283. https://doi.org/10.1626/pps.18.277
- Valverde, F. (2011). CONSTANS and the evolutionary origin of photoperiodic timing of flowering. Journal of Experimental Botany, 62(8), 2453–2463. https://doi.org/10.1093/jxb/erq449