Abstract
Objective
Estetrol (E4) represents a novel estrogen of interest to relieve vasomotor symptoms. E4 activates the nuclear estrogen receptor α (ERα) but antagonizes the estradiol ERα-dependent membrane-initiated steroid signaling pathway. The distinct pharmacological properties of E4 could explain its low impact on hemostasis. This study aimed to assess the effect of E4 on coagulation in postmenopausal women, using the thrombin generation assay (TGA).
Methods
Data were collected from a multicenter, randomized, placebo-controlled, dose-finding study in postmenopausal women (NCT02834312). Oral E4 (2.5 mg, n = 42; 5 mg, n = 29; 10 mg, n = 34; or 15 mg, n = 32) or placebo (n = 31) was administered daily for 12 weeks. Thrombograms and TGA parameters were extracted for each subject at baseline and after 12 weeks of treatment.
Results
After 12 weeks of treatment, all treatment groups showed a mean thrombogram (±95% confidence interval [CI] of the mean) within the reference ranges, that is, the 2.5th–97.5th percentile of all baseline thrombograms (n = 168), as well as for TGA parameters.
Conclusions
The intake of E4 15 mg for 12 weeks led to significant but not clinically relevant changes compared to baseline as the mean values (±95% CI of the mean) remained within reference ranges, demonstrating a neutral profile of this estrogen on hemostasis.
摘要
目标: 雌四醇(E4)代表一种新型雌激素, 用于缓解血管舒缩症状。E4激活核雌激素受体α(ERα), 但拮抗雌二醇依赖的ERα膜启动类固醇信号传导途径。E4的独特药理特性可以解释其对血液稳态影响低。本研究使用凝血酶生成测定(TGA), 评估E4对绝经后妇女凝血作用的影响。
方法: 数据收集自一项多中心、随机、安慰剂对照、剂量发现研究, 对象为绝经后妇女(NCT02834312)。口服E4(2.5mg, n = 42;5mg, n = 29;10mg, n = 34;或15mg, n = 32)或安慰剂(n = 31), 每日一次, 持续12周。在治疗前和治疗12周后, 为每个受试者提取凝血图和TGA参数。
结果: 治疗12周后, 所有治疗组显示的平均凝血图(±95%置信区间[CI]的平均值)均在参考范围内, 即所有基线凝血图(n = 168)的2.5至97.5百分位数, 以及TGA参数。
结论: 摄入E4 15mg持续12周导致与基线相比有显著但与临床无关的变化, 因为平均值(±95% CI的平均值)仍在参考范围内, 这表明这种雌激素对凝血的影响是中性的。
Clinical trial number:
Introduction
The risk of developing venous thromboembolism (VTE) is still a matter of concern for many women starting oral hormonal therapy (HT) [Citation1]. The risk of VTE is increased especially with preparations containing oral conjugated equine estrogen (CEE) [Citation2], is less pronounced with oral estradiol (E2) but is lowest with transdermal E2 preparations [Citation2,Citation3]. First ever users of HT had a twofold increased risk of pulmonary embolism within 4 months after starting therapy, compared with non-users [Citation4].
The VTE risk has been explained, at least in part, by the hemostatic imbalance induced by HT which causes changes in plasma levels of almost all proteins involved in coagulation and fibrinolysis [Citation5–8]. Although these changes might be considered relatively modest when measured separately, they have a supra-additive effect leading to a procoagulable state responsible for this increased risk of VTE [Citation9]. These hormonal changes are also associated with activated protein C (APC) resistance which results from increases in FII, FVIII or FX levels and/or decreases in protein S and tissue factor pathway inhibitor levels [Citation10–13].
The endogenous thrombin potential (ETP)-based APC resistance assay is one of the most sensitive assays toward APC resistance acquired following HT and has been linked to an increased risk of VTE in women using HT [Citation11–16]. This technique relies on the thrombin generation assay (TGA) which allows to obtain a thrombogram, that is, a visual and quantitative representation of the amount of thrombin generated over time in a testing cupule [Citation17]. On top of using this marker for the determination of APC resistance, the parameters of the thrombogram can also be exploited [Citation18] to provide information on the prothrombotic tendency independently of the resistance toward exogenous APC [Citation19–21]. As the use of HT and other known hypercoagulable states have been shown to enhance thrombin generation in vitro [Citation5,Citation22–25], the development of convenient, easily administrable HT with no or low impact on thrombin generation and the associated risk of thrombosis is of utmost importance.
Estetrol (E4) is the first native estrogen with selective tissue activity (NEST) and represents a novel estrogen of interest to relieve vasomotor symptoms [Citation26–29] and genitourinary syndrome of menopause [Citation30]. Indeed, like other estrogens, E4 activates the nuclear estrogen receptor α (ERα), but in contrast to other estrogens, induces limited activity via ERα-dependent membrane-initiated steroid signaling in several tissues including the breast, and antagonizes this pathway in the presence of E2, thereby uniquely uncoupling nuclear and membrane activation.
E4 demonstrated a low impact on the liver with minimal effects on lipids, lipoproteins, sex hormone-binding globulin (SHBG) and several coagulation and fibrinolytic proteins [Citation31].
To further delineate the minimal effective dose of E4 for the treatment of postmenopausal symptoms, the E4Relief Phase 2 trial was subsequently designed and revealed that E4 at a dose of 15 mg daily is the minimum effective daily dose for the treatment of vasomotor symptoms [Citation32,Citation33]. This study also allowed to confirm the low impact of E4 on hemostasis and supports the hypothesis that E4 may be associated with a lower risk of thrombosis, like its use in contraception [Citation34]. Nevertheless, while some coagulation factors such as protein S and tissue factor pathway inhibitor were slightly impacted by rising doses of E4, the synergistic effect of these changes on hemostasis could not be fully captured by the singular measurements proposed in these initial hemostasis investigations [Citation35,Citation36]. Therefore, as TGA allows to assess the coagulation process in its entirety and as it has been shown to be sensitive to the synergistic hemostatic alterations induced by HT [Citation17], we present here the impact of multiple ranging doses of E4 on TGA.
Materials and methods
Trial design
This was a multicenter, randomized, double-blind, placebo-controlled, phase 2, dose-finding trial in hysterectomized and non-hysterectomized postmenopausal women (ClinicalTrials.gov NCT02834312, EudraCT 2015-004018-44). The trial was approved by the independent ethics committees of participating centers and conducted in accordance with the ethical principles established by the Declaration of Helsinki and the International Conference on Harmonization – Good Clinical Practice (ICH E6 [R2]) guidelines. All participants gave written informed consent and had the right to withdraw at any time.
Trial population and treatments
Enrolled (n = 260) were postmenopausal women (amenorrhea for at least 12 consecutive months with follicle stimulating hormone [FSH] > 40 IU/l; or amenorrhea for at least 6 months with FSH >40 IU/l and E2 < 20 pg/ml; or at least 6 weeks after surgical bilateral oophorectomy with FSH >40 IU/l), aged 40–65 years with a body mass index (BMI) of 18.0–35.0 kg/m2. Women with an intact uterus were eligible if transvaginal ultrasound showed a bilayer endometrial thickness ≤5 mm. More detailed selection criteria have been described previously, as well as potential washout conditions [Citation32]. Eligible participants (n = 257) were randomly allocated (1:1:1:1:1) to daily oral treatment with E4 2.5 mg, 5 mg, 10 mg or 15 mg (SEQENS VLG CHEM, France), or placebo, for 12 weeks. E4 and placebo were manufactured by Haupt Pharma Munster GmbH, Germany and packaged and supplied by Almac, UK. All non-hysterectomized women received 10 mg dydrogesterone (Dufaston®, Abbott Italy and Mylan Italy, supplied by Almac, UK and BGP Products, Hoofddorp, The Netherlands) once daily for 14 days, after completion of E4 or placebo treatment.
Included in the thrombin generation analysis were all participants with a baseline assessment and a post-dose assessment at week 12, with treatment compliance ≥80% and no changes in concomitant medication during trial treatment that could affect any of the hemostatic parameters (TGA set population n = 168; see Supplementary Figure 1).
Measurements and outcome parameters
Trial visits were scheduled for screening (visit 1), randomization (visit 2, baseline), after 4 weeks of treatment (visit 3), after 12 weeks of treatment (visit 4 [W12], end of treatment) and after dydrogesterone treatment in non-hysterectomized women only (visit 5, follow-up). Blood samples for the assessment of hemostasis and metabolic parameters were taken at baseline and end of treatment (W12). Samples were analyzed by central laboratories (BARC, Belgium and InterLab GmbH/Synlab, Germany), and samples for the normalized APC sensitivity ratio were analyzed by QUALIblood, Belgium. Outcomes on several hemostasis, metabolic and bone parameters have been reported previously [Citation36].
The TGA was performed on a Calibrated Automated Thrombogram (Diagnostica Stago, Asnières-sur-Seine, France) using STG-ThromboScreen® (Diagnostica Stago) as a triggering reagent. A complete description of the method is reported in detail elsewhere [Citation18]. Thrombogram parameters were integrated using the Thrombinoscope software (Thrombinoscope bv, version 5.0) and the following TGA parameters were extracted from the thrombin generation curve: the lag time, corresponding to the start of thrombin generation (expressed in minutes); the peak height, corresponding to the maximal concentration of thrombin generated (expressed in nanomoles); the time to peak, corresponding to the time to reach the peak (expressed in minutes); the ETP, corresponding to the area under the curve (expressed in nanomolar minutes); and the mean velocity rate index (mVRI), corresponding to the maximal rate of thrombin generation (expressed in nanomoles per minute). Supplementary Figure 2 provides a representation of a thrombogram with the corresponding TGA parameters.
Statistical analysis
Statistical analysis was performed using GraphPad version 9.3.1 (GraphPad Prism 9.3.1 for macOs, GraphPad Software, San Diego, CA, USA; www.graphpad.com). Descriptive statistics were used to analyze the data (n, mean, standard deviation, median, minimum–maximum range, 10th–90th percentile and 95% confidence interval [CI]). Changes from baseline were assessed using ordinary one-way analysis of variance. A Dunnett’s multiple comparisons test with a single pooled variance was then run to assess the difference between the E4 groups and the placebo group. Reference ranges for thrombin generation and associated parameters are reported as the 2.5th–97.5th percentile of the baseline of the entire cohort, in accordance with the definition of the reference intervals as reported in the Clinical & Laboratory Standards Institute (CLSI) EP-28-A3C [Citation37]. Within a particular group, a comparison of the data at baseline and after 12 weeks was performed using a paired t-test. All statistical tests were evaluated with a level of significance of 0.05.
Results
Study population
The trial was conducted in 35 centers in Europe (Belgium, Czech Republic, Great Britain, Ireland and Poland). In total, 260 women were randomized, of which 257 women received treatment. A total of 168 participants were included in the analysis of TGA parameters (E4 2.5 mg, n = 42; E4 5 mg, n = 29; E4 10 mg, n = 34; E4 15 mg, n = 32; placebo, n = 31) (Supplemental Figure 1). Baseline characteristics in each of the five groups are summarized in . Mean age ranged between 53.0 and 54.8 years, mean BMI ranged between 25.1 and 26.7 kg/m2 and mean time since menopause ranged between 3.5 and 5.2 years among the different treatment groups. Treatment groups were comparable for all characteristics assessed.
Table 1. Baseline characteristics by treatment group of all participants who received at least one dose of oral estetrol (E4) or placebo.
Thrombograms and TGA parameters
Reference range and absolute values at baseline and after 12 weeks of treatment
Analyses of thrombograms and resulting TGA parameters were performed on data from the 168 subjects in the TGA set population.
The baseline thrombogram (n = 168, entire cohort) and those after 12 weeks of treatment with either E4 2.5 mg, 5 mg, 10 mg or 15 mg, or placebo are shown in . The mean baseline thrombogram and its 2.5th–97.5th percentile represents the reference interval. The reference interval was 2.1–4.3 min for the lag time, 4.3–8.8 min for the time to peak, 146.1–398.5 nM for the peak height, 1013–1893 nM*min for the ETP and 32.5–201 nM/min for the mVRI. After 12 weeks of treatment, the mean (95% CI of the mean) thrombograms were all within the reference range.
Figure 1. Mean (2.5th–97.5th percentile) thrombogram of the entire baseline cohort (n = 168) and mean thrombograms (95% confidence interval [CI] of the mean) after 12 weeks of treatment. Mean thrombogram of the entire baseline cohort is represented by the yellow line and 2.5th–97.5th percentiles, indicating reference ranges, are represented by yellow dotted lines. Women treated with placebo or E4 2.5 mg, 5.0 mg, 10 mg and 15 mg are represented in blue, red, orange, pink and purple, respectively. None of the mean thrombograms were outside the reference range established at baseline, demonstrating the low impact of estetrol (E4) on the entire coagulation process.
![Figure 1. Mean (2.5th–97.5th percentile) thrombogram of the entire baseline cohort (n = 168) and mean thrombograms (95% confidence interval [CI] of the mean) after 12 weeks of treatment. Mean thrombogram of the entire baseline cohort is represented by the yellow line and 2.5th–97.5th percentiles, indicating reference ranges, are represented by yellow dotted lines. Women treated with placebo or E4 2.5 mg, 5.0 mg, 10 mg and 15 mg are represented in blue, red, orange, pink and purple, respectively. None of the mean thrombograms were outside the reference range established at baseline, demonstrating the low impact of estetrol (E4) on the entire coagulation process.](/cms/asset/fbdfdca8-0c06-460a-acd7-24f00985ee0a/icmt_a_2292066_f0001_c.jpg)
Mean values of each TGA parameter for each subgroup at baseline and after 12 weeks of treatment are presented in . The evolution of the different TGA parameters from baseline to week 12 and their comparison with the reference range are provided in and .
Figure 2. Spaghetti plot of individual data comparing the baseline endogenous thrombin potential (ETP), peak and mean velocity rate index (mVRI) and absolute changes from baseline versus week 12 (visit 4). Changes from baseline were assessed using ordinary one-way analysis of variance with a Dunnett’s multiple comparison test. Only differences versus placebo are reported. E4, estetrol.
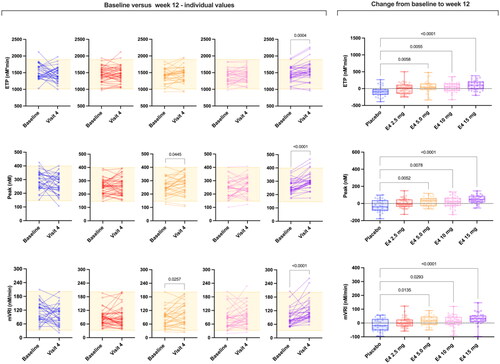
Figure 3. Spaghetti plot of individual data comparing baseline lag time and time to peak and absolute changes from baseline versus week 12 (visit 4). Changes from baseline were assessed using ordinary one-way analysis of variance with a Dunnett’s multiple comparison test. Only differences versus placebo are reported. E4, estetrol.
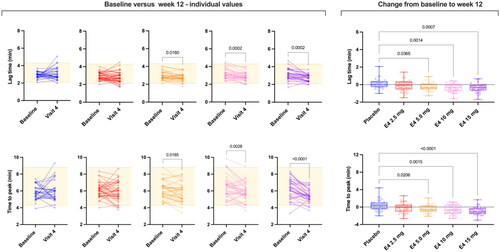
Table 2. Thrombin generation parameters with different doses of estetrol (E4) (from 2.5 mg to 15 mg once daily) at baseline and after 12 weeks of treatment.
presents the number of individual values outside the reference range after 12 weeks of treatment. More than 85% of the values were within the reference ranges, independent of the treatment group.
Table 3. Reference intervals (2.5th–97.5th percentile of entire baseline cohort) of thrombin generation parameters and out-of-range results after 12 weeks of treatment.
Changes from baseline
Results after 12 weeks of treatment were statistically different from baseline for all TGA parameters for E4 15 mg, for the lag time and time to peak for E4 10 mg and for all parameters except ETP for E4 5 mg, and no changes were observed for E4 2.5 mg and placebo ( and ). Changes remain mostly within the reference range ( and ). The absolute change from baseline is statistically significant for all TGA parameters. The multiple comparisons allowed to highlight that doses of 5 mg, 10 mg and 15 mg E4 showed statistically significant absolute change from baseline compared to placebo for all TGA parameters. The dose of 2.5 mg is not different from placebo.
Discussion
Hemostasis is a finely regulated physiological process and HT may induce changes in the levels of individual coagulation proteins which are not considered clinically relevant according to established normal ranges [Citation37,Citation38]. Nevertheless, it has been demonstrated that treatment with CEE or oral E2 may induce changes that, even if not considered clinically relevant when assessed individually (e.g. levels of prothrombin, FVII, FVIII, protein S), can be clinically relevant in global coagulation tests like the TGA [Citation5,Citation23]. Numerous publications reported an association between elevated TGA parameters and the risk of developing VTE [Citation19,Citation20,Citation39]. Therefore, as conventional oral HT increases thrombin generation [Citation5,Citation23,Citation40,Citation41], it is not surprising that this increase is also linked with the observed increased risk of thrombosis with oral HT containing CEE or E2 [Citation42]. On the other hand, it has been demonstrated that E2, once administered by a transdermal route, has a lower impact on the hemostatic system [Citation40,Citation43] and is not associated with an increased risk of thrombosis [Citation3].
In this study, we report for the first time TGA data with E4, the first NEST. The highest dose tested in this study, that is, E4 15 mg once daily, was shown to be effective for the relief of vasomotor symptoms [Citation32]. Interestingly, we demonstrated that E4 does not clinically impact thrombin generation (). Importantly, the mean thrombograms remain within the normal range established according to CLSI standards () [Citation37]. At the individual level, the impact of E4 on thrombin generation is very limited since only very few subjects have abnormal TGA parameters after 12 weeks of treatment compared to placebo (). Also, for some of these patients, the TGA results were already outside the reference range at baseline ( and ). The most influenced parameter is the peak but it is only translated into very few subjects outside the reference range (n = 4/32 for E4 15 mg versus n = 1/31 for placebo after 12 weeks of treatment; ). Importantly, the high thrombin generation curves observed in the placebo group at baseline undoubtedly influenced the change from baseline in this group. This finally also influenced the difference between the E4 groups and the placebo group when comparing the changes from baseline. This observation is confirmed by the fact that when comparing only the time point after 12 weeks of treatment, none of the E4 doses showed statistically different ETP, peak height, lag time or mVRI results compared to the placebo group after 12 weeks of treatment. It is important to note that the same dose of E4 has been used in combination with drospirenone in contraception and the results also revealed that this estrogen has a low impact on thrombin generation compared to ethinylestradiol combined with either levonorgestrel or drospirenone [Citation44].
A direct comparison of the results obtained in this study cannot be made with HT containing oral CEE or E2 as this study did not include other active comparator arms. Nevertheless, the change from baseline reported in other studies using similar thrombin generation methods suggests that the changes induced by E4 15 mg is not different from that of transdermal estrogen [Citation45]. Interestingly, this is in line with the data observed on APC resistance [Citation36]. Indeed, the median relative change from baseline in the normalized APC sensitivity ratio with E4 15 mg was 42% after 12 weeks of treatment, which is very close to the 28% observed by Post et al. with transdermal E2 at a dose of 50 µg [Citation43]. In any circumstances, the change from baseline is far lower than the ±100% normalized APC sensitivity ratio increase observed with the different oral preparations containing 17β-E2 as the estrogen [Citation43,Citation46,Citation47]. This clearly delineates that the different pharmacological profile of oral E4 compared to oral CEE or oral E2 results in a lower impact on the liver.
There is currently scientific evidence that explains the differences between oral E2 and oral E4. Orally administered E2 is already partly metabolized to estrone (E1) in the intestines [Citation48,Citation49]. The ileum produces more E1 after incubation with E2 than any other tissue except for the placenta [Citation50,Citation51]. E1 is less potent than E2 on nuclear and membrane ERα receptors, but once given orally it can accumulate in liver cells, the major site of coagulation protein synthesis [Citation52,Citation53]. Plasma levels of E1 have also been reported to be higher in users of oral E2 than in users of transdermal E2. In fact, the plasma levels of E1 and E2 are equal in transdermal E2 users while they are fivefold higher in oral E2 users [Citation54]. This also supports the possible link between E1 and increased impact on hemostasis with oral E2 [Citation5,Citation23]. The risk of a VTE event has been shown to correlate with E1 plasma levels. This risk has also been demonstrated to be 30-fold higher in women with the CYP3A5*1 genotype, encoding a high expression of the cytochrome P450 enzyme responsible for E1 formation from E2, thus implying that the formation of E1 and E1 sulfate is suggestive of an increased risk of VTE [Citation55–57]. This higher plasma E1 concentration has also been directly linked to higher thrombin generation potential [Citation23]. This evidence-based information suggests that the higher hepatic exposure to E1 that follows oral E2 administration, which is absent with transdermal HT, leads to increased thrombin generation with oral HT use [Citation23]. On the contrary, E4 is an end-stage product which means that it is not metabolized into active metabolites like E1, E2 or E3 [Citation29]. In addition, unlike E2, E4 is not converted into hydroxylated metabolites, precursors of quinone estrogens that can react and damage the DNA, which has been linked to breast cancer development [Citation58]. E4 is excreted in urine in the form of a D-ring monoglucuronide and is otherwise metabolically unaltered [Citation59]. Considering also the antagonism of E4 for the membrane-initiated steroid signaling pathway, which has been demonstrated to be an important regulator of lipid content in the liver [Citation60], there is a strong rationale to support the hypothesis that the weak effect of E4 on hemostasis is due to a combination of its specific pharmacodynamic and pharmacokinetic properties.
As estrogen response is highly tissue dependent, E4 represents the interesting combination of an estrogen with an efficient action on the uterus, bone and brain, which provides a rational for use for the relief of menopausal symptoms, while its limited effect on the liver and the breast may delineate a never-reached safety profile for an orally administered estrogen.
Conclusions
This study confirms the current evidence for the weak impact of E4 on hemostasis in postmenopausal women. After having demonstrated that E4 only minimally impacts APC resistance, the results presented in this manuscript reveal that its impact on thrombin generation, a global coagulation test sensitive to the changes induced by estrogenic compounds, are negligible and not clinically relevant. When comparing these results with the literature, it appears that oral E4 has a similar impact on thrombin generation to transdermal E2. Obviously, a direct comparison would be required to confirm this observation but the current knowledge about its pharmacological profile provide a rationale for this statement. More and more evidence is reinforcing the message that E4 has a neutral profile on hemostasis. Results from the phase 3 program will provide important additional information on the safety profile of E4 in menopause.
Potential conflict of interest
L.M and C.B. are employees of QUALIblood s.a. M.T. and M.J. are employees of Estetra SRL (an affiliate company of Mithra Pharmaceuticals). Y.O. and M.v.R were employees of Estetra SRL. U.G. is a senior consultant at Mithra Pharmaceuticals. J.-M.F. is a co-founder of Mithra Pharmaceuticals, a shareholder and a member of the Board. J.D. is CEO and founder of QUALIblood s.a. and reports personal fees and honorarium from Daiichi-Sankyo, Diagnostica Stago, DOASense, Gedeon Richter, Mithra Pharmaceuticals, Norgine, Portola, Roche and Roche Diagnostics.
Source of funding
The trial was funded by Estetra SRL (affiliate company of Mithra Pharmaceuticals); Walloon region in Belgium [convention N°7492].
Supplemental Material
Download MS Word (148.2 KB)Acknowledgements
The authors would like to thank the technical teams of QUALIblood s.a., the medical affairs team of Mithra Pharmaceuticals, the Walloon region in Belgium and the investigators in the participating centers for conducting the E4Relief trial [Citation17]. The authors also thank the members of the Donesta Scientific Advisory Boards for their valuable advice. Original data generated and analyzed during this study are included in this published article or in the data repositories listed in the references.
References
- Manson JE, Kaunitz AM. Menopause management–getting clinical care back on track. N Engl J Med. 2016;374(9):803–806. doi: 10.1056/NEJMp1514242.
- Vinogradova Y, Coupland C, Hippisley-Cox J. Use of hormone replacement therapy and risk of venous thromboembolism: nested case-control studies using the QResearch and CPRD databases. BMJ. 2019;364:k4810. doi: 10.1136/bmj.k4810.
- Rovinski D, Ramos RB, Fighera TM, et al. Risk of venous thromboembolism events in postmenopausal women using oral versus non-oral hormone therapy: a systematic review and meta-analysis. Thromb Res. 2018;168:83–95. doi: 10.1016/j.thromres.2018.06.014.
- Sundell M, Spetz Holm AC, Fredrikson M, et al. Pulmonary embolism in menopausal hormone therapy: a population-based register study. Climacteric. 2022;25(6):615–621. Dec doi: 10.1080/13697137.2022.2127352.
- Blondon M, van Hylckama Vlieg A, Wiggins KL, et al. Differential associations of oral estradiol and conjugated equine estrogen with hemostatic biomarkers. J Thromb Haemost. 2014;12(6):879–886. doi: 10.1111/jth.12560.
- Smith NL, Blondon M, Wiggins KL, et al. Lower risk of cardiovascular events in postmenopausal women taking oral estradiol compared with oral conjugated equine estrogens. JAMA Intern Med. 2014;174(1):25–31. doi: 10.1001/jamainternmed.2013.11074.
- Canonico M. Hormone therapy and risk of venous thromboembolism among postmenopausal women. Maturitas. 2015;82(3):304–307. doi: 10.1016/j.maturitas.2015.06.040.
- Harrington LB, Blondon M, Cushman M, et al. The cross-sectional association between vasomotor symptoms and hemostatic parameter levels in postmenopausal women. Menopause. 2017;24(4):360–370. doi: 10.1097/GME.0000000000000777.
- Kremers RM, Peters TC, Wagenvoord RJ, et al. The balance of pro- and anticoagulant processes underlying thrombin generation. J Thromb Haemost. 2015;13(3):437–447. doi: 10.1111/jth.12798.
- Brenner B. Haemostatic changes in pregnancy. Thromb Res. 2004;114(5–6):409–414. doi: 10.1016/j.thromres.2004.08.004.
- de Visser MC, van Hylckama Vlieg A, Tans G, et al. Determinants of the APTT- and ETP-based APC sensitivity tests. J Thromb Haemost. 2005;3(7):1488–1494. doi: 10.1111/j.1538-7836.2005.01430.x.
- Douxfils J, Morimont L, Bouvy C. Oral contraceptives and venous thromboembolism: focus on testing that may enable prediction and assessment of the risk. Semin Thromb Hemost. 2020;46(8):872–886. doi: 10.1055/s-0040-1714140.
- Morimont L, Haguet H, Dogné J-M, et al. Combined oral contraceptives and venous thromboembolism: review and perspective to mitigate the risk. Front Endocrinol. 2021;12:769187. doi: 10.3389/fendo.2021.769187.
- Canonico M, Alhenc-Gelas M, Plu-Bureau G, et al. Activated protein C resistance among postmenopausal women using transdermal estrogens: importance of progestogen. Menopause. 2010;17(6):1122–1127. doi: 10.1097/gme.0b013e3181e102eb.
- Eilertsen AL, Dahm AEA, Høibraaten E, et al. Relationship between sex hormone binding globulin and blood coagulation in women on postmenopausal hormone treatment. Blood Coagul Fibrinolysis. 2019;30(1):17–23. doi: 10.1097/MBC.0000000000000784.
- Morimont L, Dogné J-M, Douxfils J. Letter to the editors-in-Chief in response to the article of Abou-Ismail, et al. Entitled "Estrogen and thrombosis: a bench to bedside review" (thrombosis research 192 (2020) 40-51). Thrombosis Research. 2020;193:221–223. doi: 10.1016/j.thromres.2020.08.006.
- Hemker HC, Giesen P, Al Dieri R, et al. Calibrated automated thrombin generation measurement in clotting plasma. Pathophysiol Haemost Thromb. 2003;33(1):4–15. doi: 10.1159/000071636.
- Douxfils J, Morimont L, Delvigne AS, et al. Validation and standardization of the ETP-based activated protein C resistance test for the clinical investigation of steroid contraceptives in women: an unmet clinical and regulatory need. Clin Chem Lab Med. 2020;58(2):294–305. doi: 10.1515/cclm-2019-0471.
- van Hylckama Vlieg A, Christiansen SC, Luddington R, et al. Elevated endogenous thrombin potential is associated with an increased risk of a first deep venous thrombosis but not with the risk of recurrence. Br J Haematol. 2007;138(6):769–774. doi: 10.1111/j.1365-2141.2007.06738.x.
- Lutsey PL, Folsom AR, Heckbert SR, et al. Peak thrombin generation and subsequent venous thromboembolism: the longitudinal investigation of thromboembolism etiology (LITE) study. J Thromb Haemost. 2009;7(10):1639–1648. doi: 10.1111/j.1538-7836.2009.03561.x.
- Reda S, Morimont L, Douxfils J, et al. Can We measure the individual prothrombotic or prohemorrhagic tendency by global coagulation tests? Hamostaseologie. 2020;40(3):364–378. doi: 10.1055/a-1153-5824.
- Bucciarelli P, Mannucci PM. The hemostatic system through aging and menopause. Climacteric. 2009;12(Suppl 1):47–51. doi: 10.1080/13697130903006365.
- Bagot CN, Marsh MS, Whitehead M, et al. The effect of estrone on thrombin generation may explain the different thrombotic risk between oral and transdermal hormone replacement therapy. J Thromb Haemost. 2010;8(8):1736–1744. doi: 10.1111/j.1538-7836.2010.03953.x.
- Blondon M, Wiggins KL, Van Hylckama Vlieg A, et al. Smoking, postmenopausal hormone therapy and the risk of venous thrombosis: a population-based, case-control study. Br J Haematol. 2013;163(3):418–420. doi: 10.1111/bjh.12508.
- Bauer KA, Weiss LM, Sparrow D, et al. Aging-associated changes in indices of thrombin generation and protein C activation in humans. Normative aging study. J Clin Invest. 1987;80(6):1527–1534. doi: 10.1172/JCI113238.
- Abot A, Fontaine C, Buscato M, et al. The uterine and vascular actions of estetrol delineate a distinctive profile of estrogen receptor alpha modulation, uncoupling nuclear and membrane activation. EMBO Mol Med. 2014;6(10):1328–1346. doi: 10.15252/emmm.201404112.
- Arnal JF, Lenfant F, Metivier R, et al. Membrane and nuclear estrogen receptor alpha actions: from tissue specificity to medical implications. Physiol Rev. 2017;97(3):1045–1087. doi: 10.1152/physrev.00024.2016.
- Foidart JM, Gaspard U, Pequeux C, et al. Unique vascular benefits of estetrol, a native fetal estrogen with specific actions in tissues (NEST). In: Brinton RD, Genazzani AR, Simoncini T, et al. editors. Sex steroids’ effects on brain, heart and vessels. ISGE series. Cham: Springer International Publishing; 2019. p. 169–195.
- Gérard C, Arnal J-F, Jost M, et al. Profile of estetrol, a promising native estrogen for oral contraception and the relief of climacteric symptoms of menopause. Expert Rev Clin Pharmacol. 2022;15(2):121–137. doi: 10.1080/17512433.2022.2054413.
- Gaspard U, Taziaux M, Jost M, et al. A multicenter, randomized, placebo-controlled study to select the minimum effective dose of estetrol (E4) in postmenopausal participants (E4 relief): part 2. Vaginal cytology, genitourinary syndrome of menopause and health-related quality of life. Menopause. 2022;30(5):480–489. doi: 10.1097/GME.0000000000002167.
- Coelingh Bennink HJT, Verhoeven C, Zimmerman Y, et al. Pharmacodynamic effects of the fetal estrogen estetrol in postmenopausal women: results from a multiple-rising-dose study. Menopause. 2017;24(6):677–685. doi: 10.1097/GME.0000000000000823.
- Gaspard U, Taziaux M, Mawet M, et al. A multicenter, randomized study to select the minimum effective dose of estetrol (E4) in postmenopausal women (E4Relief): part 1. Vasomotor symptoms and overall safety. Menopause. 2020;27(8):848–857. Aug doi: 10.1097/GME.0000000000001561.
- Utian WH, Gaspard U, Jost M, et al. Estetrol, the next generation of hormone therapy: results of a phase 2b dose-finding study in postmenopausal women (E4 relief). San Diego, CA: NAMS; 2018.
- Douxfils J, Klipping C, Duijkers I, et al. Evaluation of the effect of a new oral contraceptive containing estetrol and drospirenone on hemostasis parameters. Contraception. 2020;102(6):396–402. Dec doi: 10.1016/j.contraception.2020.08.015.
- Douxfils J, Lobo R, Taziaux M, et al. Estetrol (E4) is a native fetal estrogen that does not modify coagulation markers in postmenopausal women and maintains sensitivity to activated protein C (APC). Maturitas. 2021;152:69. doi: 10.1016/j.maturitas.2021.08.013.
- Douxfils J, Gaspard U, Taziaux M, et al. Impact of estetrol (E4) on hemostasis, metabolism, and bone turnover in postmenopausal women. Climacteric. 2022;26(1):55–63. doi: 10.1080/13697137.2022.2139599.
- Clinical and Laboratory Standards Institute. Defining, establishing, and verifying reference intervals in the clinical laboratory; approved guideline, Third Edition. Wayne (PA): Clinical and Laboratory Standards Institute; 2010.
- Canonico M. Hormone therapy and hemostasis among postmenopausal women: a review. Menopause. 2014;21(7):753–762. doi: 10.1097/GME.0000000000000296.
- Tripodi A, Martinelli I, Chantarangkul V, et al. The endogenous thrombin potential and the risk of venous thromboembolism. Thromb Res. 2007;121(3):353–359. doi: 10.1016/j.thromres.2007.04.012.
- Oger E, Alhenc-Gelas M, Lacut K, et al. Differential effects of oral and transdermal estrogen/progesterone regimens on sensitivity to activated protein C among postmenopausal women: a randomized trial. Arterioscler Thromb Vasc Biol. 2003;23(9):1671–1676. doi: 10.1161/01.ATV.0000087141.05044.1F.
- Scarabin PY, Alhenc-Gelas M, Plu-Bureau G, et al. Effects of oral and transdermal estrogen/progesterone regimens on blood coagulation and fibrinolysis in postmenopausal women. A randomized controlled trial. Arterioscler Thromb Vasc Biol. 1997;17(11):3071–3078. doi: 10.1161/01.atv.17.11.3071.
- Canonico M, Plu-Bureau G, Lowe GD, et al. Hormone replacement therapy and risk of venous thromboembolism in postmenopausal women: systematic review and meta-analysis. BMJ. 2008;336(7655):1227–1231. doi: 10.1136/bmj.39555.441944.BE.
- Post MS, Christella M, Thomassen LG, et al. Effect of oral and transdermal estrogen replacement therapy on hemostatic variables associated with venous thrombosis: a randomized, placebo-controlled study in postmenopausal women. Arterioscler Thromb Vasc Biol. 2003;23(6):1116–1121. doi: 10.1161/01.ATV.0000074146.36646.C8.
- Morimont L, Jost M, Gaspard U, et al. Low thrombin generation in users of a contraceptive containing estetrol and drospirenone. J Clin Endocrinol Metab. 2022;108(1):135–143. doi: 10.1210/clinem/dgac511.
- Scarabin P-Y, Hemker HC, Clément C, et al. Increased thrombin generation among postmenopausal women using hormone therapy: importance of the route of estrogen administration and progestogens. Menopause. 2011;18(8):873–879. doi: 10.1097/gme.0b013e31820eee88.
- Post MS, Rosing J, Van Der Mooren MJ, et al. Increased resistance to activated protein C after short-term oral hormone replacement therapy in healthy post-menopausal women. Br J Haematol. 2002;119(4):1017–1023. doi: 10.1046/j.1365-2141.2002.03957.x.
- Post MS, van der Mooren MJ, van Baal WM, et al. Effects of low-dose oral and transdermal estrogen replacement therapy on hemostatic factors in healthy postmenopausal women: a randomized placebo-controlled study. Am J Obstet Gynecol. 2003;189(5):1221–1227. doi: 10.1067/s0002-9378(03)00599-4.
- Mashchak CA, Lobo RA, Dozono-Takano R, et al. Comparison of pharmacodynamic properties of various estrogen formulations. Am J Obstet Gynecol. 1982;144(5):511–518. doi: 10.1016/0002-9378(82)90218-6.
- Stumpf PG. Pharmacokinetics of estrogen. Obstet Gynecol. 1990;75(Supplement):15S. discussion 15S-17S. doi: 10.1097/00006250-199004001-00003.
- Ryan KJ, Engel LL. The interconversion of estrone and estradiol by human tissue slices. Endocrinology. 1953;52(3):287–291. doi: 10.1210/endo-52-3-287.
- Tebbens M, Heijboer AC, T’Sjoen G, et al. The role of estrone in feminizing hormone treatment. J Clin Endocrinol Metab. 2022;107(2):e458–e466. doi: 10.1210/clinem/dgab741.
- Di Bitondo R, Hall AJ, Peake IR, et al. Oestrogenic repression of human coagulation factor VII expression mediated through an oestrogen response element sequence motif in the promoter region. Hum Mol Genet. 2002;11(7):723–731. doi: 10.1093/hmg/11.7.723.
- Oger E, Lacut K, Mercier B, et al. Estrogen receptor alpha polymorphism and venous thromboembolism in male and female: data from the EDITH study. Thromb Res. 2007;119(4):433–439. doi: 10.1016/j.thromres.2006.05.002.
- Kuhl H. Pharmacology of estrogens and progestogens: influence of different routes of administration. Climacteric. 2005;8(sup1):3–63. doi: 10.1080/13697130500148875.
- Canonico M, Bouaziz E, Carcaillon L, et al. Synergism between oral estrogen therapy and cytochrome P450 3A5*1 allele on the risk of venous thromboembolism among postmenopausal women. J Clin Endocrinol Metab. 2008;93(8):3082–3087. doi: 10.1210/jc.2008-0450.
- Slater CC, Hodis HN, Mack WJ, et al. Markedly elevated levels of estrone sulfate after long-term oral, but not transdermal, administration of estradiol in postmenopausal women. Menopause. 2001;8(3):200–203. doi: 10.1097/00042192-200105000-00009.
- De Lignieres B, Basdevant A, Thomas G, et al. Biological effects of estradiol-17 beta in postmenopausal women: oral versus percutaneous administration. J Clin Endocrinol Metab. 1986;62(3):536–541. doi: 10.1210/jcem-62-3-536.
- Santen RJ, Yue W, Wang JP. Estrogen metabolites and breast cancer. Steroids. 2015;99(Pt A):61–66. doi: 10.1016/j.steroids.2014.08.003.
- Jirku H, Kadner S, Levitz M. Pattern of estetrol conjugation in the human. Steroids. 1972;19(4):519–534. doi: 10.1016/s0039-128x(72)80019-9.
- Pedram A, Razandi M, O’Mahony F, et al. Estrogen reduces lipid content in the liver exclusively from membrane receptor signaling. Sci Signal. 2013;6(276):ra36. doi: 10.1126/scisignal.2004013.