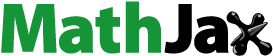
ABSTRACT
Fennel essential oil was encapsulated in β-cyclodextrin to prepare microcapsules (β-CD-FEO). Single-factor experiments and model fitting were used to optimise the microcapsules. When the optimal core/wall material ratio was 10:0.6, encapsulation time set as 90 min, and encapsulation temperature controlled at 60°C, an optimal encapsulation efficiency of 77.05 ± 0.14% can be obtained. Using β-CD-FEO blended with low acyl gellan gum (LA-GG) as main constituents, three-component coating solutions containing sodium alginate (SA), sodium carboxymethyl cellulose (CMC-Na), and konjac glucomannan (KGM) were investigated to evaluate their effect on the preservation of blueberries. Compared with the noncoated sample, coated blueberries showed excellent storage properties, especially KGM/LA-GG/β-CDs-FEO group had the highest sensory evaluation score under 25°C for 8 days. Therefore, KGM/LA-GG/β-CDs-FEO has potential application value in maintaining the quality of blueberries.
KEYWORDS:
Introduction
Blueberry (Vaccinium corymbosum L.) is often called ‘the king of berries’ owing to its attractive flavour and colour, delicate pulp, and high nutrient content, including anthocyanins, vitamins, flavonoids, and phenols, among other nutrients (Ji et al., Citation2021). Fresh blueberries are prone to deterioration via water and cell sap loss, ageing, old growth and spoilage, while their high water content increases the risk of storage-induced commercial value loss arising from microbial contamination and mechanical damage (Rokayya et al., Citation2021). In addition, the oxidation of the bioactive substances in blueberries may be induced by the postharvest storage conditions, thereby reducing their phenolic content and antioxidant capacity (Liu, Yi, et al., Citation2021). Many technologies have been employed to extend the shelf life of blueberries and prevent their deterioration during storage, such as refrigeration, modified atmosphere packaging, and ultraviolet irradiation. Hu, Liu, et al. (Citation2021) demonstrated that treatment with atmospheric cold plasma eliminates excessive microbes and fungi (e.g. Botrytis cinerea) and thereby prevents the natural decay of blueberries during postharvest storage. Additionally, appropriate radiation may modulate the activities of superoxide dismutase, catalase and peroxidase in blueberries, and thus reduce the superoxide (O2−•) and hydrogen peroxide contents, thereby reducing respiratory intensity and lipoxygenase activity (C. Wang et al., Citation2017)., Treating postharvest blueberries with melatonin is known to delay softening, prevent decay, inhibit weight loss, and enhance the expression of antioxidant genes and antioxidant capacity, further maintaining the quality of blueberries (Teng et al., Citation2020). Despite enabling effective preservation, the above technologies are expensive and cumbersome, which necessitates the further development of safe, efficient, economical, and environmentally friendly blueberry preservation methods.
Recently, the edible materials derived from plants and natural substances (e.g. proteins, polysaccharides, and lipids) have attracted significant attention (Liu, Ahmed, et al., Citation2021). These additives and polymers combined with each other to form different coatings, which can actively inhibit the growth of fungi, pathogens and human enteroviruses, and thereby extend shelf life and improve food safety (Luo et al., Citation2022). In particular, the polysaccharides extracted from algae (e.g. carrageenan, agar, and alginate) or crustaceans (e.g. chitin and chitosan) have been employed as biopolymers in edible films and coatings (Liu, Sameen, et al., Citation2021). Some approved food-grade additives, including natural extracts (e.g. green tea and grape seed), and essential oils have also been used in the fabrication of protective coatings. For example, fennel essential oil (FEO) is widely employed for its remarkable antifungal and antibacterial activities (Diao et al., Citation2014). X. Sun et al. (Citation2021) showed that a fennel oil-based nano-emulsion coating showed high antibacterial activity, and effectively inhibited the proliferation of Escherichia coli and Staphylococcus aureus. However, the direct use of plant essential oils to inhibit the growth of spoilage bacteria in food is limited by their water-insolubility, high volatility, and potential irritation (Y. X. Sun et al., Citation2021). These shortcomings can be overcome by microencapsulation, which relies on the use of natural or synthetic polymers to encapsulate the core active compounds in semi-permeable or sealed capsule membranes (Arabpoor et al., Citation2021). Microencapsulation can change the solubility and volatility of the core substances, control their release, prolong their duration of action and increase their stability (Liu et al., Citation2022). Microencapsulation can also separate active ingredients, reduce the direct contact between additives and food ingredients, and mask unpleasant tastes (Hu, Liu, et al., Citation2021). β-cyclodextrin (β-CD) is a cyclic oligosaccharide containing seven α-D-glucopyranosyl units connected by 1,4-glycosidic bonds (Deng et al., Citation2022) and features a hydrophilic surface with a hydrophobic cavity, and thus readily forms inclusion complexes with numerous guest compounds (R. Zhang et al., Citation2021). Especially, essential oils can be protected by encapsulation in β-CD, which also improves their water-solubility.
In this study, microcapsules with FEO and β-CD as the core and wall materials, respectively, were prepared by a particle gel method, and the effects of the microencapsulation parameters were analysed by single factor experiments and response surface methodology. The ability of three-component coating solutions containing sodium carboxymethyl cellulose (CMC-Na), sodium alginate (SA), or konjac glucomannan (KGM), combined with low-acyl gellan gum (LA-GG), and β-CD-FEO microcapsules to preserve blueberry freshness were investigated.
Materials and methods
Materials
Fresh blueberries were purchased from a blueberry orchard in Chengjiang City, Yunnan Province, China. FEO was purchased from Jiangxi Xinsen Natural Vegetable Oil Co., Ltd. (Jiangxi, China). SA (≥99%, M/G = 2:1, Food grade, Molecular Weight (Mw): 2,000–50,000 Da) and β-CD (98%) were obtained from Hubei Xinrunde Reagent Co., Ltd. (Wuhan, China). KGM, LA-GG, and CMC-Na were purchased from Shanghai Yuanye Biotechnology Co., Ltd. (Shanghai, China). Ultrapure water was obtained from a Milli-Q water system. All other chemicals and solvents were of analytical grade and purchased from Aladdin Biochemical Technology Co., Ltd. (Shanghai, China) unless stated otherwise.
Preparation of β-CD-FEO microcapsules
β-CD was dissolved in ultrapure water and stirred for 30, 60, 90, 120, or 150 min at 40, 50, 60, 70, or 80°C until a saturated solution was formed. Microcapsules with different core (FEO)/wall (β-CD) material ratios of 1:4, 1:5, 1:6, 1:7, or 1:8 w/w were synthesised. After stirring, the solution was cooled to 0–4°C, stood for 24 h, and vacuum filtered. The precipitate was washed three times with anhydrous ethanol and oven-dried at 50°C for 2 h to obtain β-CD-FEO microcapsules.
Response surface methodology
The effect of the ratio of core/wall materials, encapsulation temperature and encapsulation time on the encapsulation efficiency (EE) were evaluated. Single-factor experiments employed the Box-Behnken design to conduct three-factor and three-level experiments with EE as the response, and 17 experiments were performed at the central point. Every experiment was performed in triplicate.
Design Expert 8.0.6 software (Stat-Ease, Inc., Minneapolis, MN, USA) was used to implement the response surface method of experimental design and analysis, analyse EE data and generate an optimisation programme. A second-order polynomial model was established to associate the response variable with the independent variables:
where b0, bi, bii, and bij are the regression coefficients of the intercept, linear, quadratic, and interaction effects, respectively, and xi and xj are independent variables.
Preparation of coating solutions
SA, KGM, or CMC-Na was mixed with separate samples of LA-GG at a mass ratio of 4:1, and the mixture was dissolved in ultrapure water to an overall solid content of 0.2, 0.4, 0.6, 0.8, or 1.0 wt%. β-CD-FEO (0.05 wt%) and glycerine (0.01 wt%) were then added, and the dispersion was stirred until a clear solution was obtained (1–2 h) before being sonicated for 30 min at 100 W and 40°C. The resulting solution was sprayed on blueberries using a spray bottle at a distance of 10 cm to form a film on their surface.
Determination of EE
The EE was determined according to the method of Baiocco et al. (Citation2021). The FEO content of β-CD-FEO was determined using UV-vis spectroscopy. A calibration curve was obtained by dissolving FEO in absolute ethanol (0, 1, 3, 5, 7, and 9 μg/mL), and measuring the absorbance of these solutions at 280 nm. β-CD-FEO powder (20 mg) was added to absolute ethanol (50 mL), and the mixture was stirred for 45 mins before being centrifuged at 5000 rpm for 15 mins. The FEO content of the β-CD-FEO powder was calculated from the absorbance of the supernatant at 280 nm according to the standard curve, and EE was determined by EquationEquation (2)(2)
(2) :
Performance characterization
The surface morphology of the coatings was observed by scanning electron microscopy (SEM, Fei Quanta 200, the Netherlands) at an accelerating voltage of 10 kV. Prior to imaging, the samples were sputter-coated with Au under vacuum to increase conductivity. A contact angle analyser (OCA 15 PRO, Dataphysics Co., Germany) was used to measure surface hydrophobicity at 25°C. The sample was fixed on a movable plate and drops of ultrapure water (5 μL) were deposited on the sample surface. Images of these droplets were captured using a camera. Contact angles were measured at five different locations on each sample, and the mean was presented as the results. Fourier transform infrared spectra (FTIR, Nicolet iS1S0, Thermo Nicolet Inc., USA) were recorded at a resolution of 4 cm−1 in the wavelength range of 4000–500 cm−1. Thermal properties were analysed by differential scanning calorimetry (DSC, Q200 V24.2 Build 107, USA). The sample (~5 mg) was heated in an aluminium pan from 20 to 300°C at 10°C/min. X-ray diffraction patterns (XRD, MPX3, MAC Science, Japan) were recorded at a scan rate of 2°/min in the 2θ range of 5–70°. The rheological properties of the coating solutions were measured using a rheometer (AR 2000ex, TA Instrument Inc., New Castle, DE, USA). Each sample was tested at 25°C using a steady-state flow procedure between 0.1 and 100 s−1 (X. Sun et al., Citation2021).
Blueberry preservation experiment
Fresh blueberries with uniformity in size, shape, colour and physical integrity without visual defects were selected after harvest. They were divided into 125 g per plastic box, precooled at 10°C for 10 h, and then stored at 2°C with RH 90 ± 5% until loss of commodity value. Three of these groups were sprayed with one of SA/LA-GG/β-CD-FEO, KGM/LA-GG/β-CD-FEO, or CMC-Na/LA-GG/β-CD-FEO solutions, while the fourth was used as a blank (control) group. All samples were stored at 25°C for eight days. The preservation indices of each group were determined every 48 h. Each index was measured in triplicate and the average value was reported.
Weight loss
The blueberry weight loss was measured every two days and was calculated using EquationEquation (3)(3)
(3) :
where W0 is the initial weight of blueberries and Wt is the weight after storage for time t.
Firmness
To measure fruit firmness, seven fruit were randomly selected from each group, the equatorial epidermis was removed, and the flesh hardness of each fruit on the sunny side and the dark side was measured using a texture analyser (TMS-PRO, FTC, USA) with a 3 mm probe at a test speed of 1 mm s−1 and a trigger force of 0.375 N (Chu et al., Citation2018).
Determination of pH and titratable acidity
The pH value was measured using a portable pH meter/potentiometer (PHBJ-260, 0.01 pH resolution: Shanghai Precision & Scientific Instrument, Shanghai, China). The titratable acid (TA) was measured by an automatic titrator (ZD-2, Lei ci, Shanghai, China). The 10 mL blueberries juice was titrated at the final point (pH 8.3 ± 0.1) by 0.025 mol/L NaOH by an automatic titrator (ZD-2, Lei ci, Shanghai, China). EquationEq. (4)(4)
(4) was used to calculate the TA content (Emragi et al., Citation2022)
where the volume of NaOH was recorded as V1 (mL), the control volume V0 was set with 10 mL of distilled water instead of the sample (mL), c is the concentration of NaOH, v is the total volume of sample juice, Vs is the volume of titre using sample juice, 0.064 is the conversion factor of critic acid (g/mM) and m is the weight of the blueberries (g).
Determination of total soluble solid content
Total soluble solids (TSS) (%) in blueberries was determined using a digital refractometer (Reichert A2R200, Reichert GmbH, Seefeld, Germany) and expressed as °Brix. Values were expressed as mean ± standard deviation (SD, n = 30).
Determination of ascorbic acid (AA) content of blueberries
The AA content of blueberry juice was determined by titration with 2,6-dichlorophenolindophenol (DCPIP) (Xu et al., Citation2018). An aliquot (10 mL) of juice extracted from five fruits randomly picked from one group was diluted to 100 mL with an aqueous solution of oxalic acid (20 g/L). Subsequently, the supernatant (10 mL) was removed and titrated against a DCPIP solution (0.1 g/L) to a permanent pink colour. The AA content was calculated and expressed in mgAA per kgjuice.
Sensory evaluation
Sensory evaluation of the control group and three coating groups was performed based on the method of Ma et al (Ma et al., Citation2021). An untrained sensory panel of 50 judges assigned sensory scores for the changes in blueberry quality during storage. A 9-point scoring scale table was used to quantitatively assess sensory attributes such as taste, colour, odour, texture, and overall acceptability. The 9 points of the scoring scale are as follows. (9) like very much; (8) like very much; (7) like moderately; (6) like slightly; (5) neither like nor dislike; (4) dislike slightly; (3) dislike moderately; (2) dislike very much; and (1) dislike very much. Prior to evaluation, blueberries (approximately 10 g) were rinsed with water and placed in disposable white plastic trays bearing a random three-digit code. In addition, water was provided to rinse the mouth between the evaluation of different samples to neutralise the carryover effect of the aftertaste. All samples were prepared and randomly assigned to members of the same sensory panel. Evaluations were performed in a separate room in the sensory analysis laboratory.
Statistical analysis
All data were expressed as the mean ± standard deviation (SD). The one-way analysis of variance (ANOVA) and Duncan tests were performed in SPSS 22.0 to determine the significant differences between any two groups of data. A value of p < 0.05 was considered statistically significant.
Results and discussion
Single-factor experiments and model fitting
Extraction conditions were optimised using single factor experiments and response surface methodology, taking EE as an evaluation index. The factors and level of the Box-Behnken centre composite design were determined by evaluating the core: wall material ratio (%), encapsulation time (min), and encapsulation temperature (°C) as single factors ().
Table 1. Three-factor and three-level Box-Behnken design used in the response surface methodology and the experimental data related to survey response.
As the core wall material ratio increases, the EE initially increases, reaching a peak at a ratio of 65.2 ± 0.54%, before decreasing (). This behaviour is attributed to the existence of an optimal ratio. Ratios greater than or less than the optimal ratio cause a shortage of β-CD (resulting in unencapsulated FEO) and FEO (resulting in fewer microcapsules), respectively. The EE is relatively low at an encapsulation temperature of 40°C (); however, the EE increased with encapsulation temperature, reaching at peak at 60°C (71.37 ± 0.66%) before decreasing. This behaviour was ascribed to the fact that β-CD exhibits a maximum solubility at 60°C. The highest EE (72 ± 0.71%) was obtained with an encapsulation time of 90 mins. This behaviour is ascribed to the interplay between the effects of mixing, which both promotes FEO encapsulation and destroys the capsule shell, thereby leading to FEO outflow. Based on the results of single factor experiments, 17 new experiments were designed and implemented to optimise encapsulation conditions (), and the results were evaluated by regression and significance analysis (). The following correlation was established:
Figure 1. Influence of (a) Core wall material ratio, (b) Embedding temperature, and (c) Mixing time on EE.
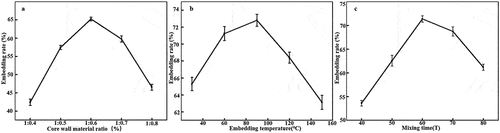
Table 2. The significance of the regression coefficients.
where X1, X2, and X3 are the core/wall material ratio, encapsulation time, and encapsulation temperature, respectively.
The influence of important variables was evaluated by linear and quadratic ANOVA (). High F-values (30.54) and low P-values (0.0001) were considered statistically significant. Moreover, the high significance (p < 0.01) and correlation coefficient R2 (0.9752) of the ANOVA regression model demonstrated that the generality and accuracy of the polynomial model sufficiently explained the changes in most dependent variables. P-values lower than 0.05 and 0.01 indicate statistical significance and high statistical significance, respectively. The encapsulation efficiency of the microcapsules was significantly affected by X2, X2X3, X12, X22, and X32. Three-dimensional response surfaces () illustrate the interactions between pairs of independent variables, and demonstrates the optimal values of these variables.
The interaction between X1 and X3 had a significant impact on the EE (), whereas the interaction between X3 and X2 was weak. Under constant X3, the EE initially increases with increasing X2, then decreases (), though the change was not statistically significant. Although the interaction between X1 and X2 was not obvious (), the former parameter significantly influenced the EE. Under constant X2, the EE changed significantly with X1. As a result, the optimal core/wall material ratio, encapsulation time, and encapsulation temperature were identified as 10:0.6, 90 min, and 60°C, respectively, giving an optimal EE of 77.05 ± 0.14%.
Morphology
The microstructure of the coating is closely related to its functional characteristics and matrix composition (W. Zhang et al., Citation2019). SEM images of the three coatings () show the existence of microporous and loose sponge structures in all cases (which may be formed by precipitation after freeze-drying) in all cases. The common point of the three coating fluids is uneven, showing a similar ‘pie’ shape with different sizes, while certain pores can be observed in the coating. Further comparison revealed that many aggregates were present on the surfaces of SA/LA-GG/β-CD-FEO (A-1, A-2) and CMC-Na/LA-GG/β-CD-FEO (B-1, B-2), which formed a large convex structure. The surface of KGM/LA-GG/β-CD-FEO (C-1, C-2) was smoother, more uniform and more compact, with no cracks, and superior interlayer overlap is better. Concurrently, a small number of spherical particles were detected owing to the insufficient mixing of FEO microcapsules in the coating solution, which could be evenly coated on fruits (Li et al., Citation2013).
Water contact angle
The water contact angle is positively correlated with the surface hydrophobicity (Du et al., Citation2021; Rhim & Wang, Citation2014). Contact angle measurements () show that the contact angle of KGM/LA-GG/β-CD-FEO (73.23–76.61°) was significantly higher than those of CMC-Na/LA-GG/β-CD-FEO (46.96–48.99°) and SA/LA-GG/β-CD-FEO (38.29–38.71°). The hydrophobicity of the coatings depends on the properties of the outer material (Souza et al., Citation2018). Among the three coating materials studied herein, KGM exhibited the highest hydrophobicity and, hence, the strongest ability to block water vapour and thereby preclude the loss of internal water and the entry of external water, which is crucial for fruit preservation.
FTIR spectra
The FTIR spectra of the samples () show a strong, broad peak at 3300–3400 cm−1 owing to the stretching vibration of O – H. The peak at 2925 cm−1 is attributed to the antisymmetric stretching vibration of −CH2− groups, while those at 1155 and 965 cm−1 are attributed to the C – O stretching of ether (C – O – C) groups in the cavity of β-CD and the corresponding in-plane rocking vibration, respectively (Huang et al., Citation2019). All the peaks are similar to those in the spectrum of pure β-CD; however, no peaks characteristic of FEO were observed, which is consistent with the encapsulation of FEO by β-CD. The spectra of the coatings containing β-CD-FEO were very similar to those of the microcapsules, as the added polysaccharide had a molecular weight < 20% of that of β-CD, while the spectrum showed bands coinciding with those of β-CD. This explanation is consistent with previous reports (J. Wang et al., Citation2011; Yuan et al., Citation2012). However, careful observation showed that, unlike β-CD-FEO, other spectra have a peak characteristic of LA-GG at 2925 cm−1, indicating that LA-GG shows good biocompatibility with both essential oil microcapsules and the other three materials, allowing β-CD-FEO to be uniformly dispersed and retained in the cavity structure.
DSC
The successful binding of guest molecules is usually reflected in their DSC spectra by the shift or disappearance of endothermic melting peaks (Chao Yuan et al., Citation2019). The DSC curves of β-CD-FEO and the three coatings () reveal two types of thermal events (volatilisation and decomposition) in the range of 50–250°C in all cases. The DSC curve of β-CD-FEO showed numerous thermal events attributed to the release of water (50–130°C) and an endothermic event at 150–250°C; however, no characteristic peaks other than those of β-CD were observed, demonstrating that FEO was successfully encapsulated by β-CD. Similar behaviour has been observed in the encapsulation of other bioactive compounds by β-CD (Pushpalatha et al., Citation2018). The DSC curves of the coatings is similar with that of β-CD-FEO; however, the coatings have higher minimum melting temperatures. The added polysaccharide may increase the melting temperature or provide additional free water, which consumes more heat for its removal and so requires a higher temperature. The characteristic FEO peak was not observed in the spectra of the coatings, which indicated that β-CD-FEO and the coating materials were well mixed, forming a uniform and highly stable coating.
XRD
shows the XRD patterns of β-CD, β-CD-FEO and the three coatings. The XRD pattern of β-CD-FEO is similar to that of β-CD; however, the intensities of the individual peaks differ slightly: the peak intensity of β-CD-FEO is reduced because the incorporated FEO molecules are separated from each other and cannot form crystalline aggregates (Celebioglu & Uyar, Citation2020). The XRD patterns of the three composite coatings showed similar characteristic peaks, with two weak peaks at 18.2° and 33.8° and one broad peak at 22.3°, which were attributed to LA-GG (X. R. Zhang et al., Citation2021): however, none of the spectra of any of the three coatings exhibit any peaks characteristic of β-CD-FEO, indicating that the microcapsules were well dispersed in the three coating solutions, resulting in a stable and homogeneous coating. Moreover, the added FEO microcapsules were compatible with LA-GG, SA, KGM and CMC-Na, and thus had no effect on the crystalline properties of the coating.
Rheological properties
The apparent viscosity of coating solutions decreased with increasing shear (), indicating that all the solutions are pseudoplastic fluids that exhibited shear thinning behaviour. The behaviour of these solutions can therefore be described by the power law model, which is typically used to calculate the flow behaviour index and consistency coefficient of food emulsions (Silva et al., Citation2015). The reduction in viscosity may be explained by the deconstruction of polymer chain entanglement induced by the high shear rate and change of particle flow direction, thereby leading to the destruction of the gel network (Xue et al., Citation2019). The consistency coefficient, which is positively correlated with the intermolecular forces of the emulsion, decreased in the order of CMC-Na/LA-GG/β-CD-FEO > SA/LA-GG/β-CD-FEO > KGM/LA-GG/β-CD-FEO.
Blueberry preservation performance
Weight loss
Blueberries typically lose their nutrients and surface water during storage owing to their strong postharvest respiration, which leads to rapid quality deterioration. Aerobic respiration of blueberry transforms some organic substances into CO2 and H2O (Niu et al., Citation2019). The weight loss in each group of blueberries increases with the storage time (). No obvious weight loss or difference was observed between the two groups during the first 2 days of storage (p < 0.05). However, from 4th day of storage, significant differences in the weight loss of the sample groups were observed, particularly in the control group. By 8th day, a weight loss of up to 34% was observed. The observed weight loss in the three groups of coated blueberries was significantly lower than that in the control group owing to the formation of surface coatings which hindered gas exchange and respiration and thereby maintained the freshness of the blueberries (Qureshi et al., Citation2020). The KGM/LA-GG/β-CD-FEO group exhibited the lowest weight loss and this may be ascribed to the ability of the strongly hydrophobic KGM to prevent the dispersion and loss of internal water. These factors may hinder the penetration of external water, keep the dry surface of the berries, and thus inhibit the growth of spoilage bacteria.
Hardness
The firmness of blueberry fruits decreases with time during low-temperature storage (). In the late storage period, the degree of softening in the control group was significantly higher than those of coated groups. On the 1st day of storage, the hardness of the control group was slightly higher than those of the coating groups owing to the harmful influence of the acid coating solution on hardness. However, from the 2nd day, the hardness of coated blueberries decreased more slowly than that of the control group. There are two main reasons affecting the changes of hardness. On the one hand, the coatings may act as a barrier to inhibit the transpiration of blueberries. This can influence the activities of enzymes related to the degradation of pectin, hemicellulose and cellulose, retain cell wall structural polysaccharides, slow down the fruit softening, and maintain the texture and hardness of the pulp on postharvest blueberries.
On the other hand, with the extension of storage time, the water generated by fruit respiration will degrade the coating solution, which in turn leads to the release of FEO from the microcapsules into the coating. FEO will present on the surface of the fruit by diffusion, it could cause severe disintegration of the cell membrane and organelles of the pathogenic fungus at the cellular level and suppress the incidence of postharvest diseases of blueberries, further extend softening during fruit maturation, leading to cell wall loosening and disintegration. Among the three coating groups, the KGM/LA-GG/β-CD-FEO group exhibited the smallest reduction in hardness owing to the high hydrophobicity of KGM, which kept the surface of fruit sufficiently dry to prevent microbial decomposition, effectively inhibiting the metabolic activity related to respiration and thus maintaining fruit quality (Nair et al., Citation2020).
pH
The pH value of the blueberries in all groups increased with storage time, which was attributed to metabolic processes during storage and the gradual conversion of starch and acids to sugar () (Teng et al., Citation2020), which is in agreement with the known impact of fruit storage on nutritional status. The maturity of fruit is initially low, and the accumulation of internal nutrients leads to an increase in acid content. As the maturity increases, some acids are converted into sugars and other substances, thereby increasing pH value. In addition, the decomposition of amino acids leads to the accumulation of ammonia and amines, which further increases pH. The observed change in the pH value of each group differed significantly. On day 0, no significant differences in the pH of the control group and coating groups were observed (p < 0.05). From the next day, the pH value of the control group was significantly higher than those of the coating groups owing to the above-mentioned effects of protective coatings.
Titratable acidity (TA)
The TA of all groups decreases with the passage of time (). Because acids are used as respiratory substrates, the loss of acidity is accompanied by ripening, which is most significant in the control group. This behaviour is attributed to the antibacterial and antifungal effects of FEO (Y. X. Sun et al., Citation2021) and the ability of the coating to prevent outside air from entering, maintain a low-oxygen environment, and inhibit respiration (the main reason for the reduction in TA) (Cao et al., Citation2017). KGM/LA-GG/β-CD-FEO exhibited the best performance among the three coatings owing to its high hydrophobicity, insulation properties, and other advantages confirmed by previous hardness and pH analyses. All three coating solutions featured good film-forming properties, forming an effective barrier that inhibited respiration, reduced the extent of microbial damage, and inhibited the reduction in TA.
Total soluble solid (TSS) content
The TSS content of the samples is mainly composed of soluble sugars and organic acids, and its composition reflects the maturity, flavour and physiological changes of the blueberries. In each of the four groups, the TSS content initially increases and then decreases over time (). This behaviour was ascribed to the interplay between the increase in the content of sugars and other soluble species during postharvest ripening and the corresponding reduction of these compounds owing to respiration (Gholami et al., Citation2017). The changes in the TSS content of the control group was the most apparent. In this case, the maximum (20.03%) and minimum values (15.99%) were observed on the 4th and 8th days, respectively. This behaviour is attributed to the fact that the berries in the control group showed higher respiratory intensity because they were not protected by any coating. In contrast, the coatings inhibit the ripening of blueberries and prolong their shelf life by slowing down their respiration (Almajano et al., Citation2008). The quality and value of the blueberries are maximised when they are not picked at maturity. Therefore, the harvested blueberries will undergo post-ripening owing to the changes in the TSS content.
Ascorbic acid (AA)content
The AA content of all groups decreased over time (), with the reduction in that of the control group being the most significant. In this case, the minimum value of 13.21% was observed on the eighth day of storage. During the entire storage period, no significant difference in the AA content between SA/LA-GG/β-CD-FEO and CMC-Na/LA-GG/β-CD-FEO groups was observed (p < 0.05). The AA contents of these two groups were higher than that of the control group but significantly lower than that of the KGM/LA-GG/β-CD-FEO group. The super-hydrophobicity of KGM effectively prevents outside air from entering the blueberries, thereby limiting the oxygen concentration of the fruits and inhibiting their gas exchange rate, thereby inhibiting respiration, reducing the consumption of AA, and retaining the nutritional value of the blueberries.
Appearance
The coated blueberries retained their appearance better than those in the control group (). Blueberries in the control group showed clear signs of water loss and shrinkage by the fourth day of storage. At the end of the storage period, decay was observed in all groups; however, this was most pronounced in the control group. The KGM/LA-GG/β-CD-FEO group exhibited the good preservation effect, in agreement with previous performance index measurements.
Sensory assessment
shows the sensory evaluation scores of the blueberries. Initially, these scores indicated only a slight difference in the flavour and appearance of the control and treated samples, with the treated group being slightly lower than the control owing to the fragility of the blueberry skin, which was slightly damaged during the coating procedure. The sensory evaluation scores decreased with increasing storage time. By the eighth day of storage, the control group had completely lost its edibility, having a reduced score of 3, while the samples of the treated group were still edible, with a score of more than 5. The KGM/LA-GG/β-CDs-FEO group had the highest sensory evaluation score. The sensory evaluation scores showed that none of our three coating solutions produced any adverse odour in the blueberries.
Conclusion
Box-Behnken experimental design was used to evaluate the encapsulation efficiency of synthesised microcapsules of β-CD-enclosed FEO. The optimal encapsulation conditions were determined with a core/wall material ratio, encapsulation time, and temperature of 1:0.6, 90 min, and 60°C, respectively, affording a maximum encapsulation efficiency of 77.05 ± 0.14%. Three types of β-CD-FEO-loaded composite coating solutions were prepared, which provided uniform and compact films with good biocompatibility. These three adhesive coatings have good film-forming properties and form efficient gas and moisture barrier. Compared with the control (noncoated) sample, coated blueberries showed excellent storage properties in terms of weight loss, hardness, pH, TSS content, TA and AA content, particularly in the case of the KGM/LA-GG/β-CD-FEO coating.
Credit author statement
Xuedong Gu: Conceptualisation, Methodology, Investigation, Writing – original draft. Juan Li: Conceptualisation, Writing – review & editing. Lin Yang: Methodology, Investigation. Lei Liu: Investigation, Writing – review & editing. Tong Li: Writing – review & editing. Hailin Zhang: Writing – review & editing. Yu Gao: Writing – review & editing. Lan Xiao: Funding acquisition, Project administration.
Disclosure statement
No potential conflict of interest was reported by the author(s).
Additional information
Funding
References
- Almajano, M. P., Carbó, R., Jiménez, J. A. L., & Gordon, M. H. (2008). Antioxidant and antimicrobial activities of tea infusions. Food Chemistry, 108(1), 55–63. https://doi.org/10.1016/j.foodchem.2007.10.040
- Arabpoor, B., Yousefi, S., Weisany, W., & Ghasemlou, M. (2021). Multifunctional coating composed of eryngium campestre L. essential oil encapsulated in nano-chitosan to prolong the shelf-life of fresh cherry fruits. Food Hydrocolloids, 111, 106394. https://doi.org/10.1016/j.foodhyd.2020.106394
- Baiocco, D., Preece, J. A., & Zhang, Z. (2021). Microcapsules with a fungal chitosan-gum Arabic-maltodextrin shell to encapsulate health-beneficial peppermint oil. Food Hydrocolloids for Health, 1, 100016. https://doi.org/10.1016/j.fhfh.2021.100016
- Cao, X., Huang, R., & Chen, H. (2017). Evaluation of pulsed light treatments on inactivation of Salmonella on blueberries and its impact on shelf-life and quality attributes. International Journal of Food Microbiology, 260, 17–26. https://doi.org/10.1016/j.ijfoodmicro.2017.08.012
- Celebioglu, A., & Uyar, T. (2020). Development of ferulic acid/cyclodextrin inclusion complex nanofibers for fast-dissolving drug delivery system. International Journal of Pharmacology, 584, 119395. https://doi.org/10.1016/j.ijpharm.2020.119395
- Chu, W., Gao, H., Chen, H., Fang, X., & Zheng, Y. (2018). Effects of cuticular wax on the postharvest quality of blueberry fruit. Food Chemistry, 239, 68–74. https://doi.org/10.1016/j.foodchem.2017.06.024
- Deng, C., Cao, C., Zhang, Y., Hu, J., Gong, Y., Zheng, M., & Zhou, Y. (2022). Formation and stabilization mechanism of β-cyclodextrin inclusion complex with C10 aroma molecules. Food Hydrocolloids, 123, 107013. https://doi.org/10.1016/j.foodhyd.2021.107013
- Diao, W. R., Hu, Q. P., Zhang, H., & Xu, J. G. (2014). Chemical composition, antibacterial activity and mechanism of action of essential oil from seeds of fennel (foeniculum vulgare Mill.). Food Control, 35(1), 109–116. https://doi.org/10.1016/j.foodcont.2013.06.056
- Du, Y., Yang, F., Yu, H., Cheng, Y., Guo, Y., Yao, W., & Xie, Y. (2021). Fabrication of novel self-healing edible coating for fruits preservation and its performance maintenance mechanism. Food Chemisty, 351, 129284. https://doi.org/10.1016/j.foodchem.2021.129284
- Emragi, E., Kalita, D., & Jayanty, S. S. (2022). Effect of edible coating on physical and chemical properties of potato tubers under different storage conditions. LWT, 153, 112580–112581. https://doi.org/10.1016/j.lwt.2021.112580
- Gholami, R., Ahmadi, E., & Farris, S. (2017). Shelf life extension of white mushrooms (Agaricus bisporus) by low temperatures conditioning, modified atmosphere, and nanocomposite packaging material. Food Packaging and Shelf Life, 14, 88–95. https://doi.org/10.1016/j.fpsl.2017.09.001
- Huang, H., Belwal, T., Aalim, H., Li, L., Lin, X., Liu, S., Ma, C., Li, Q., Zou, Y., & Luo, Z. (2019). Protein-polysaccharide complex coated W/O/W emulsion as secondary microcapsule for hydrophilic arbutin and hydrophobic coumaric acid. Food Chemistry, 300, 125171. https://doi.org/10.1016/j.foodchem.2019.125171
- Hu, X., Liu, C., Zhang, H., Hossen, M. A., Sameen, D. E., Dai, J., Qin, W., Liu, Y., & Li, S. (2021). In vitro digestion of sodium alginate/pectin co-encapsulated lactobacillus bulgaricus and its application in yogurt bilayer beads. International Journal of Biological Macromolecules, 193, 1050–1058. https://doi.org/10.1016/j.ijbiomac.2021.11.076
- Ji, Y., Hu, W., Liao, J., Xiu, Z., Jiang, A., Guan, Y., Yang, X., & Feng, K. (2021). Ethanol vapor delays softening of postharvest blueberry by retarding cell wall degradation during cold storage and shelf life. Postharvest Biology and Technology, 177, 111538. https://doi.org/10.1016/j.postharvbio.2021.111538
- Li, F., Biagioni, P., Finazzi, M., Tavazzi, S., & Piergiovanni, L. (2013). Tunable green oxygen barrier through layer-by-layer self-assembly of chitosan and cellulose nanocrystals. Carbohydrate Polymers, 92(2), 2128–2134. https://doi.org/10.1016/j.carbpol.2012.11.091
- Liu, Y., Ahmed, S., Sameen, D. E., Wang, Y., Lu, R., Dai, J., Li, S., & Qin, W. (2021). A review of cellulose and its derivatives in biopolymer-based for food packaging application. Trends in Food Science & Technology, 112, 532–546. https://doi.org/10.1016/j.tifs.2021.04.016
- Liu, Y., Ma, Y., Liu, Y., Zhang, J., Hossen, M. A., Sameen, D. E., Dai, J., Li, S., & Qin, W. (2022). Fabrication and characterization of pH-responsive intelligent films based on carboxymethyl cellulose and gelatin/curcumin/chitosan hybrid microcapsules for pork quality monitoring. Food Hydrocolloids, 124, 107224. https://doi.org/10.1016/j.foodhyd.2021.107224
- Liu, Y., Sameen, D. E., Ahmed, S., Wang, Y., Lu, R., Dai, J., Li, S., & Qin, W. (2021). Antimicrobial peptides and their application in food packaging. Trends in Food Science & Technology, 112, 471–483. https://doi.org/10.1016/j.tifs.2021.04.019
- Liu, Y., Yi, S., Sameen, D. E., Hossen, M. A., Dai, J., Li, S., Qin, W., & Lee, K. J. (2021). Designing and utilizing 3D printed chitosan/halloysite nanotube/tea polyphenol composites to maintain the quality of fresh blueberries. Innovative Food Science & Emerging Technologies, 74, 102808. https://doi.org/10.1016/j.ifset.2021.102808
- Luo, Q., Hossen, M. A., Zeng, Y., Dai, J., Li, S., Qin, W., & Liu, Y. (2022). Gelatin-based composite films and their application in food packaging: A review. Journal of Food Engineering, 313, 110762. https://doi.org/10.1016/j.jfoodeng.2021.110762
- Ma, Y., Zhao, Y., Xie, J., Sameen, D. E., Ahmed, S., Dai, J., Qin, W., Li, S., & Liu, Y. (2021). Optimization, characterization and evaluation of papaya polysaccharide-corn starch film for fresh cut apples. International Journal of Biological Macromolecules, 166, 1057–1071. https://doi.org/10.1016/j.ijbiomac.2020.10.261
- Nair, M. S., Tomar, M., Punia, S., Kukula-Koch, W., & Kumar, M. (2020). Enhancing the functionality of chitosan and alginate-based active edible coatings/films for the preservation of fruits and vegetables: A review. International Journal of Biological Macromolecules, 164, 304–320. https://doi.org/10.1016/j.ijbiomac.2020.07.083
- Niu, B., Shao, P., Chen, H., & Sun, P. (2019). Structural and physiochemical characterization of novel hydrophobic packaging films based on pullulan derivatives for fruits preservation. Carbohydrate Polymers, 208, 276–284. https://doi.org/10.1016/j.carbpol.2018.12.070
- Pushpalatha, R., Selvamuthukumar, S., & Kilimozhi, D. (2018). Cross-linked, cyclodextrin-based nanosponges for curcumin delivery - physicochemical characterization, drug release, stability and cytotoxicity. Journal of Drug Delivery Science and Technology, 45, 45–53. https://doi.org/10.1016/j.jddst.2018.03.004
- Qureshi, M. A., Nishat, N., Jadoun, S., & Ansari, M. Z. (2020). Polysaccharide based superabsorbent hydrogels and their methods of synthesis: A review. Carbohydrate Polymer Technologies and Applications, 1, 100014. https://doi.org/10.1016/j.carpta.2020.100014
- Rhim, J. W., & Wang, L. F. (2014). Preparation and characterization of carrageenan-based nanocomposite films reinforced with clay mineral and silver nanoparticles. Applied Clay Science, 97-98, 174–181. https://doi.org/10.1016/j.clay.2014.05.025
- Rokayya, S., Jia, F., Li, Y., Nie, X., Xu, J., Han, R., Yu, H., Amanullah, S., Almatrafi, M.M., & Helal, M. (2021). Application of nano-titanum dioxide coating on fresh highbush blueberries shelf life stored under ambient temperature. LWT, 137, 110422. https://doi.org/10.1016/j.lwt.2020.110422
- Silva, E. K., Gomes, M. T. M. S., Hubinger, M. D., Cunha, R. L., & Meireles, M. A. A. (2015). Ultrasound-assisted formation of annatto seed oil emulsions stabilized by biopolymers. Food Hydrocolloids, 47, 1–13. https://doi.org/10.1016/j.foodhyd.2015.01.001
- Souza, M. P., Vaz, A. F. M., Costa, T. B., Cerqueira, M. A., De Castro, C. M. M. B., Vicente, A. A., & Carneiro-da-Cunha, M. G. (2018). Construction of a biocompatible and antioxidant multilayer coating by layer-by-layer assembly of κ-carrageenan and quercetin nanoparticles. Food and Bioprocess Technology, 11(5), 1050–1060. https://doi.org/10.1007/s11947-018-2077-6
- Sun, X., Wu, Q., Picha, D. H., Ferguson, M. H., Ndukwe, I. E., & Azadi, P. (2021). Comparative performance of bio-based coatings formulated with cellulose, chitin, and chitosan nanomaterials suitable for fruit preservation. Carbohydrate Polymers, 259, 117764. https://doi.org/10.1016/j.carbpol.2021.117764
- Teng, X., Zhang, M., Devahastin, S., & Yu, D. (2020). Establishment of lower hygroscopicity and adhesion strategy for infrared-freeze-dried blueberries based on pretreatments using CO2 laser in combination with ultrasound. Food and Bioprocess Technology, 13(12), 2043–2053. https://doi.org/10.1007/s11947-020-02543-5
- Wang, J., Cao, Y., Sun, B., & Wang, C. (2011). Physicochemical and release characterisation of garlic oil-β-cyclodextrin inclusion complexes. Food Chemistry, 127(4), 1680–1685. https://doi.org/10.1016/j.foodchem.2011.02.036
- Wang, C., Gao, Y., Tao, Y., Wu, X., & Zhibo, C. (2017). Influence of γ-irradiation on the reactive-oxygen metabolism of blueberry fruit during cold storage. Innovative Food Science & Emerging Technologies, 41, 397–403. https://doi.org/10.1016/j.ifset.2017.04.007
- Xue, F., Gu, Y., Wang, Y., Li, C., & Adhikari, B. (2019). Encapsulation of essential oil in emulsion based edible films prepared by soy protein isolate-gum acacia conjugates. Food Hydrocolloids, 96, 178–189. https://doi.org/10.1016/j.foodhyd.2019.05.014
- Xu, D., Qin, H., & Ren, D. (2018). Prolonged preservation of tangerine fruits using chitosan/montmorillonite composite coating. Postharvest Biology and Technology, 143, 50–57. https://doi.org/10.1016/j.postharvbio.2018.04.013
- Yuan, C., Jin, Z., & Xu, X. (2012). Inclusion complex of astaxanthin with hydroxypropyl-beta-cyclodextrin: UV, FTIR, 1H NMR and molecular modeling studies. Carbohydrate Polymers, 89(2), 492–496. https://doi.org/10.1016/j.carbpol.2012.03.033
- Yuan, C., Wang, Y., Liu, Y., & Cui, B. (2019). Physicochemical characterization and antibacterial activity assessment of lavender essential oil encapsulated in hydroxypropyl-beta-cyclodextrin. Industrial Crops and Products, 130, 104–110. https://doi.org/10.1016/j.indcrop.2018.12.067
- Zhang, R., Ma, Y., Lan, W., Sameen, D. E., Ahmed, S., Dai, J., Qin, W., Li, S., & Liu, Y. (2021). Enhanced photocatalytic degradation of organic dyes by ultrasonic-assisted electrospray TiO2/graphene oxide on polyacrylonitrile/β-cyclodextrin nanofibrous membranes. Ultrasonics Sonochemistry, 70, 105343. https://doi.org/10.1016/j.ultsonch.2020.105343
- Zhang, W., Shu, C., Chen, Q., Cao, J., & Jiang, W. (2019). The multi-layer film system improved the release and retention properties of cinnamon essential oil and its application as coating in inhibition to penicillium expansion of apple fruit. Food Chemistry, 299, 125109. https://doi.org/10.1016/j.foodchem.2019.125109