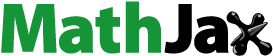
ABSTRACT
Maize-legume intercropping is a sustainable strategy to improve maize production for smallholder farmers. Maize is a staple food crop in Bhutan and integration of legumes with maize has been considered as an alternative option towards enhancing maize production in the country. Here, we conducted two studies in eastern Bhutan: first study (2021) to identify suitable legumes for intercropping with maize and second study (2022) to evaluate the effects of different maize-soybean intercropping patterns on grain yield, land use efficiency, and economic benefits. The maize-soybean intercropping system showed better productivity and economic viability than the kidney bean and groundnut systems in both the sites. Row intercropping and strip intercropping with 2 rows of maize alternating with 2 rows of soybean (2 M:2S) spaced 0.3 × 0.3 m showed stable and consistently higher grain yield at Lingmethang and Wengkhar (LING = 6.05 t ha−1; WENG = 8.37 t ha−1). The land equivalent ratio (LING = 1.78; WENG = 1.73) and net return (0.16 million Nu. ha−1 for LING and 0.21 million Nu. ha−1 for WENG) were notably higher for these intercropping methods. Our results suggest that the integration of legumes between conventional maize planting density, row intercropping and strip intercropping (2 M:2S) spaced at 0.3 × 0.3 m would be beneficial for achieving higher productivity for smallholder farmers in eastern Bhutan.
1. Introduction
Bhutan, the least-developed South Asian country, relies 69% of population on subsistence agriculture (Katwal et al., Citation2020). Maize, a staple food crop, is grown by 64% of rural households (Minisity of Agriculture and Forest, Citation2019), particularly in six eastern districts (NSB, Citation2021). Maize ensures food security for smallholder farmers, accounting for 44% of the national food composition (Wangmo, Citation2019), with per capita consumption of 109.6 g d−1 (Department of Agriculture, Citation2021b). About 80% of the total production is consumed by households, while the rest is used for seeds, processed food, and livestock feed (Katwal et al., Citation2015). The demand for maize is increasing due to rising population and the establishment of feed and distilleries (DAMC, Citation2019).
Maize yield in Bhutan has declined, with an average productivity of 3.7 t ha−1 (FAOSTAT, Citation2021), which is 37% lower than South Asian countries. The maize self-sufficiency rate also dropped from 99.2% in 2006 to 72.3% in 2019 (Department of Agriculture, Citation2021b). This decline can be attributed to various factors, including fungal diseases (Katwal et al., Citation2013), declining soil fertility, dry spell (Chhetri, Citation2019), weed infestation (Katwal et al., Citation2006), a lack of assured irrigation (Katwal, Citation2013), crop damage (MoAF, Citation2019), and socioeconomic factors (Chhogyel & Kumar, Citation2018). Commercialization and mechanization are challenging due to small farm sizes and geographical terrains (Katwal, Citation2013). Although efforts have been made to increase productivity in line with promoting organic farming (Neuhoff et al., Citation2014), synthetic fertilizers remain widely used, with 37% of fertilizers being commonly used (ICIMOD, Citation2018), and 13% specifically applied to maize fields (Dorji, Citation2008).
This emphasizes the importance of implementing sustainable agricultural practices to enhance maize productivity, especially in areas with yield decline, limited farm size, and reliance on synthetic inputs. Intercropping, which involves growing multiple crops simultaneously (Li et al., Citation2013; Maitra et al., Citation2019; Maitra & Ray, Citation2019), has been shown to offers 2–63% yield benefits over monocropping (Muoneke et al., Citation2007; Seran & Brintha, Citation2010) and contributes to 15–20% of the world’s food supply (Chai et al., Citation2021; Lithourgidis et al., Citation2011). This practice brings higher profits, risk reduction, conserved soil moisture, control pests and diseases, and balanced nutrition (Maitra, Citation2020; Maitra et al., Citation2020; Matusso et al., Citation2014). Smallholder farmers widely adopt intercropping, particularly maize-legumes systems (Foyer et al., Citation2016; Gaba et al., Citation2015; Rao et al., Citation1987), with optimal practices varying based on region, crop species, climatic conditions, terrain (Gao et al., Citation2010; Kariaga, Citation2004; Seran & Brintha, Citation2009), and spatial arrangement (Lithourgidis et al., Citation2006; Undie et al., Citation2012; Yang et al., Citation2015). For instances, maize-soybean relay strip intercropping is widely practiced in southwestern China (Yan et al., Citation2010), maize-groundnut strip intercropping in India (Choudhary & Choudhury, Citation2018), paired rows of soybean and two rows of maize in Nepal (Paudel et al., Citation2015), and paired rows of maize between four rows of groundnuts in Bangladesh (Kadir et al., Citation2021). These practices cater to the needs of marginal farmers and contribute to a more sustainable agricultural system.
Intercropping is an age-old agricultural practice in Bhutan. Many smallholder farmers still practice various types of multiple cropping such as intercropping, crop rotation, mixed cropping or sequential cropping (Katwal, Citation2013). The practices of multiple cropping are dominated by traditional technologies and practiced by farmers based on their experience. A very little research focuses on this discipline, and modern technologies for mixed cropping are limited to a few major staple crops (Katwal, Citation2013). Further, the studies by Katwal (Citation2013) documented only the practices of multiple cropping that are available and practiced by farmers in the country. Moreover, diversifying legumes through intercropping or crop rotation is considered an alternative strategy under organic farming (NOP, Citation2007), yet the proportion of legumes in mixed systems remains low, accounting for less than 52% of arable land (Katwal, Citation2013). Therefore, there are no empirical studies on multiple cropping in Bhutan, especially on the efficiency of intercropping to exploit the potential and opportunities of these practices, which is why this study was conceptualized.
The study examined the compatibility of three legume species (soybean, kidney bean, and groundnut) in two different agroecological zones, representing typical maize-growing areas in the subtropical and warm temperate zones in Bhutan. It aimed to identify suitable legumes for intercropping with maize and determine profitable intercropping patterns (mixed, row, and relay strip) relevant to the Bhutanese cropping system. The findings highlight opportunities to enhance maize production, promote sustainable agriculture practices, contribute to a more resilient farming system, and improve smallholder farmers livelihoods.
2. Materials and methods
2.1. Site description and climatic conditions
A two-year field experiment was conducted at two research centers, Agriculture Research and Development Subcenter – Lingmethang (hereinafter called ‘LING’) (27.25° N, 91.17° E); and Agriculture Research and Development Center – Wengkhar (hereinafter called ‘WENG’) (27.27° N, 91.27° E) during summer season (March–September) of 2021 (Experiment 1) and 2022 (Experiment 2) as indicated in . LING, situated at a lower altitude of 640 masl, is characterized by warm and dry climate with an average monthly temperature of 22.0°C and 492 mm rainfall. WENG, located at a higher altitude of 1780 masl, has a cool temperate climate with an average monthly temperature of 19.6°C and 516 mm rainfall, as shown in . The maize and soybean sowing season in WENG starts from March to April, while in LING, it is from April to May. The soil at both sites was silty clay, with a pH ranging from 6.56 to 6.93. The soil at WENG is generally deep and well to imperfectly drained. In contrast, the soils in LING are mostly shallow, limited by boulders, stones and gravels (NSSC, Citation2003). According to Agriculture Research and Development Center (ARDC), Wengkhar (Citation2021), the organic carbon content in WENG was 0.92%, while in LING it was 0.76%. Other soil chemical properties are as follows: N (LING: 0.07%; WENG: 0.09%), available P (LING: 64.51 mg kg−1; WENG: 3.83 mg kg−1) and available K (LING: 32.28 mg kg−1; WENG:163.59 mg kg−1) at both sites. Overall fertility potential and inherent fertility can be classified as slightly poor in both the sites (NSSC, Citation2003).
2.2. Agronomic practices and crop management
The fields were assigned different intercropping treatments using a randomized complete block design (RCBD) with three replications () . Each replicate plot measured 12 m−2 area (4 m × 3 m). The maize variety ‘Yangtsipa’ was used, an open – pollinated improved variety that matures in 110–130 days. Locally grown legume varieties such as soybean (Khangma libi), groundnut (Chalipa badam), and kidney bean (Oray tshalu), were used for intercropping. All legume varieties had a determinate growth habit that took over 120 days to mature.
Figure 3. Planting design of maize-legume intercropping system in experiment I (a) and maize-soybean intercropping patterns in experiment II (b). SM; sole maize, SS; sole soybean, SK; sole kidney bean, SG; sole groundnut, M+S; maize-soybean, M+K; maize-kidney bean, M+G; maize-groundnut, strip intercrop 2 M:2S (0.6:0.3 m); two rows of maize alternating with two rows of soybean with 0.60 m row spacing and 0.30 m plant spacing, strip intercrop 2 M:2S (0.3:0.3 m); two rows of maize alternating with two rows of soybean with 0.30 m row spacing and 0.30 m plant spacing.

Three weeks prior to sowing, the soil was tilled twice, and farmyard manure (FYM) was applied at 5–7.5 t ha−1 1 week before sowing. Maize and legumes were sown simultaneously according to the row arrangement of each intercropping pattern, with reseeding at 15 days after sowing (DAS) to maintain the plant population. Maize plots were treated with chemical fertilizers as per the package of practices at the rate of 126:63:45 N:P2O5:K2O kg ha−1, in which nitrogen fertilizer being applied in three split doses: 50% at basal fertilization, 25% as top-dressing at the knee-height stage, and 25% at tasseling stage. The top-dressing of nitrogen at the knee-height and tasseling stages were only applied to the sole maize and the rows of maize in intercropping plots. Basal application of phosphorous and potassium were applied only to sole maize. As per the seed production manual for legumes (Department of Agriculture, Citation2021a), the fertilizers rate for soyabean is 20:80:40 NPK kg ha−1, however in this study no fertilizers were applied to sole soybean and intercropped soybean rows. Weeding was performed twice, at 30 and 50 DAS, however no pesticide application was carried out considering the farmers practices. Both maize and legumes were harvested at maturity.
2.3. Experiment I: identifying suitable legume species to intercrop with maize (in 2021)
Seven maize-legume intercropping systems were evaluated, including sole maize, sole soybean, sole kidney bean, sole groundnut, maize + soybean intercropping, maize + kidney bean intercropping, and maize + groundnut intercropping, using a Randomized Complete Block Design (RCBD) with three replicates. Both maize and legumes were sown simultaneously on March 30 and 9 April 2021, in WENG and LING, respectively, with a 0.7 m spacing between rows and 0.35 m between plants. In this study, sole maize (SM) was used as the farmers’ practice, and an additive intercropping design was made considering the farmers’ practices. Subsequently, a similar row configuration was used, wherein one row of maize is alternated with one row of respective legumes, i.e. soybean, kidney bean, and groundnut (hereinafter abbreviated as 1 M:1 L) for all intercropping systems (). The plant densities of maize and legumes were 4.08 plants m−2 in both sole crops and intercrops.
2.4. Experiment II: determining appropriate maize-soybean intercropping pattern (in 2022)
Considering limited land owned by the farmers, in the subsequent field experiment, we used different planting density to optimize the plant populations. Seven treatments including sole maize (T1) and sole soybean (T2), several planting patterns, such as mixed intercropping (T3), row intercropping (T4), relay intercropping (T5), strip intercropping 2 M:2S (0.6:0.3 m spacing) (T6), and strip intercropping 2 M:2S (0.3:0.3 m spacing) (T7), were evaluated under a RCB design with three replicates (). Although intercropping patterns differed in the second study, row-to-plant spacing, was similar for T1 to T6 (0.6-m row to 0.3-m plants), except for T7 where row-to-plant spacing was 0.3-m. Both maize and soybean were sown simultaneously irrespective of row arrangement on March 30 and 12 May 2022, in WENG and LING, respectively; however, soybeans in the relay intercropping plots were sown 30 days after maize planting when maize plants reached the knee-height stages. Strip intercropping had two rows of maize alternating with two rows of soybean (hereinafter abbreviated as 2 M:2S), with 0.60-m row spacing in T6 and 0.30-m in T7 (). The density of maize and soybean in T1, T2, T4, T5, and T7 was 5.6 plants m−2, similar to conventional planting density, whereas it was 2.8 plants m−2 in T3 and T6, which was 50% lower than that of sole maize and intercrop patterns.
2.5. Measurements
The maize was harvested at its physiological maturity to determine the grain yield. All cobs from the harvested area were weighed to determine the plot yield (kg). Twenty cobs from each plot were shelled, and the fresh grain weight (FGW) was recorded. The shelling recovery percentage (SR%) was calculated by dividing the FGW (kg) by weight (kg) of the 20 cobs.
The grain yield of legumes (soybean, kidney bean, and groundnut) was measured at their physiological maturity. All pods of soybean, kidney bean and groundnut from the harvested area were shelled to obtain the FGW (kg). Grain moisture content of both maize and legumes was determined using a universal grain moisture meter (INDOSAN S6010) and adjusted to standardized moisture content as shown in formula 1. The grain yield was then expressed in t ha−1 at 15% moisture content, as indicated in formula 2.5.2.
Intercropping advantages were evaluated by calculating the partial land equivalent ratio (PLER) and land equivalent ratio (LER). Those are an indicator that assess the resource use efficiency and productivity of intercropping over sole cropping (Bilalis et al., Citation2005). PLER and LER were calculated as Yang et al. (Citation2018) and Mead and Willey (Citation1980).
where Yintercrop is the grain yield of maize and legumes (soybean, kidney bean and groundnut) in intercropping, while Ysolecrop is the grain yield of maize and legumes in sole cropping.
The land equivalent ratio (LER) was calculated as the sum of the relative yield of maize and legumes in the intercropping plots to the sole cropping (Mead & Willey, Citation1980).
where Yil is the grain yield of legumes in intercropping and Yim is the grain yield of maize in intercropping. While, Ysl indicates the grain yield of legumes in sole cropping, Ysm is the grain yield of maize in sole cropping.
A LER values greater than 1.0 suggest intercropping is more productive and efficient over sole cropping, and vice versa (Kermah et al., Citation2017; Li et al., Citation2011).
Land Equivalent coefficient (LEC) used to assess the interaction and production potential of crop mixture (Adetiloye et al., Citation1983).
where Yaa and Ybb: pure crop yield of maize and intercrops, respectively, Yab and Yba: yield of main crop and intercrops in mixture. In the land equivalent coefficient is more than 25% (0.25), it suggests there is yield advantage over other systems (Ejigu Ejara & Tolessa Taye, Citation2017).
System productivity index (SPI) were used to identify the intercrop have higher productivity and stability (Odo, Citation1991).
where, SA and LB: yield of maize and intercrops in sole cropping, Sa and Lb: yield of maize and intercrops in intercropping.
The economic benefits of intercropping systems were determined by calculated gross margin and economic output. Gross margin was calculated by determining gross revenue (GR) and total variable costs incurred during the growing season. Variable costs (Bhutanese currency Nyultrum, Nu), included labor, inputs, manure application, and machinery charges (Nu. 1500 day−1), weeding, irrigation, pesticide application, harvesting, and post-harvest handling. Labor costs are based on the national wage rate (Nu. 265 day−1). Total grain yield was categorized as the total output, and gross revenue was estimated by multiplying the total yield (kg ha−1) by market prices (maize = Nu. 25 kg−1, soybean = Nu. 90 kg−1, kidney bean = Nu. 80 kg−1, groundnut =Nu.100 kg−1). Net return (Nu ha−1) was determined by subtracting the total input cost from gross revenue.
The benefit – cost ratio (BCR) was used to assess the relationship between costs and benefits (Hayes et al., Citation2022), with a BCR greater than 1.0, indicating a positive net benefit for smallholder farmers and vice versa (Hayes et al., Citation2022). BCR was obtained by dividing the net return by the total input cost of each intercropping system, as adopted from previous study of Yang et al. (Citation2018) as follows:
2.6. Statistical analysis
A two-way ANOVA was performed to test the effects of intercropping systems and the interaction of these factors with location. The treatments were compared using the least significant difference (LSD) test at p < 0.05. The Statistical Tool for Agricultural Research (STAR), Statistical Package for Social Sciences (SPSS) v25, and Microsoft Excel 2019 were used for statistical analyses.
3. Results
3.1. Experiment I: identifying suitable legume species to intercrop with maize (in 2021)
3.1.1. Grain yield of maize and legumes
Intercropping systems did not significantly influence (p > 0.05) maize grain yield at any site; however, the intercropping plots showed a slightly higher maize grain yield than sole cropping (). Maize – soybean intercropping tended to exhibit better maize grain yield at the LING and WENG sites, with 6.50 t ha−1 and 6.86 t ha−1, respectively ().
Figure 4. Grain yield of maize among the different maize-legume intercropping systems. Within each location, values followed by different lower-case alphabetical letter represent significant differences between treatments at 5% significance level. Error bars indicate the standard error of three replicates. LING; LIngmethang, WENG; Wengkhar, SM; sole maize, M+S; maize-soybean, M+K; maize-kidney bean and M+G; maize-groundnut.

Meanwhile, the grain yield of intercropped legumes was significantly reduced at both sites as compared to sole cropping, but no interaction between location and system was observed (). Sole soybean had a considerably higher grain yield, with 1.17 t ha−1 at LING and 1.24 t ha−1 at WENG sites (). No differences in yield reduction were observed among legume species, with groundnut intercropping showing a 34% yield reduction, kidney bean a 31% reduction, and soybean a 25% reduction ().
Figure 5. Grain yield of legumes (soybean, kidney bean and groundnut) among different maize – legume intercropping systems. Within each location, values followed by different lower-case alphabetical letter represent significant differences between treatments at 5% significance level. Error bars indicate the standard error of three replicates. LING; Lingmethang, WENG; Wengkhar, SS; sole soybean, SK; sole kidney bean, SG; sole groundnut, M+S; maize-soybean, M+K; maize-kidney bean and M+G; maize-groundnut.

3.1.2. Intercropping efficiency
There were no significant effects of intercropping system on PLER maize, PLER legumes and LER values exhibiting no interaction between location and intercropping systems, but all system showed LER values greater than one, indicating better yield advantages over sole cropping (). Similarly, SPI did not vary among the systems, however productivity differed significantly by location (). Among all, maize-soybean had better PLER maize (LING = 1.18; WENG = 1.07) and PLER legumes (LING = 0,67; WENG = 0.82), LER values (LING = 1.85; WENG = 1.89), LEC (LING = 0.78; WENG = 0.88) and SPI (LING = 10.23; WENG = 12.14) at both sites as shown in .
Table 1. Efficiency of different maize – legume intercropping systems.
3.1.3. Economic advantages
Economic characteristics, such as total cost, output, net return, and benefit–cost ratio, were significantly influenced by intercropping systems, but no interaction between location and system was observed (). Maize intercropped with groundnuts had higher production costs (LING = Nu. 0.093 million and WENG = Nu. 0.100 million) (). Maize-soybean intercropping showed substantially greater output (LING = Nu. 0.233 million; WENG = Nu. 0.240 million) and net return ability (LING = Nu. 0.143 million; WENG = Nu. 0.147 million) (). The benefit-cost ratio (BCR) in the intercropping plots (M+S, M+K, and M+G) was significantly higher than sole cropping (SM, SS, SK, and SG), with BCR ranging from 1.17 to 1.56 at LING and 1.18–1.57 at WENG condition as shown in . All intercrops were observed to be economically profitable, with the maize-soybean intercropping system (LING = 1.56; WENG = 1.57) being the most profitable system compared to the other two legume intercrops ().
Table 2. Economic benefits of different maize – legume intercropping systems all expressed in millions of nu (nu = ngultrum, Bhutanese).
3.2. Experiment II: determining appropriate maize-soybean intercropping pattern (in 2022)
3.2.1. Grain yield of maize and soybean
There were significant differences in maize grain yield among intercropping patterns, with an interaction between location and intercropping patterns (). In a cool climate (WENG), strip intercropping with 2 M:2S at a 0.3 m interval (8.37 t ha−1) and row intercropping (7.45 t ha−1) showed significantly higher maize grain yield. In a warm and dry climate (LING), relay intercropping (6.89 t ha−1) and strip intercropping with 2 M:2S at a 0.3 m interval (6.05 t ha−1) displayed considerably higher maize yields. Despite variability in its performance, strip intercropping with 2 M:2S at 0.3 m interval had a stable, consistently higher yield at both sites (). Mixed intercropping and strip intercropping with 2 M:2S at a 0.6 m interval had significantly lower grain yields compared to sole maize, decreasing by 67% and 61%, respectively.
Figure 6. Grain yield of maize under different maize-soybean intercropping patterns. Within each location, values followed by different lower-case alphabetical letter represent significant differences between treatments at 5% significance level. Error bars indicate the standard error of three replicates. LING; Lingmethang, WENG; Wengkhar, SM; sole maize, MI; mixed intercropping, RI; row intercropping, relay I; relay intercropping, SI 2 M:2S (0.6:0.3 m): strip intercropping with two rows of maize alternating with two rows of soybean at 0.60-m row spacing and 0.30-m plant spacing and SI 2 M:2S (0.3:0.3 m): strip intercropping with two rows of maize alternating with two rows of soybean at 0.30-m row spacing and 0.30-m plant spacing.

Similarly, grain yield of intercropped soybean was significantly reduced among the intercropping patterns, especially in relay intercropping, by 67% and 66% at LING and WENG, respectively, as compared to sole cropping (). As compared to other intercropping patterns, sole soybean showed consistently significantly higher yields in both locations ().
Figure 7. Grain yield of soybean under different maize-soybean intercropping patterns. Within each location, values followed by different lower-case alphabetical letter represent significant differences between treatments at 5% significance level. Error bars indicate the standard error of three replicates. LING; Lingmethang, WENG; wengkhar, SS: sole soybean, MI; mixed intercropping, RI; row intercropping, relay I; relay intercropping, SI 2 M:2S (0.6:0.3 m): strip intercropping with two rows of maize alternating with two rows of soybean at 0.60-m row spacing and 0.30-m plant spacing and SI 2 M:2S (0.3:0.3 m): strip intercropping with two rows of maize alternating with two rows of soybean at 0.30-m row spacing and 0.30-m plant spacing.

3.2.2. Intercropping efficiency
Intercropping patterns significantly affect LER values, with all intercropping patterns showing yield advantages over sole cropping (). Row (1.77) and strip intercropping with 2 M:2S at a 0.3-m interval (1.73) showed higher yield advantages of 44% and 42%, respectively, compared to sole maize under WENG conditions. Relay intercropping (1.53) and strip intercropping with 2 M:2S at a 0.3-m interval displayed 35% and 44%, respectively, yield advantages under LING conditions. However, strip intercropping with 2 M:2S at a 0.6-m interval and mixed intercropping had the lowest LER values, suggesting lower productivity and yield benefits ().
Table 3. Intercropping efficiency under different maize-soybean intercropping patterns.
Significant interaction between location and intercropping patterns was observed in LEC (LING; 0.76; WENG = 0.60) and SPI (LING = 10.17; WENG = 11.64), with strip intercropping with 2 M:2S at 0.3-m interval exhibiting higher system productivity at both sites ().
3.2.3. Economic advantages
Economic characteristics varied among intercropping patterns, with strip intercropping with 2 M:2S at a 0.3-m interval showing optimal output (LING = Nu. 0.262 million; WENG = Nu. 0.311 million) despite the highest production cost (LING = 0.103 million; WENG = Nu. 0.105 million) at either site (). The net return ability varied site-specifically, with strip intercropping with 2 M:2S at a 0.3-m interval (Nu. 0.205 million) and row intercropping (Nu. 0.204 million) producing higher net returns in cooler climatic conditions. Relay intercropping (Nu. 0.146 million) and strip intercropping with 2 M:2S at a 0.3-m interval (Nu. 0.159 million) showed higher net returns in warm and dry climatic conditions. The benefit–cost ratio for sole soybean, mixed intercropping, and strip intercropping with 2 M:2S at a 0.6-m interval is exhibited below one, indicating inefficiency and unprofitability. Row intercropping (LING = 1.20; WENG = 2.02) and strip intercropping with 2 M:2S at a 0.3-m interval (LING = 1.54; WENG = 1.95) showed stable and consistently higher BCR values, suggesting economically profitable intercropping patterns ().
Table 4. Economic benefits of different maize-soybean intercropping patterns all expressed in millions of nu (nu = ngultrum, Bhutanese).
4. Discussions
In this study, field experiments were conducted to develop an intercropping system with maize and legumes in eastern Bhutan, focusing on the optimal legume crop and the ideal planting pattern. Although both field experiments were conducted in a single year, they were conducted in two different environments, Lingmethang (640 masl) and Wengkhar (1780 masl), and some of the results were common to both environments. Therefore, these findings are important for the future development of maize-based mixed-cropping systems in Bhutan.
Although fertility potential and inherent fertility at both sites were classified as slightly poor, we believe that the grain yield of maize was not affected because we applied chemical fertilizer to maize based on its package of practice. Moreover, legumes are symbiotic (Blessing et al., Citation2022) and can improve soil fertility by fixing atmospheric nitrogen at approximately 150 tons year−1 globally (Ananthi et al., Citation2017; Maitra et al., Citation2021; Martin-Guay et al., Citation2018). Maize-legume intercropping efficiently utilizes available resources (Yu et al., Citation2016) and has shown yield advantages ranging from 2 to 63% over monocultures (Muoneke et al., Citation2007; Seran & Brintha, Citation2010). Thus, inclusion of legumes in conventional maize planting could enhance soil fertility, which might subsequently reduce the use of synthetic fertilizers that some smallholder farmers cannot afford to purchase. The results of this studies were mainly the result of light competition, however further studies are needed to determine the optimal fertilizers management in maize-soybean intercropping systems.
The maize-legume intercropping system showed no differences in maize grain yield and land equivalent ratio ( and ), attributed to similar row arrangements (1 M: 1 L), plant density, and agronomic practices. These results align with previous studies by Kheroar and Patra (Citation2013), who observed no discernible effect on maize grain yield when intercropped with green gram, black gram, soybean, and peanut under a similar row arrangement (1:1). Although there was no significant difference in maize grain yield among the intercropping systems, the maize grain yield in the intercropped plots was slightly greater than that in the pure stands, which is consistent with the findings of Z. G. Wang et al. (Citation2014), Ren et al. (Citation2017), and Sikirou and Wydra (Citation2008). Better grain yield could be due to the inclusion of legumes (such as soybeans, kidney beans, and peanuts), which might have resulted in the complementarity of growth resources (Maitra & Ray, Citation2019), particularly soil N in which legumes in intercrops fixed 15–97 kg ha−1 of N2 representing 67–71% of N2 fixed by respective sole crop legumes (Kermah et al., Citation2017) However, the maize-soybean intercropping system was tended to be more productive and profitable than the kidney bean and groundnut systems ( and ). This can be explained by the high price of soybeans in the Bhutanese market. A kilogram of soybean costs Nu.90 in Bhutan, which is comparatively higher than other legumes. Moreover, soybean yield was relatively better than that of other legumes. Despite the insignificant maize yield among intercropping systems in our study, we believe that there is room for soybean crop to utilize the light that maize cannot absorb and that the nutrient accumulation ability might be better than that of other legumes. This was consistent with the results of Padhi and Panigrahi (Citation2006), where maize-soybean intercropping displayed the highest soil available N and P compared to blackgram and groundnut intercropping, resulting in better maize yield. Similarly, Singh et al. (Citation2008) also confirmed that maize-soybean intercropping showed the highest total available soil N, P, and K content, complementing better yield compared to cowpea, green gram, and french bean intercrops regardless of the row arrangement. This may have made maize-soybean intercropping more profitable for smallholder farmers in Bhutan than the other two legumes intercropping systems.
Subsequent field experiment in 2022, maize grain yield (), LER, LEC, SPI () and economic characteristics () varied significantly among different maize-soybean intercropping patterns, which could be ascribed to the varying row arrangements of maize and soybean plants. This is consistent with the findings of Zhang et al. (Citation2011) that different planting arrangements affect productivity and intercropping efficiency. Variations in planting arrangements also influence how plants perceive and utilize growth resources to enhance grain yields, as reported by Wei (Citation2016).
As the results indicated, the efficiencies of intercropping patterns were highly variable and site-specific, where row intercropping and strip intercropping with 2 M:2S at a 0.3-m interval showed considerably higher grain yield under WENG with cool climatic conditions. However, relay and strip intercropping with 2 M:2S at a 0.3-m interval had greater performance under LING with warm and dry climatic conditions. The variation in the efficiencies of intercropping patterns at each location could be attributed to interaction of different intercropping pattern with growing microclimatic conditions and varying row arrangement, that favored certain intercropping patterns and limited the others that exhibits difference in its performances at each location. Though relay intercropping performed better at low altitudes, but maize grain yield was not very low at high altitudes, showing a similar trend in its productivity.
Although optimal intercropping patterns differ with location, the row intercropping and strip intercropping with 2 M:2S at a 0.3-m interval showed stable and consistent grain yield, LER, and economic characteristics, indicating a profitable intercropping pattern in both the study sites. This stability can be attributed to higher planting density of maize and better grain yield of soybeans. The planting density of maize in these plots was 5.6 plants m−2, similar to the conventional planting density practiced by farmers. Incorporating soybean in between the rows of conventional planting density could be beneficial that enhances grain yield and land use efficiencies for smallholder farmers. These results align with previous studies Stacciarini et al. (Citation2010) and Yang et al. (Citation2015) showing higher planting densities of maize result in higher grain yields. Therefore, the study sites represent the major maize growing area in Bhutan, thus the results of this study can be generalized as a profitable intercropping method that can be recommended in the country.
On the other hand, grain yield of intercropped legumes is significantly reduced, exclusively attributed to the shading effect of maize plants. Several studies have also reported a reduction in legume yield in intercropped plots due to shading effects (Kebebew, Citation2014; Mudita et al., Citation2008; Undie et al., Citation2012). In addition, different planting patterns modify the plant population in intercropping plots, resulting in a 20–50% lower yield than sole cropping (Kheroar & Patra, Citation2014; Manasa et al., Citation2018). Among all, grain yield of soybean in relay intercropping was significantly reduced, possibly ascribed to the timing of planting soybean, which planted during knee-height stages of maize (approximately 30 DAS) might have shaded the understory soybean and affected the grain yield, suggesting timing of soybean planting or soybean varieties with shade tolerances is critical for relay intercropping practices. Despite a significant reduction in legume yield, the productivity of the entire system was improved, or rather, the maize yield was increased.
The study observed that mixed intercropping and strip intercropping with 2 M:2S at a 0.6-m interval had 53% and 52% lower efficiencies compared to strip intercropping with 2 M:2S at a 0.3-m interval under WENG and LING, respectively, indicating these patterns have no yield advantages over sole cropping. This inefficiency is ascribed to low planting density per unit area (2.8 plants m−2), which is 50% lower than sole maize and other patterns, consistent with the results of Abdel-Aziz et al. (Citation2012) and M. Yang et al. (Citation2014). The low yield of soybean in mixed cropping systems is likely due to shading by taller maize plants, which may have reduced the photosynthetic rate of soybean in the understory (Bitew et al., Citation2021; Dhar et al., Citation2013; Harsono et al., Citation2020; Mandal et al., Citation2014; Mugi-Ngenga et al., Citation2022). Other studies have also reported that shading by maize significantly reduces the yield of intercropped soybean (Kebebew, Citation2014; Mudita et al., Citation2008; Undie et al., Citation2012). Furthermore, the efficient use of solar radiation and the quality of light intercepted by the canopy are important determinants of yield components in intercropping (Liu et al., Citation2010). Considering those, the maize and soybean plants under strip intercropping 2 M:2S (row interval at 0.3-m and 0.6-m) might have received more solar radiation than those maize and soybean plants with row interval at 0.6-m in RI and Relay I. This could have been attributed to the wider space in the upper canopy, which enabled higher RUE, resulting in better yield in strip intercropping, as reported by Maitra and Ray (Citation2019) and Z. Wang et al. (Citation2015). In this regard, the grain yield of maize and soybean observed in our study was somewhat consistent, in which strip intercropping with two rows of maize alternated with two rows of soybean (2 M:2S) exhibited considerably higher grain yield. Our results are consistent with the findings of Pandey and Bhambri (Citation2017) that the paired row of maize-soybean intercropping exhibited the highest light use efficiency (LUE), resulting in a higher grain yield. In addition, Cui et al. (Citation2014) and Yang et al. (Citation2017) reported that maize-soybean strip intercropping provides understory soybean with higher light penetration of up to 50–60% without compromising the desired light quality. Furthermore, Liu et al. (Citation2017) observed that the photosynthetically active radiation (PAR) intercepted by maize – soybean strip intercropping was 1.35 times higher than that in row intercropping (Liu et al., Citation2017). Anyhow, the results of mixed intercropping contradict farmers current practices, that assumed mixed cropping is profitable, suggesting that planting density could be the key factor influencing grain yield under existing mixed intercropping practices. Modifying or adjusting crop rates in the mixture could optimize its productivity relative to other practices.
In addition to increasing crop productivity per unit area, providing better economic returns to smallholder farmers is the main purpose of intercropping. The study found that all intercropping systems were economically profitable, but the performance of each economic characteristic varied significantly among the patterns (), attributed to different row configurations necessitating distinct intercultural practices during the growing season. Row intercrop and strip intercropping with 2 M:2S at a 0.3-m interval had considerably higher economic benefits, especially net return abilities and benefit-cost ratios, despite higher production costs (). Higher grain yields and better market prices for soybeans were associated with increased economic benefits. These intercropping patterns are profitable in Bhutanese maize-based cropping systems, but they require higher labor requirements, raising efficiency concerns suggesting further studies to reduce the labor requirements are needed to optimize their efficiencies.
5. Conclusion
Two experiments conducted in eastern Bhutan found no significant differences in intercropping systems for legumes and maize. The maize-soybean intercropping tended to be more productive and profitable than kidney beans and groundnuts systems. Subsequent experiments in 2022 showed that maize-soybean intercropping productivity was highly variable and site-specific. Among them, row and strip intercrop with 2 M:2S at a 0.3-m interval showed stable and consistently higher grain yield (LING = 6.05 t ha−1; WENG = 8.37 t ha−1), LER (LING = 1.78; WENG = 1.73), and economic return (LING = 0.16 million; WENG = 0.21 million), indicating that these configurations are the most profitable pattern for existing maize cropping systems in eastern Bhutan. In addition to higher productivity, higher labor inputs were also required, which could be a challenge for farmers with limited labor. Therefore, strategies for labor-saving techniques must be explored by optimizing row configurations so that agronomic practices can be mechanized wherever possible. This study highlights that integrating legumes between conventional maize planting density could be beneficial, and that strip intercropping with 2 M:2S at a 0.3-m interval needs to be promoted through an extensional program to diversify the existing cropping systems. However, an evaluation of variety selection, optimal row spacing, and planting density for strip intercropping is needed to develop a reliable approach to increase smallholder maize productivity.
Acknowledgments
We thank field attendants of both ARDC-Wengkhar and ARDSC-Lingemthang for the support of field experiments.
Disclosure statement
No potential conflict of interest was reported by the author(s).
Additional information
Funding
References
- Abdel-Aziz, M., Abou-Elela, U., Abd El Razek, A., & Khalil, H. E. (2012). Yield and its components of maize/soybean intercropping systems as affected by planting time and distribution. Australian Journal of Basic and Applied Sciences, 6(13), 238–245.
- Adetiloye, P. 0., Ezedinma, F. O. C., & Okigbo, B. N. (1983). A land equivalent coefficient (LEC) concept for the evaluation of competitive and productive interactions in simple to complex crop mixtures. Ecological Modelling, 19(1), 27–39. https://doi.org/10.1016/0304-3800(83)90068-6
- Agriculture Research and Development Center, Wengkhar (ARDC-W). (2021). Soil Survey Report. Soil Section, Bhutan, Mongar, Wengkhar.
- Ananthi, T., Amanullah, M. M., & Al-Tawaha, A. R. M. S. (2017). A review on maize-legume intercropping for enhancing the productivity and soil fertility for sustainable agriculture in India. Advances in Environmental Biology, 11(5), 49–64.
- Bilalis, D. J., Sidiras, N., Kakampouki, I., Efthimiadou, A., Papatheohari, Y., & Thomopoulos, P. (2005). Effects of organic fertilization on maize/legume intercrop in a clay loam soil and mediterranean climate-can the land equivalent ratio (LER) index be used for root development? Journal of Food Agriculture and Environmental, 3(3/4), 117.
- Bitew, Y., Derebe, B., Worku, A., Chakelie, G., & Shah, A. N. (2021). Response of maize and common bean to spatial and temporal differentiation in maize-common bean intercropping. Public Library of Science ONE, 16(10), e0257203. https://doi.org/10.1371/journal.pone.0257203
- Blessing, D. J., Gu, Y., Cao, M., Cui, Y., Wang, X., & Asante-Badu, B. (2022). Overview of the advantages and limitations of maize-soybean intercropping in sustainable agriculture and future prospects: A review. Chilean Journal of Agricultural Research, 82(1), 177–188. https://doi.org/10.4067/S0718-58392022000100177
- Chai, Q., Nemecek, T., Liang, C., Zhao, C., Yu, A., Coulter, J. A., Siddique, K. H. M., Hu, F., Wang, L., Siddique, K. H. M., & Gan, Y. (2021). Integrated farming with intercropping increases food production while reducing environmental footprint. Proceedings of the National Academy of Sciences, 118(38), e2106382118. https://doi.org/10.1073/pnas.2106382118
- Chhetri, S. (2019). Integrated plant nutrition system modules for major crops and cropping systems in Bhutan (Vol. 176, p. 78). SAARC Agriculture Centre.
- Chhogyel, N., & Kumar, L. (2018). Climate change and potential impacts on agriculture in Bhutan: A discussion of pertinent issues. Agriculture & Food Security, 7(1), 1–13. https://doi.org/10.1186/s40066-018-0229-6
- Choudhary, V. K., & Choudhury, B. U. (2018). A staggered maize–legume intercrop arrangement influences yield, weed smothering and nutrient balance in the eastern himalayan region of India. Experimental Agriculture, 54(2), 181–200. https://doi.org/10.1017/S0014479716000144
- Cui, L., Su, B. Y., Yang, F., & Yang, W. Y. (2014). Effects of photo-synthetically active radiation on photosynthetic characteristics and yield of soybean in different maize/soybean relay strip intercropping systems. Scientia Agricultura Sinica, 47(8), 1489–150. https://doi.org/10.3864/j.issn.0578-1752.2014.08.005
- Department of Agriculture. (2021a). Seed production manual for legumes. Agriculture Research and Extension Division, Department of Agriculture, Ministry of Agriculture and Forest.
- Department of Agriculture. (2021b). Self-sufficiency and dietary energy supply of food crops in Bhutan: Agriculture research and extension division, department of agriculture. Ministry of Agriculture and Forest.
- Department of Agriculture and Marketing Cooperatives. (2019). Value chain study on maize, ministry of agriculture and forest, Thimphu, Bhutan. www.agrimarket.gov.bt
- Dhar, P. C., Awal, M. A., Sultan, M. S., Rana, M. M., & Sarker, A. (2013). Interspecific competition, growth and productivity of maize and pea in intercropping mixture. Journal of Crop Science, 2(10), 136–143.
- Dorji, K. D. (2008). Agriculture and soil fertility management in Bhutan: An overview. Paper presented in the meeting of Asia-Pacific Net on Integrated Plant Nutrient Management & International Workshop on Sustainable Nutrient Management: Technology and Policy, Shijiazhuang, Hebei, China, May 27-31, 2008.
- Ejigu Ejara, N. B., & Tolessa Taye, Y. S. (2017). Determinaion of appropriate maize haricot bean arrangement in moisture stress areas of Borana, southern ethiopa. Journal of Ecobiotechnology, 9, 18–23. https://doi.org/10.25081/jebt.2017.v9.3447
- Food and Agriculture Organization. (2021). Food and agriculture data-FAO STAT. https://www.fao.org/faostat/en/#data/QCL
- Foyer, C. H., Lam, H. M., Nguyen, H. T., Siddique, K. H. M., Varshney, R. K., Colmer, T. D., Hodgson, J. M., Bramley, H., Mori, T. A., Hodgson, J. M., Cooper, J. W., Miller, A. J., Kunert, K., Vorster, J., Cullis, C., Ozga, J. A., Wahlqvist, M. L., Liang, Y. … Valliyodan, B. (2016). Neglecting legumes has compromised human health and sustainable food production. Nature Plants, 2(8), 1–10. https://doi.org/10.1038/nplants.2016.112
- Gaba, S., Lescourret, F., Boudsocq, S., Enjalbert, J., Hinsinger, P., Journet, E. P., Navas, M.-L., Wery, J., Louarn, G., Malézieux, E., Pelzer, E., Prudent, M., & Ozier-Lafontaine, H. (2015). Multiple cropping systems as drivers for providing multiple ecosystem services: From concepts to design. Agronomy for Sustainable Development, 35(2), 607–623. https://doi.org/10.1007/s13593-014-0272-z
- Gao, Y., Duan, A., Qiu, X., Sun, J., Zhang, J., Liu, H., & Wang, H. (2010). Distribution and use efficiency of photosynthetically active radiation in strip intercropping of maize and soybean. Agronomy Journal, 102(4), 1149–1157. https://doi.org/10.2134/agronj2009.0409
- Harsono, A., Elisabeth, D. A. A., Muzaiyanah, S., & RIANTO, S. A. (2020). Soybean-maize intercropping feasibility under drought-prone area in East Java, Indonesia. Biodiversitas Journal of Biological Diversity, 21(8). https://doi.org/10.13057/biodiv/d210842
- Hayes, A., Kindness, D., & Li, T. (2022). Benefit-cost ratio (BCR): Definition, formula, and example. Investopedia. https://www.investopedia.com/terms/b/bcr.asp
- International Center for Integrated Mountain Development (ICIMOD). (2018). Organic agriculture development strategies: ROadmap for 12th five year plan and beyond. ICIMOD. https://lib.icimod.org/record/33841
- Kadir, M., Rahman, J., Riad, M. I., & Alam, M. K. (2021). Intercropping maize with groundnut in Char Land. Tropical Agrobiodiversity, 2(1), 51–53. https://doi.org/10.26480/trab.01.2021.51.53
- Kariaga, B. M. (2004). Intercropping maize with cowpeas and beans for soil and water management in Western Kenya. Proceedings of the 13th International Soil Conservation Organization Conference, July 4-9, 2004 (pp. 1–5). Conserving Soil and Water for Society. htt ps://doi.org/2010.135.145
- Katwal, T. B. (2013). Multiple cropping in Bhutanese agriculture: Present status and opportunities. Paper presented at the Regional Consultative Meeting on Popularizing Multiple Cropping Innovations as a Means to Raise Productivity and Farm Income in SAARC Countries, 31st October-1st November, Peradeniya, Kandy, Srilanka.
- Katwal, T. B., Bazile, D., & Chemura, A. (2020). First adaptation of quinoa in the Bhutanese mountain agriculture systems. Public Library of Science ONE, 15(1), e0219804. https://doi.org/10.1371/journal.pone.0219804
- Katwal, T. B., Dem, P., Chhetri, G. B., Bockel, L., & Punjabi, M. (2006). Maize commodity chain analysis. Department of Agriculture, Ministry of Agriculture.
- Katwal, T. B., Wangchuk, D., Dorji, L., Wangdi, N., & Choney, R. (2013). Evaluation of gray leaf spot tolerant genotypes from CIMMYT in the highland maize production eco-systems of Bhutan. Journal of Life Sciences, 7(5), 443.
- Katwal, T. B., Wangchuk, D., Wangdi, N., Choney, R., & Dorji, L. (2015). Community based seed production—A sustainable seed production model for subsistence of Bhutanese maize farmers. The Journal of Food Science Engineering, 5(2), 76–84. https://doi.org/10.17265/2159-5828/2015.02.004
- Kebebew, S. (2014). Intercropping soybean (glycine max L. Merr.) at different population densities with maize (zea mays L.) on yield component, yield and system productivity at Mizan Teferi, Ethiopia. Journal of Agricultural Economics, Extension and Rural Development, 1(7), 121–127.
- Kermah, M., Franke, A. C., Adjei-Nsiah, S., Ahiabor, B. D. K., Abaidoo, R. C., & Giller, K. E. (2017). Maize-grain legume intercropping for enhanced resource use efficiency and crop productivity in the Guinea savanna of northern Ghana. Field Crops Research, 213, 38–50. https://doi.org/10.1016/j.fcr.2017.07.008
- Kheroar, S., & Patra, B. C. (2013). Advantages of maize-legume intercropping systems. Journal of Agricultural Science and Technology, 3(10B), 733.
- Kheroar, S., & Patra, B. C. (2014). Productivity of maize-legume intercropping systems under rainfed situation. African Journal of Agricultural Research, 9(20), 1610–1617. https://doi.org/10.5897/AJAR2013.7997
- Li, Q. Z., Sun, J. H., Wei, X. J., Christie, P., Zhang, F. S., & Li, L. (2011). Overyielding and interspecific interactions mediated by nitrogen fertilization in strip intercropping of maize with faba bean, wheat and barley. Plant and Soil, 339(1–2), 147–161. https://doi.org/10.1007/s11104-010-0561-5
- Lithourgidis, A. S., Dordas, C. A., Damalas, C. A., & Vlachostergios, D. N. (2011). Annual intercrops: An alternative pathway for sustainable agriculture. Australian Journal of Crop Science, 5(4), 396–410.
- Lithourgidis, A. S., Vasilakoglou, I. B., Dhima, K. V., Dordas, C. A., & Yiakoulaki, M. D. (2006). Forage yield and quality of common vetch mixtures with oat and triticale in two seeding ratios. Field Crops Research, 99(2–3), 106–113. https://doi.org/10.1016/j.fcr.2006.03.008
- Liu, B., Liu, X. B., Wang, C., Jin, J., Herbert, S. J., & Hashemi, M. (2010). Responses of soybean yield and yield components to light enrichment and planting density. International Journal of Plant Production, 4(1), 1–10.
- Liu, X., Rahman, T., Song, C., Su, B., Yang, F., Yong, T., Wu, Y., Zhang, C., & Yang, W. (2017). Changes in light environment, morphology, growth and yield of soybean in maize-soybean intercropping systems. Field Crops Research, 200, 38–46. https://doi.org/10.1016/j.fcr.2016.10.003
- Li, L., Zhang, L., & Zhang, F. (2013). Crop mixtures and the mechanisms of overyielding. In S. A. Levin (Ed.), Encyclopedia of Biodiversity (2nd ed, Vol. 2, pp. 382–395). Academic Press. https://doi.org/10.1016/B978-0-12-384719-5.00363-4
- Maitra, S. (2020). Intercropping of small millets for agricultural sustainability in drylands: A review. Crop Research, 55(3and4), 162–171.
- Maitra, S., Hossain, A., Brestic, M., Skalicky, M., Ondrisik, P., Gitari, H., Palai, J. B., Shankar, T., Bhadra, P., Palai, J. B., Jena, J., Bhattacharya, U., Duvvada, S. K., Lalichetti, S., & Sairam, M. (2021). Intercropping—A low input agricultural strategy for food and environmental security. Agronomy for Sustainable Development, 11(2), 343. https://doi.org/10.3390/agronomy11020343
- Maitra, S. Palai, J. B. Manasa, P. & Kumar, D. P. (2019). Potential of intercropping system in sustaining crop productivity. International Journal of Agriculture, Environment Biotechnology, 12(1), 39–45. https://doi.org/10.30954/0974-1712.03.2019.7
- Maitra, S., & Ray, D. P. (2019). Enrichment of biodiversity, influence in microbial population dynamics of soil and nutrient utilization in cereal-legume intercropping systems: A review. International Journal of Bioresource Science, 6(1), 11–19. https://doi.org/10.30954/2347-9655.01.2019.3
- Maitra, S., Shankar, T., & Banerjee, P. (2020). Potential and advantages of maize-legume intercropping system. In A. Hossain (Ed.) Maize-Production Use (pp. 1–14). IntechOpen. https://doi.org/10.5772/intechopen.91722
- Manasa, P., Maitra, S., & Reddy, M. D. (2018). Effect of summer maize-legume intercropping system on growth, productivity and competitive ability of crops. International Journal of Management, Technology, and Engineering, 8(12), 2871–2875.
- Mandal, M. K., Banerjee, M., & Banerjee, H. (2014). Productivity of maize (zea mays) based intercropping system during kharif season under red and lateritic tract of West Bengal. The Bioscan, 9(1), 117–126. https://doi.org/10.3329/sja.v12i1.21118
- Martin-Guay, M. O., Paquette, A., Dupras, J., & Rivest, D. (2018). The new green revolution: Sustainable intensification of agriculture by intercropping. Science of the Total Environment, 615, 767–772. https://doi.org/10.1016/j.scitotenv.2017.10.024
- Matusso, J. M. M., Mugwe, J. N., & Mucheru-Muna, M. (2014). Potential role of cereal-legume intercropping systems in integrated soil fertility management in smallholder farming systems of Sub-Saharan Africa. Research Journal of Agriculture Environmental Management, 3(3), 162–174.
- Mead, R., & Willey, R. W. (1980). The concept of a ‘land equivalent ratio’and advantages in yields from intercropping. Experimental Agriculture, 16(3), 217–228. https://doi.org/10.1017/S0014479700010978
- Ministry of Agriculture and Forest. (2019). RNR census of Bhutan 2019. Renewable natural resources statistics division. Bhutan https://www.nsb.gov.bt/rnr-census-reports/
- Mudita, I. I., Chiduza, C., Richardson-Kageler, S., & Murungu, F. S. (2008). Evaluation of different strategies of intercropping maize (zea mays L.) and soya bean (glycine max (L.) merrill) under small-holder production in sub-humid Zimbabwe. Journal of Agronomy, 7(3), 237–243. https://doi.org/10.3923/ja.2008.237.243
- Mugi-Ngenga, E., Bastiaans, L., Anten, N. P. R., Zingore, S., & Giller, K. E. (2022). Immediate and residual-effects of sole and intercropped grain legumes in maize production systems under rain-fed conditions of Northern Tanzania. Field Crops Research, 287, 108656. https://doi.org/10.1016/j.fcr.2022.108656
- Muoneke, C. O., Ogwuche, M. A. O., & Kalu, B. A. (2007). Effect of maize planting density on the performance of maize/soybean intercropping system in a guinea savannah agroecosystem. African Journal of Agricultural Research, 2(12), 667–677.
- National Organic Programe. (2007). A guide to organic agriculture in Bhutan: National organic program, department of agriculture, minitry of agriculture. Royal Government of Bhutan.
- National Soil Service Center. (2003). Techincal report on the detailed soil survey RNRN research sub-center, Lingmethang. Department of Agriculture, Ministry of Agriculture and Forests.
- National Statistics Bureau. (2021). 2021 agriculture survey report. https://www.nsb.gov.bt/wp-content/uploads/dlm_uploads/2022/06/ASR2021-Book-for-WEB.pdf
- Neuhoff, D., Tashi, S., Rahmann, G., & Denich, M. J. O. A. (2014). Organic agriculture in Bhutan: Potential and challenges. Organic Agriculture, 4(3), 209–221. https://doi.org/10.1007/s13165-014-0075-1
- Odo, P. E. (1991). Evaluation of short and tall sorghum varieties in mixtures with cowpea in the Sudan savanna of Nigeria: Land equivalent ratio, grain yield and system productivity index. Experimental Agriculture, 27(4), 435–441. https://doi.org/10.1017/S0014479700019426
- Padhi, A. K., & Panigrahi, R. K. (2006). Effect of intercrop and crop geometry on productivity, economics, energetics and soil-fertility status of maize (zea mays)-based intercropping systems. Indian Journal of Agronomy, 51(3), 174–177. https://doi.org/10.59797/ija.v51i3.5001
- Pandey, P., & Bhambri, M. C. (2017). Growth response of maize to different crop arrangements and nutrient managements under maize (zea mays L.) and soybean (glycine max L.) intercropping system. Plant Archives, 17(2), 967–972.
- Paudel, B., Karki, T. B., Shah, S. C., & Chaudhary, N. K. (2015). Yield and economics of maize (zea mays)+ soybean (glycin max L. merrill) intercropping system under different tillage methods. World Journal of Agricultural Research, 3(2), 74–77.
- Rao, M. R., Rego, T. J., & Willey, R. W. (1987). Response of cereals to nitrogen in sole cropping and intercropping with different legumes. Plant and Soil, 101(2), 167–177. https://doi.org/10.1007/BF02370641
- Ren, Y. Y., Wang, X. L., Zhang, S. Q., Palta, J. A., & Chen, Y. L. (2017). Influence of spatial arrangement in maize-soybean intercropping on root growth and water use efficiency. Plant and Soil, 415(1–2), 131–144. https://doi.org/10.1007/s11104-016-3143-3
- Seran, T. H., & Brintha, I. (2009). Study on determining a suitable pattern of capsicum (capsicum annum L.)-vegetable cowpea (Vigna unguiculata L.) intercropping. Karnataka Journal of Agricultural Sciences, 22(5), 1153–1154.
- Seran, T. H., & Brintha, I. (2010). Review on maize based intercropping. Journal of Agronomy, 9(3), 135–145. https://doi.org/10.3923/ja.2010.135.145
- Sikirou, R., & Wydra, K. (2008). Effect of intercropping cowpea with maize or cassava on cowpea bacterial blight and yield/Der einfluß des mischkulturanbaus von augenbohne mit mais oder maniok auf den bakterienbrand und den ertrag. Journal of Plant Diseases and Protection, 115(4), 145–151. https://doi.org/10.1007/BF03356262
- Singh, U., Saad, A. A., & Singh, S. R. (2008). Production potential, biological feasibility and economic viability of maize (zea mays)-based intercropping systems under rainfed conditions of Kashmir valley. Indian Journal of Agricultural Sciences, 78(12), 1023.
- Stacciarini, T. D. C. V., de Castro, P. H. C., Borges, M. A., Guerin, H. F., Moraes, P. A. C., & Gotardo, M. (2010). Effect of row spacing reduction and increase in population density on agronomic traits of corn. Revista Ceres, 57(4), 516–519. https://doi.org/10.1590/S0034-737X2010000400012
- Undie, U. L., Uwah, D. F., & Attoe, E. E. (2012). Effect of intercropping and crop arrangement on yield and productivity of late season maize/soybean mixtures in the humid environment of south southern Nigeria. Journal of Agricultural Science, 4(4), 37–50. https://doi.org/10.5539/jas.v4n4p37
- Wang, Z. G., Jin, X., Bao, X. G., Li, X. F., Zhao, J. H., Sun, J. H., Christie, P., & Li, L. (2014). Intercropping enhances productivity and maintains the most soil fertility properties relative to sole cropping. Public Library of Science ONE, 9(12), e113984. https://doi.org/10.1371/journal.pone.0113984
- Wangmo, K. (2019). Effect of harvesting stages of maize on quality and consumption preferences of Tengma. Bhuanese Journal of Agriculture, 2(1), 131–142.
- Wang, Z., Zhao, X., Wu, P., He, J., Chen, X., Gao, Y., & Cao, X. (2015). Radiation interception and utilization by wheat/maize strip intercropping systems. Agricultural and Forest Meteorology, 204, 58–66. https://doi.org/10.1016/j.agrformet.2015.02.004
- Wei, H. E. (2016). Field evaluation of maize legume intercropping systems in the mid hills of Nepal. Wageningen University, Droevendaalsesteeg 1-6708 PB.
- Yang, C., Fan, Z., & Chai, Q. (2018). Agronomic and economic benefits of pea/maize intercropping systems in relation to N fertilizer and maize density. Agronomy, 8(4), 52.
- Yang, F., Liao, D., Fan, Y., Gao, R., Wu, X., Rahman, T., Yong, T., Liu, W., Liu, J., Du, J., Shu, K., Wang, X., & Yang, W. (2017). Effect of narrow-row planting patterns on crop competitive and economic advantage in maize–soybean relay strip intercropping system. Plant Production Science, 20(1), 1–11. https://doi.org/10.1080/1343943X.2016.1224553
- Yan, Y. H., Gong, W. Z., Yang, W. Y., Wan, Y., Chen, X. L., Chen, Z. Q., & Wang, L. Y. (2010). Seed treatment with uniconazole powder improves soybean seedling growth under shading by corn in relay strip intercropping system. Plant Production Science, 13(4), 367–374. https://doi.org/10.1626/pps.13.367
- Yang, F., Wang, X., Liao, D., Lu, F., Gao, R., Liu, W., Yong, T., Wu, X., Du, J., Liu, J., & Yang, W. (2015). Yield response to different planting geometries in maize–soybean relay strip intercropping systems. Agronomy Journal, 107(1), 296–304. https://doi.org/10.2134/agronj14.0263
- Yang, M., Zhang, Y., Qi, L., Mei, X., Liao, J., Ding, X., Vivanco, J. M., Fan, L., He, X., Vivanco, J. M., Li, C., Zhu, Y., & Zhu, S. (2014). Plant-plant-microbe mechanisms involved in soil-borne disease suppression on a maize and pepper intercropping system. Public Library of Science ONE, 9(12), e115052. https://doi.org/10.1371/journal.pone.0115052
- Yu, Y., Stomph, T. J., Makowski, D., Zhang, L., & Van Der Werf, W. (2016). A meta-analysis of relative crop yields in cereal/legume mixtures suggests options for management. Field Crops Research, 198, 269–279. https://doi.org/10.1016/j.fcr.2016.08.001
- Zhang, G., Yang, Z., & Dong, S. (2011). Interspecific competitiveness affects the total biomass yield in an alfalfa and corn intercropping system. Field Crops Research, 124(1), 66–73. https://doi.org/10.1016/j.fcr.2011.06.006