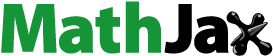
Abstract
Background
Dialysis-related amyloidosis (DRA) is a severe complication in end-stage kidney disease (ESKD) patients undergoing long-term dialysis treatment, characterized by the deposition of β2-microglobulin-related amyloids (Aβ2M amyloid). To inhibit DRA progression, hexadecyl-immobilized cellulose bead (HICB) columns are employed to adsorb circulating β2-microglobulin (β2M). However, it is possible that the HICB also adsorbs other molecules involved in amyloidogenesis.
Methods
We enrolled 14 ESKD patients using HICB columns for DRA treatment; proteins were extracted from HICBs following treatment and identified using liquid chromatography–linked mass spectrometry. We measured the removal rate of these proteins and examined the effect of those molecules on Aβ2M amyloid fibril formation in vitro.
Results
We identified 200 proteins adsorbed by HICBs. Of these, 21 were also detected in the amyloid deposits in the carpal tunnels of patients with DRA. After passing through the HICB column and hemodialyzer, the serum levels of proteins such as β2M, lysozyme, angiogenin, complement factor D and matrix Gla protein were reduced. These proteins acted in the Aβ2M amyloid fibril formation.
Conclusions
HICBs adsorbed diverse proteins in ESKD patients with DRA, including those detected in amyloid lesions. Direct hemoperfusion utilizing HICBs may play a role in acting Aβ2M amyloidogenesis by reducing the amyloid-related proteins.
Introduction
The life expectancy of patients undergoing dialysis for end-stage kidney disease (ESKD) has improved in recent years [Citation1–3]. However, long-term dialysis treatment can impair the quality of life of dialysis patients due to chronic kidney disease (CKD)-related systemic disorders [Citation4].
A serious complication that can occur in ESKD patients undergoing long-term dialysis is dialysis-related amyloidosis (DRA), which is caused by the deposition of amyloid fibrils related to β2-microglobulin (Aβ2M amyloid fibrils) [Citation5–9]. DRA primarily manifests as osteoarticular disorders, such as carpal tunnel syndrome (CTS), osteoarthropathy and destructive spondyloarthropathy. In some cases, DRA can also lead to visceral diseases such as heart failure and ischemic colitis. However, the exact pathophysiological mechanisms of DRA are not well understood.
Direct hemoperfusion using a column containing hexadecyl-immobilized cellulose beads (HICBs) in addition to regular hemodialysis (HD) effectively treats DRA [Citation10]. HICBs adsorb circulating β2-microglobulin (β2M) efficiently, and this column improves joint pain and physical function in dialysis patients with DRA [Citation11–13]. In vitro studies have suggested that HICBs also adsorb other proteins, including inflammatory cytokines [Citation14,Citation15] and protein-bound uremic toxins [Citation16]. Therefore, the HICB column not only removes β2M but also other proteins associated with Aβ2M amyloidogenesis in ESKD patients with DRA.
This study used a mass spectrometry (MS)-based proteomic approach to identify the proteins absorbed by the HICB column and those present in amyloid deposits. We further investigated the impact of the proteins on the in vitro formation of Aβ2M amyloid fibrils.
Methods
Design and study participants
We enrolled 28 ESKD patients who underwent HD treatment. Among them, 14 were assigned to the HICB-HD group, receiving HD with the HICB column, whereas the other 14 constituted the HD group, receiving regular HD treatment. These patients were recruited from six different dialysis units. The number of HD patients using HICB columns was very small, so we recruited patients for the HD group who were matched with them in terms of age and gender. All patients had no residual kidney function and underwent 4–5 h of HD three times weekly using standard bicarbonate dialysate (Na+: 140 mEq/L, K+: 2.0 mEq/L, Ca2+: 2.75 mEq/L, Mg2+: 1.0 mEq/L, Cl–: 112.25 mEq/L and HCO3–: 27.5 mEq/L) and dialyzers with synthetic polysulfone membranes ranging 1.6–2.1 m2 in size. In the HICB-HD group, the HICB column (Lixelle S-35, Kaneka Co., Tokyo, Japan) was used along with the hemodialyzer during dialysis sessions. The HICB column was connected to the hemodialyzer via an exclusive connection tube. The blood flow rate ranged from 180 to 250 mL/min, and the dialysate flow rate was 500 mL/min.
Baseline data, including age, sex, body mass index, cause of ESKD, systolic blood pressure, duration of dialysis treatment, single pool Kt/V urea, and serum levels of albumin, β2M, calcium, phosphate, intact parathyroid hormone, C-reactive protein and hemoglobin, were measured at the pre-dialysis session. Continuous variables are reported as medians (interquartile range). Additionally, we recorded the patients’ medical histories, specifically noting any occurrences of CTS, destructive spondyloarthropathy, joint arthropathy or trigger finger as these conditions are associated with DRA. The Mann–Whitney and Chi-square tests were used to assess the differences between the HICB-HD and HD groups.
This study was conducted in accordance with the Helsinki Declaration of 1975, as revised in 2013. The study protocol was approved by the Niigata University Ethics Committee (no. 2015-2352, 14 November 2012 and no. 2018-0015, 2 May 2018) and was registered with the University Hospital Medical Information Network Centre (UMIN000035264).
Extraction of proteins adsorbed by HICBs
Proteins adsorbed in HICB were extracted using the following procedure. First, HICBs were collected from the column after use in regular dialysis treatment of 14 patients with DRA. The collected HICBs were then washed five times with phosphate-buffered saline. Subsequently, proteins adsorbed by HICBs were extracted. To begin the extraction, the proteins underwent reductive alkylation using ammonium bicarbonate (25 mM, Sigma-Aldrich, St. Louis, MO), dithiothreitol (10 mM, Thermo Fisher Scientific, Waltham, MA), and iodoacetamide (55 mM, FUJIFILM Wako Pure Chemical Co., Osaka, Japan). Following this step, the HICBs were incubated overnight with trypsin protease (1 mg/mL, Thermo Fisher Scientific, Waltham, MA). Finally, the extracted peptides were obtained by treating the incubated mixture with trifluoroacetic acid (0.1%, Sigma-Aldrich, St. Louis, MO) and acetonitrile (40%, FUJIFILM Wako Pure Chemical Co., Osaka, Japan).
Extraction of proteins from amyloid lesions
Proteins were extracted from carpal tunnel tissues of dialysis patients who developed CTS. The remaining formalin-fixed paraffin-embedded tissue from diagnostic biopsy examinations was sectioned into 5-μm-thick slices. Congo red staining was used to identify the amyloid lesions. Subsequently, individual amyloid tissues, lesions staining with Congo red, and non-amyloid tissues, lesions not staining with Congo red, were collected using laser microdissection with the MMI CellCut Plus system (Molecular Machines & Industries, Eching, Germany) [Citation17]. Trypsin was used to extract the peptides, and MonoSpin C18 columns (GL Sciences, Tokyo, Japan) were utilized for purification, following the manufacturer’s instructions [Citation18].
LC–MS/MS analysis and protein identification
Samples from HICBs and carpal tunnel specimens were subjected to liquid chromatography–tandem mass spectrometry (LC–MS/MS) analysis using an Orbitrap Fusion mass spectrometer (Thermo Fisher Scientific, Waltham, MA) in data-dependent acquisition mode. Peptides were separated using a nanoflow LC system (Easy nanoLC1200, Thermo Fisher Scientific, Waltham, MA) equipped with a trap column (2 cm × 75 μm, Acclaim Pepmap 100 column) and a separation column (12.5 cm × 75 μm, NTCC-360) at a flow rate of 300 nL/min, employing a multistep gradient. The mobile phases consisted of water with 0.1% formic acid (A) and acetonitrile with 0.1% formic acid (B). A total of 500 ng of peptides were injected and eluted from the analytical column over a linear gradient from 2% B to 35% B in 143 min.
The mass spectrometer was operated in positive ion mode, performing MS and MS/MS scans in the m/z ranges of 350–1500 and 200–2000, respectively. Spectra with charge states 2 and 3 were fragmented using higher-energy collisional dissociation in a 3-s cycle time. The MS and MS/MS scan settings included a collision energy of 30%, an electrospray voltage of 2.0 kV, a capillary temperature of 250 °C, and an isolation window of 1.6 m/z.
The acquired data were analyzed using Proteome Discoverer TM software (version 2.2, Thermo Fisher Scientific, Waltham, MA) with SEQUEST HT algorithms for protein and peptide identification. The searches were performed against the UniProtKB/SWISS-Prot database (version 2015-08; Homo sapiens, 20,203 sequences). The parameters for the database searches included a precursor mass tolerance of ±10 ppm, a fragment ion mass tolerance of ±0.6 Da, trypsin enzyme specificity, and a maximum of two missed cleavages. Carbamidomethylation of cysteine was set as a fixed modification. False discovery rate (FDR) adjustment was performed using a percolator to achieve a 1% FDR at the peptide level.
For quantitative analysis, the normalized spectral index (SIN) value was used for label-free quantification [Citation19]. After extracting the UniProt accession containing the SIN value, proteins adsorbed on HICB were detected in eight out of 14 cases, and with SIN values greater than 1 were identified as specific proteins adsorbed on HICBs. The proteins identified in the synovial tissue of five out of eight dialysis patients with CTS were selected. Further, among them, proteins with SIN values greater than 1 were determined as the specific proteins in the tissues. In the CTS samples, DRA-related molecules were identified based on uniqueness or an SIN amyloid–non-amyloid lesion ratio greater than 2, which we named the amyloid-dominant proteins. The t-test was employed to evaluate the disparities in SIN levels among proteins within both amyloid and non-amyloid lesions. Functional annotation of proteins in amyloid lesions was performed using Metascape [Citation20]. The Metascape provides a comprehensive gene list annotation and analysis resource from functional enrichment, interactome analysis and gene annotation from over 40 knowledgebases.
Measurement of protein serum levels in dialysis patients
We selected proteins adsorbed by HICBs and detected abundantly in the amyloid lesions. Serum levels of proteins were measured using ELISA. The protein removal rates during passage through the dialyzer with and without the HICB column were calculated. The difference in the removal rate between the HD and HICB-HD groups was analyzed using the Wilcoxon signed-rank test. Statistical significance was set at p < .05. All statistical analyses and figure preparations were performed using SPSS version 26 (IBM, Armonk, NY) and GraphPad Prism (GraphPad Software, San Diego, CA), respectively.
In vitro Aβ2M amyloid fibril formation
The reaction mixtures consisted of recombinant β2M monomer (25 µM), sodium phosphate (pH 7, 50 mM), sodium chloride (100 mM), sodium dodecyl sulfate (0.25 mM), thioflavin T (ThT, 5 µM) and zirconia beads. We added four recombinant proteins – lysozyme (0–500 µg/mL, Nacalai Tesque, Kyoto, Japan), angiogenin (0–25 µg/mL, Elabscience, Houston, TX), complement factor D (0–25 µg/mL, BioLegend, San Diego, CA) and matrix Gla protein (0–25 µg/mL, CUSABIO, Houston, TX) – into the reaction mixture. This aimed to investigate how these molecules significantly adsorbed with an HICB column, impact the formation of Aβ2M amyloid fibrils. In vitro Aβ2M amyloid fibril formation was also examined with human serum albumin (0–10 mg/mL, Nacalai Tesque, Kyoto, Japan) and recombinant apolipoprotein E3 (0–300 µg/mL, Thermo Fisher Scientific, Waltham, MA) as controls which were shown in the previous study [Citation21–23]. The solutions (100 µL/well) were incubated at 37 °C with agitation (one minute on, 14 min off). The ThT fluorescence intensity was measured with excitation and emission wavelengths of 445 and 485 nm, respectively, using an SH-9000 microplate reader (Corona Electric Co., Hitachinaka, Japan). To show the differences between curves clearly, concentration-dependent increasing/decreasing curves in ThT fluorescence value and lag time are empirically fitted using the four-parameter logistic (4PL) model, known as the Hill-Slope model [Citation24]
where y is the intensity and x is the corresponding concentration. The EC50 and IC50 are half maximal (50%) effective and inhibitory concentrations, respectively, and A1/A′1 are their amplitude, and the slope/slope′ are Hill coefficients. The global fitting is performed by linking these EC50/IC50/slope/slope′ parameters between ThT fluorescence and lag time.
Aβ2M amyloid fibrils were observed using a transmission electron microscope (TEM). A 5-µL aliquot of the sample solution was placed on a collodion-coated copper grid (Nisshin EM Co., Tokyo, Japan) for 1 min, and excess solution was removed by blotting with filter paper. The grid was negatively stained with a 5-µL droplet of 2% (w/v) phosphotungstic acid for 1 min. The liquid on the grid was removed by blotting and then dried. TEM was performed using an H-7650 transmission electron microscope (Hitachi, Tokyo, Japan) operating at an acceleration voltage of 80 kV.
Results
Proteomic analysis of proteins adsorbed by HICBs
presents a summary of the characteristics of ESKD patients that underwent HD with the HICB column (HICB-HD group) and regular HD (HD group). In HICB-HD group, the median age was 68.3 years (IQR: 62.6–71.0 years) and 64.3% of the patients were males. The median dialysis vintage was 32.9 years (IQR: 28.1–38.5 years), and in 86% of cases, the primary cause of ESKD was chronic glomerulonephritis. ESKD patients in the HICB column group had a history of DRA, including CTS (100%) and destructive spondyloarthropathy (35.7%). LC–MS/MS analysis was conducted to identify the proteins adsorbed by HICBs, revealing the detection of 200 proteins. A list of the top 30 proteins with high SIN is provided in . The SIN value of β2M was 193.9 ± 143.4, which is consistent with previous reports indicating high levels of protein adsorption by HICBs [Citation10,Citation16].
Table 1. Baseline characteristics of ESKD patients undergoing HD with HICB column (HICB-HD) and regular HD.
Table 2. Top 30 proteins with high SIN extracted from HICBs after dialysis treatment of 14 DRA patients.
Proteomic analysis of proteins in amyloid lesions deposited in carpal tunnel synovia
Laser microdissection was used to collect both amyloid and non-amyloid lesions from the carpal tunnel synovia of eight dialysis patients with DRA. The characteristics of these patients are presented in Supplemental Table 1. A total of eight unique proteins were detected in the amyloid-positive lesions, and the amyloid-dominant proteins, which exhibited SIN ratios more than twice as high as those in non-amyloid lesions, comprised 29 proteins (Supplemental Table 2 and Figure 1). The SIN ratio of β2M was 3.4 (SIN value: 149.3 ± 125.2 in the amyloid-positive lesions and 43.4 ± 74.2 in the non-amyloid lesions, p = .018).
Adsorption properties of HICBs for amyloid-related proteins
and Supplemental Figure 1 present the results of proteomic analysis, which identified 21 proteins that were adsorbed by HICBs and were found predominantly in the amyloid lesions compared with non-amyloid lesions of the carpal tunnel synovia of ESKD patients. The functional annotation analysis of those proteins revealed that the term most enriched was the Amyloid fiber formation (R-HAS-977225) (Supplemental Figure 2). Subsequently, we evaluated the serum levels () and the removal rates () of these proteins during passage through the HICB column and dialyzer. The removal rates of β2M (HICB-HD: 80.9% [74.1–88.0] vs. HD: 43.7% [17.4–55.9], p < .001), lysozyme (HICB-HD: 81.0% [76.4–86.7] vs. HD: 15.4% [–1.23 to 36.3], p < .001), angiogenin (HICB-HD: 88.2% [75.1–91.0] vs. HD: 13.6% [–1.1 to 52.7], p < .001), matrix Gla protein (HICB-HD: 75.2% [56.6–83.2] vs. HD: 14.6% [6.0–39.3], p < .001) and complement factor D (HICB-HD: 55.6% [38.5–63.5] vs. HD: 24.8% [6.99–34.1], p < .001) were higher in the HICB-HD group compared with in the HD group.
Figure 1. The serum levels of amyloid-related proteins using the HICB columns and dialyzers. Serum levels of proteins in before and after passing through the dialyzer and HICB column (HICB-HD) was compared with the regular dialyzer (HD). HICB: hexadecyl-immobilized cellulose beads; HD: haemodialysis.
Figure 2. The removal rate of amyloid-related proteins using the HICB columns and dialyzers. Serum levels of proteins were measured using ELISAs. The removal rate using the dialyzer and HICB column (HICB-HD) was compared with the regular dialyzer (HD). The differences in the removal rate between HICB-HD and HD were analyzed using the Wilcoxon signed-rank test. HICB: hexadecyl-immobilized cellulose beads; HD: haemodialysis.
Table 3. Proteins adsorbed by HICBs and present in the amyloid lesions in carpal tunnel synovia of dialysis patients with DRA.
The effect of HICB-adsorbed proteins on β2M amyloid fibril formation in vitro
To examine the effect of HICB-adsorbed proteins on Aβ2M amyloid fibril formation, we conducted in vitro amyloid fibril formation assay with concentrations in the physiological range of lysozyme, angiogenin, complement factor D and matrix Gla protein (). Lysozyme caused an increase in ThT fluorescence at lower concentrations ranging 0.001–1 μg/mL (), and TEM revealed the presence of extended amyloid fibrils at 0.1 μg/mL lysozyme (). However, at concentrations exceeding 2 μg/mL, lysozyme inhibited amyloid fibril formation (), that is, ThT fluorescence decreased, and lag time increased possibly owing to precipitation via an interaction between negatively charged β2M (the isoelectric point (pI) of β2M is 6.1) and positively charged lysozyme (the pI of lysozyme is 9.3) at a pH 7. Angiogenin exhibited concentration-dependent promotion of amyloid fibril formation reactions up to 0.001–10 µg/mL, with reduced activity at 25 µg/mL (). Complement factor D demonstrated concentration-dependent promotion of the reaction up to 0.001–1 µg/mL, with diminished activity at 10 µg/mL and above (). Matrix Gla protein concentration-dependently promoted the reaction within the range of 0.001–25 µg/mL (). When human serum albumin and apolipoprotein E3 were added in the reaction mixture, those molecules showed the inhibitory effect on the Aβ2M amyloid fibril formation (Supplemental Figure 4). The apparent EC50/IC50 values calculated based on the 4PL model are shown in Supplemental Table 3.
Figure 3. In vitro Αβ2M amyloid fibril formation with the amyloid-related proteins adsorbed by HICBs. Time courses of the aβ2M amyloid fibril formation with lysozyme (A), angiogenin (B), complement factor D (C) and matrix Gla protein (D) were monitored using a thioflavin T (ThT) fluorescence assay. Maximum values of ThT fluorescence are shown in the reaction with lysozyme (E), angiogenin (F), complement factor D (G) and matrix Gla protein (H). Morphological analysis was performed using TEM in the reaction with lysozyme (I), angiogenin (J), complement factor D (K) and matrix Gla protein (L). The concentrations are shown at the top of the figures. Scale bars = 200 nm. HICB: hexadecyl-immobilized cellulose beads.
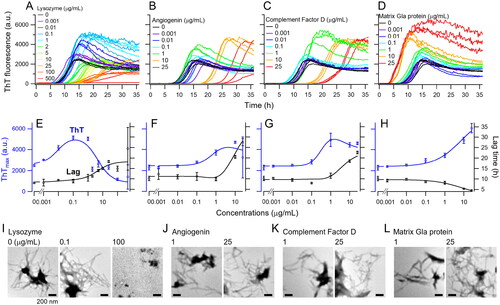
Discussion
In this study, HICBs were found to adsorb various circulating proteins, some of which were detected in amyloid lesions of ESKD patients with DRA. In vitro assays demonstrated that lysozyme, angiogenin, complement factor D and matrix Gla protein, which were adsorbed with HICBs and detected in amyloid lesion dominantly, acted the Aβ2M amyloid fibril formation.
DRA is a systemic amyloidosis that frequently occurs in ESKD patients undergoing long-term dialysis [Citation25]. The factors contributing to DRA development have been suggested through in vitro studies on amyloid fibril formation and extension [Citation26,Citation27]. Several biological molecules, including glycosaminoglycans [Citation28,Citation29], collagen [Citation30], lysophospholipids [Citation31], non-esterified fatty acids [Citation32] and polyphosphates [Citation33], promote these reactions in vitro. This suggests that the accumulation of multiple biological molecules enhances Aβ2M amyloid fibril formation and extension in ESKD patients.
The HICB column was developed for the direct hemoperfusion method to efficiently remove β2M in ESKD patients with DRA. Its use has resulted in the alleviation of symptoms associated with DRA, such as stiffness, joint pain and bone cysts [Citation11,Citation34]. Several clinical studies [Citation10,Citation16] have suggested that the HICB column adsorbs several proteins in addition to β2M, and it effectively adsorbs protein-binding molecules like indoxyl sulfate and p-cresyl sulfate [Citation16]. The use of HICBs also reduces the levels of inflammatory cytokines, including interleukin 1β and interleukin 6, in an ESKD patient with DRA [Citation35]. In this study, 200 proteins were adsorbed by the HICB column. Of these, 21 proteins were found in significantly higher amounts in the amyloid lesions compared with in the non-amyloid lesions ( and Supplemental Figure 1). Notably, lysozyme, angiogenin, complement factor D and matrix Gla protein were efficiently adsorbed by the HICB column compared with hemodialyzer alone, and those molecules were found to act Aβ2M amyloid fibril formation in vitro ().
The objectives of this study were to identify proteins: (1) abundant in amyloid, (2) removed by HICB columns and (3) potentially acting as factors for Aβ2M amyloid fibril formation. Therefore, the focus was on suggesting candidate molecules associated with the promotion rather than inhibition of amyloid fibril formation. Lysozyme at physiological low concentrations promoted the formation of Aβ2M amyloid fibrils; however, exposure to a high dose inhibited this reaction (). Previous studies reported that lysozyme inhibited amyloid fibril formation induced with Aβ protein [Citation35] and serum amyloid A [Citation36]. In our study, a higher concentration of lysozyme inhibited the formation of Aβ2M amyloid fibrils, likely due to the binding effect of lysozyme with β2M monomers. On the other hand, the lower concentrations of lysozyme promote amyloid fibril formation, possibly through molecular interactions, such as the conformational change of β2M monomers and the stabilization of amyloid fibrils. Angiogenin belongs to the vertebrate-specific RNase A superfamily and plays a crucial role in inducing angiogenesis, which is necessary for promoting the growth and metastatic spread of cancer cells [Citation36]. A review article suggested that complement factor D is secreted from adipocytes, activates the alternative pathway, and associates with cancer stem cell interactions [Citation37]. While angiogenin and complement factor D are identified as middle molecular weight of uremic toxins [Citation38], there is no report demonstrating its role in DRA. Matrix Gla protein with vitamin K suppresses vascular calcification accelerated with kidney disease [Citation39]. Bone cysts are formed with the progression of DRA, and it is possible that matrix Gal protein is associated with the Aβ2M amyloidogenesis. The effects of the HICB column on DRA progression are expected to be attributed not only to β2M removal but also to the removal of other molecules associated with amyloidogenesis in ESKD patients. This study proposes the involvement of the four proteins through comprehensive analysis; however, further investigation is necessary to explore the detailed mechanisms of their molecular roles in Aβ2M amyloidogenesis.
We confirmed that albumin and apolipoprotein E inhibited the reaction, suggesting our in vitro Aβ2M amyloid fibril formation is proper evaluation to understand the molecular interactions for amyloidogenesis. It is possible that various proteins adsorbed with HICBs promote or inhibit the amyloid fibril formation. The non-amyloid lesions may also contain the proteins to inhibit the amyloid fibril formation, and understanding the inhibitory effect will be important using further proteomic approach.
This study had several limitations. First, the cross-sectional study design and small sample size might limit the generalizability of the findings. However, by employing a proteomic approach, we were able to conduct detailed examinations of proteins adsorbed by HICBs and demonstrate the multifunctional effects of the HICB column. It is unclear whether the concentrations that promote or inhibit amyloid fibril formation in vitro correspond to the blood concentrations. Additionally, the concentrations of these molecules in the blood may differ from those in tissues. For example, it is highly likely that matrix Gla protein is more abundant in tissues than in the bloodstream. In this regard, since it is currently unclear whether molecules adsorbed to HICB have a promoting effect on amyloid fibril formation in tissues. To comprehend the clinical significance of candidate molecules for DRA, larger observational or interventional studies with a substantial number of participants are warranted to show the effects of the HICB column on DRA.
In conclusion, HICBs adsorbed diverse proteins in ESKD patients with DRA, including those detected in amyloid lesions. Among them, lysozyme, angiogenin, complement factor D and matrix Gla protein were shown to act on Aβ2M amyloid fibril formation in vitro. The multifaceted adsorption properties of HICBs suggest that the use of HICB column is a blood purification method to influence the progression of DRA.
Author contributions
All authors contributed to the study’s conception, design, analysis and interpretation of data. The specific contributions are as follows: conception and study design: S.Y., F.G., Y.G., T.Y. and I.N.; sampling: S.Y., K.G., K.O., N.I., H.S., I.E. and R.A.; proteomic analysis: S.Y., Keiko Yamamoto (K.Y.1), Y.H. and M.S.; measurement of serum levels of proteins: S.Y., M.K. and M.D.; in vitro amyloid fibril formation: S.Y., Keiichi Yamaguchi (K.Y.2), K.N. and Y.G. Data interpretation: S.Y., S.G. and I.N.; manuscript drafting: S.Y., K.Y.1 and K.Y.2. Supervision and mentorship: Y.G., S.G., F.G., T.Y. and I.N. Each author contributed important intellectual content during the drafting or revision of the manuscript and takes responsibility for the overall work, ensuring that any questions regarding the accuracy or integrity of any part of the study are appropriately investigated and resolved.
Abbreviations | ||
CKD | = | chronic kidney disease |
CTS | = | carpal tunnel syndrome |
DRA | = | dialysis-related amyloidosis |
ESKD | = | end-stage kidney disease |
FDR | = | false discovery rate |
HD | = | hemodialysis |
HICBs | = | hexadecyl-immobilized cellulose beads |
LC–MS/MS | = | liquid chromatography–tandem mass spectrometry |
MS | = | mass spectrometry |
SIN | = | normalized spectral index |
TEM | = | transmission electron microscope |
ThT | = | thioflavin T |
β2M | = | β2-microglobulin |
Supplemental Material
Download MS Word (562.8 KB)Acknowledgements
The authors thank Kaneka Co. for the technical assistance.
Disclosure statement
This study was conducted via a collaboration between Niigata University and Kaneka Co. SY has received honoraria from Kyowa Kirin and research funding from Toray Medical Co., Ltd and Kaneka Co. The other authors declare no competing interests.
Data availability statement
Restrictions apply to the availability of the data analyzed in this study to preserve patient confidentiality. Data will be shared upon request to the corresponding author.
Additional information
Funding
References
- Harding JL, Morton JI, Shaw JE, et al. Changes in excess mortality among adults with diabetes-related end-stage kidney disease: a comparison between the USA and Australia. Nephrol Dial Transplant. 2022;37(10):2004–2013. doi: 10.1093/ndt/gfab315.
- Johansen KL, Chertow GM, Foley RN, et al. US Renal Data System 2020 Annual Data Report: epidemiology of kidney disease in the United States. Am J Kidney Dis. 2021;77(4 Suppl. 1):A7–A8. doi: 10.1053/j.ajkd.2021.01.002.
- Nitta K, Goto S, Masakane I, et al. Annual dialysis data report for 2018, JSDT Renal Data Registry: survey methods, facility data, incidence, prevalence, and mortality. Ren Replace Ther. 2020;6(1):41. doi: 10.1186/s41100-020-00286-9.
- Unruh ML, Hess R. Assessment of health-related quality of life among patients with chronic kidney disease. Adv Chronic Kidney Dis. 2007;14(4):345–352. doi: 10.1053/j.ackd.2007.07.011.
- Gejyo F, Yamada T, Odani S, et al. A new form of amyloid protein associated with chronic hemodialysis was identified as beta 2-microglobulin. Biochem Biophys Res Commun. 1985;129(3):701–706. doi: 10.1016/0006-291x(85)91948-5.
- Gejyo F, Arakawa M. Dialysis amyloidosis: current disease concepts and new perspectives for its treatment. Contrib Nephrol. 1990;78:47–60, discussion 59–60. doi: 10.1159/000418269.
- Charra B, Calemard E, Uzan M, et al. Carpal tunnel syndrome, shoulder pain and amyloid deposits in long-term haemodialysis patients. Proc Eur Dial Transplant Assoc Eur Ren Assoc. 1985;21:291–295.
- Koch KM. Dialysis-related amyloidosis. Kidney Int. 1992;41(5):1416–1429. doi: 10.1038/ki.1992.207.
- Portales-Castillo I, Yee J, Tanaka H, et al. Beta-2 microglobulin amyloidosis: past, present, and future. Kidney360. 2020;1(12):1447–1455. doi: 10.34067/KID.0004922020.
- Gejyo F, Kawaguchi Y, Hara S, et al. Arresting dialysis-related amyloidosis: a prospective multicenter controlled trial of direct hemoperfusion with a beta2-microglobulin adsorption column. Artif Organs. 2004;28(4):371–380. doi: 10.1111/j.1525-1594.2004.47260.x.
- Gejyo F, Amano I, Ando T, et al. Survey of the effects of a column for adsorption of beta2-microglobulin in patients with dialysis-related amyloidosis in Japan. Ther Apher Dial. 2013;17(1):40–47. doi: 10.1111/j.1744-9987.2012.01130.x.
- Yamamoto Y, Hirawa N, Yamaguchi S, et al. Long-term efficacy and safety of the small-sized beta2-microglobulin adsorption column for dialysis-related amyloidosis. Ther Apher Dial. 2011;15(5):466–474. doi: 10.1111/j.1744-9987.2011.00937.x.
- Abe T, Uchita K, Orita H, et al. Effect of beta(2)-microglobulin adsorption column on dialysis-related amyloidosis. Kidney Int. 2003;64(4):1522–1528. doi: 10.1046/j.1523-1755.2003.00235.x.
- Kutsuki H. Beta(2)-microglobulin-selective direct hemoperfusion column for the treatment of dialysis-related amyloidosis. Biochim Biophys Acta. 2005;1753(1):141–145. doi: 10.1016/j.bbapap.2005.08.007.
- Tsuchida K, Takemoto Y, Nakamura T, et al. Lixelle adsorbent to remove inflammatory cytokines. Artif Organs. 1998;22(12):1064–1067. doi: 10.1046/j.1525-1594.1998.06179.x.
- Yamamoto S, Sato M, Sato Y, et al. Adsorption of protein-bound uremic toxins through direct hemoperfusion with hexadecyl-immobilized cellulose beads in patients undergoing hemodialysis. Artif Organs. 2018;42(1):88–93. doi: 10.1111/aor.12961.
- Yoshida Y, Nameta M, Kuwano M, et al. Proteomic approach to human kidney glomerulus prepared by laser microdissection from frozen biopsy specimens: exploration of proteome after removal of blood-derived proteins. Proteomics Clin Appl. 2012;6(7–8):412–417. doi: 10.1002/prca.201200016.
- Hirao Y, Saito S, Fujinaka H, et al. Proteome profiling of diabetic mellitus patient urine for discovery of biomarkers by comprehensive MS-based proteomics. Proteomes. 2018;6(1):9. doi: 10.3390/proteomes6010009.
- Griffin NM, Yu J, Long F, et al. Label-free, normalized quantification of complex mass spectrometry data for proteomic analysis. Nat Biotechnol. 2010;28(1):83–89. doi: 10.1038/nbt.1592.
- Zhou Y, Zhou B, Pache L, et al. Metascape provides a biologist-oriented resource for the analysis of systems-level datasets. Nat Commun. 2019;10(1):1523. doi: 10.1038/s41467-019-09234-6.
- Nakajima K, Yamaguchi K, Noji M, et al. Macromolecular crowding and supersaturation protect hemodialysis patients from the onset of dialysis-related amyloidosis. Nat Commun. 2022;13(1):5689. doi: 10.1038/s41467-022-33247-3.
- Myers SL, Jones S, Jahn TR, et al. A systematic study of the effect of physiological factors on beta2-microglobulin amyloid formation at neutral pH. Biochemistry. 2006;45(7):2311–2321. doi: 10.1021/bi052434i.
- Yamaguchi I, Hasegawa K, Takahashi N, et al. Apolipoprotein E inhibits the depolymerization of beta 2-microglobulin-related amyloid fibrils at a neutral pH. Biochemistry. 2001;40(29):8499–8507. doi: 10.1021/bi0027128.
- Beck B, Chen YF, Dere W, et al. Assay operations for SAR support. In: Markossian S, Grossman A, Brimacombe K, editors. Assay guidance manual. Bethesda (MD): Eli Lilly & Company and the National Center for Advancing Translational Sciences; 2004.
- Buxbaum JN, Dispenzieri A, Eisenberg DS, et al. Amyloid Nomenclature 2022: update, novel proteins, and recommendations by the International Society of Amyloidosis (ISA) Nomenclature Committee. Amyloid. 2022;29(4):213–219. doi: 10.1080/13506129.2022.2147636.
- Naiki H, Okoshi T, Ozawa D, et al. Molecular pathogenesis of human amyloidosis: lessons from beta2-microglobulin-related amyloidosis. Pathol Int. 2016;66(4):193–201. doi: 10.1111/pin.12394.
- Yamamoto S. Molecular mechanisms underlying uremic toxin-related systemic disorders in chronic kidney disease: focused on beta(2)-microglobulin-related amyloidosis and indoxyl sulfate-induced atherosclerosis—Oshima Award Address 2016. Clin Exp Nephrol. 2019;23(2):151–157. doi: 10.1007/s10157-018-1588-9.
- Yamamoto S, Yamaguchi I, Hasegawa K, et al. Glycosaminoglycans enhance the trifluoroethanol-induced extension of beta 2-microglobulin-related amyloid fibrils at a neutral pH. J Am Soc Nephrol. 2004;15(1):126–133. doi: 10.1097/01.asn.0000103228.81623.c7.
- Relini A, De Stefano S, Torrassa S, et al. Heparin strongly enhances the formation of beta2-microglobulin amyloid fibrils in the presence of type I collagen. J Biol Chem. 2008;283(8):4912–4920. doi: 10.1074/jbc.M702712200.
- Hoop CL, Zhu J, Bhattacharya S, et al. Collagen I weakly interacts with the beta-sheets of beta(2)-microglobulin and enhances conformational exchange to induce amyloid formation. J Am Chem Soc. 2020;142(3):1321–1331. doi: 10.1021/jacs.9b10421.
- Ookoshi T, Hasegawa K, Ohhashi Y, et al. Lysophospholipids induce the nucleation and extension of beta2-microglobulin-related amyloid fibrils at a neutral pH. Nephrol Dial Transplant. 2008;23(10):3247–3255. doi: 10.1093/ndt/gfn231.
- Hasegawa K, Tsutsumi-Yasuhara S, Ookoshi T, et al. Growth of beta(2)-microglobulin-related amyloid fibrils by non-esterified fatty acids at a neutral pH. Biochem J. 2008;416(2):307–315. doi: 10.1042/BJ20080543.
- Zhang CM, Yamaguchi K, So M, et al. Possible mechanisms of polyphosphate-induced amyloid fibril formation of beta(2)-microglobulin. Proc Natl Acad Sci U S A. 2019;116(26):12833–12838. doi: 10.1073/pnas.1819813116.
- Kuragano T, Inoue T, Yoh K, et al. Effectiveness of beta(2)-microglobulin adsorption column in treating dialysis-related amyloidosis: a multicenter study. Blood Purif. 2011;32(4):317–322. doi: 10.1159/000330332.
- Nakano T, Nagae H, Murakami N, et al. Fever associated with severe dialysis-related amyloidosis. CEN Case Rep. 2012;1(2):112–116. doi: 10.1007/s13730-012-0024-3.
- Sultana MF, Abo H, Kawashima H. Human and mouse angiogenins: emerging insights and potential opportunities. Front Microbiol. 2022;13:1022945. doi: 10.3389/fmicb.2022.1022945.
- Khaledian B, Thibes L, Shimono Y. Adipocyte regulation of cancer stem cells. Cancer Sci. 2023;114(11):4134–4144. doi: 10.1111/cas.15940.
- Duranton F, Cohen G, De Smet R, et al. Normal and pathologic concentrations of uremic toxins. J Am Soc Nephrol. 2012;23(7):1258–1270. doi: 10.1681/ASN.2011121175.
- Kaur R, Singh R. Mechanistic insights into CKD-MBD-related vascular calcification and its clinical implications. Life Sci. 2022;311(Pt B):121148. doi: 10.1016/j.lfs.2022.121148.