ABSTRACT
Introduction
Despite huge efforts, tuberculosis (TB) is still a major public health threat worldwide, it is estimated that a quarter of the global population is infected by Mycobacterium tuberculosis (Mtb). For controlling TB and reducing Mtb transmission it is fundamental to diagnose TB infection (TBI) as well as the progressors from TBI to disease to identify those requiring preventive therapy. At present, there is no gold standard test for TBI diagnosis although several new methodologies have been attempted.
Areas covered
This review provides an update on the most recent approaches to develop reliable tests to diagnose TBI and progressors from infection to disease. Experimental tests are based on either the direct identification of Mtb (i.e., Mtb DNA upon host cells isolation; Mtb proteins or peptides) or host response (i.e., levels and quality of specific anti-Mtb antibodies; host blood transcriptome signatures).
Expert opinion
The experimental tests described are very interesting. However, further investigation and randomized clinical trials are needed to improve the sensitivity and specificity of these new research-based tests. More reliable proofs-of-concept and simplification of technical procedures are necessary to develop new diagnostic tools for identifying TBI patients and those that will progress from infection to TB disease.
1. Introduction
Tuberculosis (TB) is an infectious disease caused by Mycobacterium tuberculosis (Mtb) and represents the major cause of ill health and one of the main leading causes of death worldwide [Citation1]. The World Health Organization (WHO) estimated that in 2021 almost 10 million people were affected by TB and about 1.6 million people died from the disease [Citation2].
TB is an airborne-transmitted disease through the inhalation of infectious droplet nuclei released by contagious individuals [Citation1,Citation3]. After exposure to Mtb, an estimated 20–25% of people get infected; among them 5–10% can develop TB disease in their life span and half of this risk is within the first 2 years from infection [Citation2]. Almost the 90% of the infected individuals control the replication of the pathogen through innate and adaptive immune responses leading to a latent state, which was traditionally denominated LTBI (Latent TB Infection) [Citation4]. This term indicates a state of persistent immune response to stimulation by Mtb antigens without clinically and/or microbiological manifestations of TB disease. Recently, the WHO adopted the term TB infection (TBI) to better indicate the process that starts with the Mtb infection and may lead to TB disease [Citation5].
TB disease is the clinical manifestation of bacterial replication that leads to tissue damage and inflammation. TB disease mainly involves lungs but may be localized in any tissue and organ system, displaying diverse manifestations depending on the specific site [Citation6].
The spectrum of TB is determined by a dynamic equilibrium between the host immune system and Mtb, therefore besides the state of disease and infection there are two additional intermediate clinical stages: incipient TB and subclinical TB. Incipient TB is characterized by bacteria replication in the involved tissues and partial failure of the host immune response with no clinical, radiological, and microbiological signs of the disease; it is thought that this stage may progress to TB disease if not managed by therapy. Subclinical TB is characterized by the absence of symptoms, although there is an increase in Mtb replication detectable by microbiologic assays and in tissue damage detected by radiological images [Citation4,Citation7]. Higher risk for TB progression has been reported for people with an impaired immune response, such as individuals with HIV infection, diabetes [Citation8], immunosuppressive therapy [Citation9–11], or people co-infected with severe acute respiratory syndrome coronavirus 2 (SARS-CoV-2) [Citation12–15].
There are not yet standardized diagnostic tools to identify the subjects with incipient TB. An accurate diagnosis of TB infection is crucial as a tool to prevent disease development providing preventive therapy, which is an important component for global TB control [Citation5,Citation16], being 1.7 billion the estimated individuals with TBI [Citation2]. In this review, we report the research tests for TBI diagnosis, and we will focus on new emerging technologies.
2. Routine tests and their new developments
TBI is a state in which we detect an immune response to Mtb in the absence of clinical, microbiological and radiological signs of disease. Mtb is likely in a dormant or low-replicative stage and in the absence of standardized technologies to detect these bacilli, we measure the Mtb-specific immune response as an indirect assessment of infection using the tuberculin skin test (TST) and the interferon (IFN)-γ release assays (IGRA). No gold standard for the diagnosis of TBI are yet available.
TST is based on the intradermal injection of purified protein derivative (PPD), which induces a delayed-type hypersensitivity reaction; TST response is then evaluated by measuring the diameter of induration 48–72 h after PPD injection [Citation17]. Since PPD is a mixture of antigens, many of which are shared by Mtb, M. bovis, bacille Calmette – Guérin (BCG) and several species of environmental mycobacteria, TST has suboptimal specificity to detect Mtb infection [Citation18,Citation19]. Moreover, it is characterized by a low sensitivity in the immunocompromised individuals [Citation20].
To improve TST accuracy, new versions of skin tests have been proposed. They are based on the intradermal injections of the Mtb-specific antigens early secretory antigenic target (ESAT-6) and the 10-kDa culture filtrate protein (CFP-10), which are encoded in the Mtb-specific region of difference (RD)-1 and absent in BCG and in most of environmental mycobacteria [Citation21], leading to a better specificity compared to TST. These assays, namely ESAT-6/CFP-10 (EC)-based skin tests, are the Diaskintest (Generium, Moscow, Russian Federation), EC-skin test (Anhui Zhifei Longcom Biopharmaceutical, Anhui, China) and c-Tb test (Serum Institute of India, Pune, India) [Citation22–24] and their accuracy has been recently reported [Citation25].
IGRAs are either whole blood- or peripheral blood mononuclear cells (PBMC)-based tests. They measure the cellular immune response detecting IFN-γ released after in vitro stimulation with the Mtb-specific antigens ESAT-6 and CFP-10. The first IGRAs commercially available were the QuantiFERON-TB Gold In-Tube (QFT-GIT) (QIAGEN, Venlo, The Netherlands) and the T-SPOT.TB (Oxford Immunotec, Abingdon, UK). The QFT-GIT is a whole-blood IGRA based on enzyme-linked immunosorbent assay (ELISA) that has recently been replaced by the QuantiFERON-TB Gold Plus (QFT-Plus), the fourth generation of the QuantiFERON test. However, several studies comparing QFT-Plus with QFT-GIT in cohorts at high and low risk of TB have shown no significant improvement in the accuracy of the new tests [Citation26]. Differently, T-SPOT.TB quantifies IFN-γ production upon antigen stimulation of PBMC using the enzyme-linked immunospot (ELISPOT) assay [Citation27] ().
Table 1. Characteristics of routine and new routine tests for TBI diagnosis.
New whole blood IGRAs are available: STANDARD E TB-Feron (SD Biosensor, Suwon, Republic of Korea), AdvanSure TB-IGRA ELISA (LG Chem, Seoul, Republic of Korea) and LIOFeron TB/LTBI (LIONEX Diagnostics & Therapeutics, Braunschweig, Germany), that are based on IFN-γ detection by ELISA after stimulation with Mtb peptides or recombinant proteins [Citation28–31].
Other IGRAs based on technology different from ELISA include LIAISON QuantiFERON-TB Gold Plus (QIAGEN, Venlo, The Netherlands) and AdvanSure I3 TB-IGRA (LG Chem, Seoul, Republic of Korea), in which IFN-γ is detected by chemiluminescence immunoassay [Citation32,Citation33] and VIDAS TB-IGRA (bioMérieux, Marcy l’Etoile, France), which uses a fully automated enzyme-linked fluorescent assay (ELFA) for IFN-γ measuring [Citation30,Citation34]. Recently, simplified version of IGRAs have been developed using a lateral flow chromatography (LFA) technology that require less manual handing than ELISA-based IGRA: STANDARD TB-Feron FIA (SD Biosensor, Republic of Korea), QIAreach QuantiFERON-TB (QIAGEN, Venlo, The Netherlands), ichroma IGRA-TB (Boditech Med, Chuncheon, Republic of Korea) and Erythra TB-KIT, that is the only LFA IGRA based on PPD stimulation (Erythra Inc., Stanford, CA, U.S.A.) [Citation30,Citation35,Citation36]. Finally, GBTsol Latent TB Test Kit (Glory Biotechnologies Corp., Republic of Korea) directly detects Mtb antigen-specific T-cells through a microfilter separation of whole blood [Citation36] ().
The chemokine IFN-γ-induced protein-10 (IP-10) could be used as an alternative biomarker, because it is associated with TBI and produced at higher levels compared to IFN-γ [Citation37–39]. Interestingly, two whole blood assays, IP-10 IGRA ELISA and IP-10 IGRA LFA (rBiopharm, Darmstadt, Germany), which detect IP-10 using ELISA and LFA respectively, have been developed although not yet commercially available [Citation30].
IGRA or EC-based skin tests, differently from TST, are not affected by BCG vaccination and exposure to environmental mycobacteria. IGRA are more expensive than skin tests and require specific laboratory infrastructures thus rendering them less applicable in developing countries.
Main characteristics, advantages, and disadvantages of TST and IGRA are summarized in . All the reported tests are important to detect an immune response to Mtb, but they fail to distinguish the different stages of TB, particularly they fail to identify those at higher risk to progress to disease [Citation36,Citation40,Citation41]. Therefore, there is an urgent need to develop new tests.
3. New strategies for the diagnosis of TBI
3.1. Mtb DNA detection
An interesting approach for TBI diagnosis would be the development of a test for detecting the Mtb itself rather than the host response.
One recent approach aims to detect the presence of Mtb in circulating blood cells of TBI subjects. This attempt originated from the observation that stem cells may represent the niche for Mtb long-term intracellular viability. It has been reported that Mtb in vitro infects and survives in human bone marrow-derived mesenchymal stem cell (BM-MSC). Moreover, in ex-vivo studies, Mtb has been reported in the CD271+ BM-MSC of both mice, a model of dormant tuberculosis, and TB patients who had successfully completed anti-Mtb drug treatment [Citation42]. Other studies have reported stem cells as a potential niche for Mtb during TBI in either patients [Citation43,Citation44] or mice [Citation43,Citation45]. Interestingly, Mtb isolated from peripheral CD34+ cells of TBI subjects (asymptomatic individuals with positive IGRA) did not form colonies on agar, either because stressed or dormant. However, the Mtb became an active replicating bacterium causing disease when the CD34+infected cells were intra-tracheal injected into immune-deficient mice [Citation44]. Therefore, the DNA detection of intracellular Mtb in the bloodstream may be used as a potential biomarker for TBI diagnosis (). Several molecular methods exist to detect Mtb, thus potentially overcoming the reliance on host immune response for TBI diagnosis. However, widely used PCR platforms are not very sensitive in detecting specimens with low bacterial content such as in smear-negative or extrapulmonary TB patients. Recently, it has been reported that the sensitivity of DNA detection is significantly enhanced when the digital PCR (dPCR) methodology is applied. dPCR is a third generation of PCR that, combined with microfluidic technology, allows the absolute quantification of low level of nucleic acid target within a sample, offering a better signal-to-noise ratio than quantitative PCR (qPCR) without the presence of standard positive controls [Citation49]. dPCR is based on the division of both samples and reagents into thousands of partitions where the single PCR amplification of target genes occurs. These partitions are generated using different technologies such as emulsified microdroplets in oil [droplet dPCR (ddPCR)], manufactured microwells, or microfluidic valving. When the specific signal is detected, a threshold is assigned based on fluorescence amplitude, and the absolute target concentration is calculated by Poisson distribution, calculating the ratio of positive partitions (presence of fluorescence) over the total number of partitions, regardless of the presence of a standard curve [Citation49]. For these features, dPCR is suited for many applications that require accurate molecular characterization with high sensitivity such as in precision medicine, oncology as well as to detect the presence of microorganisms [Citation50–53].
Table 2. New strategies for M. tuberculosis DNA detection.
An important step forward in the TBI diagnosis has been made when the Mtb DNA was detected in PBMC isolated from TBI subjects using the ddPCR technology [Citation46]. Specifically, Mtb DNA was found in the 79% of an Ethiopian patient cohort; interestingly, the Mtb DNA was detected mainly in the circulating CD34+ cells rather than in CD34− cells (75% vs 22%, respectively), implying that these hematopoietic stem cells are a niche for Mtb during infection. Notably, the number of ddPCR-driven Mtb DNA detected donors was higher in people living with HIV than in HIV-uninfected donors (89% vs 73%, respectively). Since in HIV-infected patients ddPCR showed a higher sensitivity for Mtb DNA detection compared to IGRA (89% vs 33%, respectively), it is conceivable that ddPCR-based analysis can be an important assay for the TBI diagnosis in immune-compromised individuals. Remarkably, preventive therapy reduced Mtb DNA detection in the 44% of HIV-infected people that were scored positive before therapy. These results suggest a potential application of this test also for monitoring the outcome of preventive therapy [Citation46].
A further approach to increase Mtb identification by molecular methods is the improvement of DNA extraction. To this aim, it has been used a lytic bacteriophage-based blood assay [Citation47]. Bacteriophages are viruses that infect bacteria and have two distinct life cycles. They may be either lytic that replicate and break open their host, thus releasing bacteria genomic DNA, or temperate that integrate into the host chromosome. Verma and colleagues [Citation47] utilized the lytic bacteriophage-based blood assay actiphage (PBD Biotech Ltd) to extract Mtb DNA from PBMC of patients with either TB disease (i.e., Xpert-Ultra- or Mtb culture-positive) or TBI (IGRA-positive). Notably, actiphage-based DNA extraction was performed using PBMC isolated from only 2 mL of blood and Mtb was detected after DNA concentration and standard qPCR using the multicopy IS6110 gene as target. Although in this work very few patients were analyzed, Mtb DNA was detected in most of TB disease patients (11/15, 73%). On the other hand, among the 18 asymptomatic IGRA positive individuals, only 3 were scored Mtb DNA positive (17%). Very interestingly, 2 of them progressed to TB disease within 7 months, thus indicating that this methodology may be utilized as predictor for TB progression [Citation47]. Unfortunately, the absence of a larger diagnostic trial using this technology does not allow to draw definitive conclusions on the potential diagnostic power of this bacteriophage-based blood assay, and therefore further studies are needed.
Another approach was based on the detection of a cell-free form of Mtb DNA in the plasma of patients with either pulmonary TB or TBI, using ddPCR or qPCR methodology [Citation48]. While cell-free Mtb DNA was found in the 54.2% (13 out of 24) of TB patients, it was detected only in the 3.5% (2 out of 57) of TBI donors [Citation48]. It is unknown if the TBI subjects’ cell-free-Mtb DNA positive eventually progressed to TB disease.
3.2. Mtb antigens detection in blood circulation
Another approach to identify Mtb is the direct detection of its proteins in the plasma of TB disease or TBI subjects. The dormant/low replicative bacillus present in a granuloma is under hypoxic conditions with limited access to nutrients. If the bacilli reactivate, this leads to the expression of Mtb antigens such as Ag85A, ESAT-6, and CFP-10, which can be detected either as proteins or peptides and used as a target for diagnosis. Although these studies were designed to discriminate TB disease from TBI, some information can help us to develop a strategy for defining the different TB stages.
An assay based on the combination of 4 Mtb proteins (Rv1860, RV3881c, Rv2031c, and Rv3803c) reached a sensitivity of 93.3% and specificity of 97.7% for distinguishing TB disease from TBI using a protein microarray [Citation54]. These results were confirmed in plasma samples of non-human primates with TBI in which low detection of CFP-10-derived peptides was found compared to those with TB disease (i.e., Mtb infection at high doses) by a mass spectrometry (MS)-based approach [Citation55]. Similarly, peptides of ESAT-6 and CFP-10 were detected by MS in the blood circulation of TB disease patients (25 out of 27; 92.6%) but also in 4 out of 31 (12.9%) TBI individuals [Citation56]. It is unknown whether these 4 TBI subjects developed TB disease. Interestingly, Mtb peptides from 8 proteins, which are known to be involved in the intracellular survival of Mtb (Ag85B, Ag85C, Apa, BfrB, GlcB, HspX, KatG, and Mpt64), detected by MS have been found in the circulating exosomes of TBI individuals, regardless of HIV status [Citation57].
Overall, these data indicate that the detection of Mtb proteins/peptides may help to develop a strategy for defining the different TB stages, possibly from incipient TB to TB disease (). Interestingly, peptidomic studies have been performed in animal models to identify Mtb antigens valuable for Mtb detection for diagnostic purposes [Citation58].
Table 3. New strategies for M. tuberculosis antigens detection.
3.3. Antibodies anti-Mtb antigens detection in blood circulation
The role of humoral immunity in the protection against Mtb infection and disease is not yet fully understood, however there is evidence supporting the role of antibodies in the establishment of an effective immune response against Mtb [Citation59]. In this regard, the detection of specific anti-Mtb antibodies in the circulation may represent an alternative and/or a diagnostic aid for TB identification/classification. In fact, although the antibody-based assays for TB diagnosis showed modest sensitivity and specificity, they can be developed into simple, cost-effectiveness, and fast test performance for their use in resource limited setting [Citation60].
In a Japanese cohort of TBI subjects it has been measured the host humoral response to Mtb proteins expressed either in the growth phase of Mtb (i.e., ESAT6, CFP10, and Ag85A), or in the dormant phase of the bacillus (mycobacterial DNA‐binding protein 1, MDP1, and alpha‐crystallin like protein, Acr). The authors found that the serum levels of these antibodies were higher in TB disease patients compared to those with TBI. Importantly, they found that the antibody titers against these Mtb antigens, with the exception of anti-CFP10, were significantly higher in subjects with recent TBI (n = 13) compared to those with remote TBI (n = 12) [Citation61]. Another Mtb antigen that induces a strong antibody response is the Mtb proline-proline-glutamic acid protein PPE17 (Rv1168c). In a study conducted in India, anti-PPE17 antibodies were detected in 53 out of 61 (87%) of TBI individuals (QFT–GIT positive) compared to non-TB control donors. The levels of anti-PPE17 were significantly higher compared to those of antibodies directed against other Mtb antigens such as ESAT-6, CFP-10 and PPD. Unfortunately, antibodies anti-PPE17 levels were not able to discriminate between the TBI and TB disease patients [Citation62].
Less recently, the humoral response to PPE55, another member of Mtb PPE proteins, has been investigated. The antibodies levels to the C-terminal part of PPE55 (PPE-C) have been retrospectively detected in the sera of 17 out of 21 (81%) of HIV-infected patients prior the development of the TB disease, suggesting that this protein is expressed during incipient and/or subclinical TB, at least in HIV-infected humans [Citation63]. Interestingly, the cellular response, in terms of IFN-γ and IL-17 production by whole blood stimulated with the same protein, has been found increased in health care workers exposed to Mtb compared to that of TB patients [Citation64].
To identify differences in humoral response between TB disease and TBI subjects, a screening for both the presence and the quality of specific anti-Mtb antibodies has been performed using 209 different Mtb antigens as target. Changes in antigen-recognition by the different antibody serotypes between TB disease and TBI subjects have been found [Citation65]. Interestingly, it was possible to define a minimal set of antibodies that i) recognize specific Mtb antigens and ii) carries peculiar Fc-glycosylation, which influences the binding to Fcγ-receptors (FcγR2AR, FcγR2B, FcγR3AV, and FcγR3B). Considering the antibodies levels and their Fc receptor-binding characteristics, the authors discriminated TB disease and TBI in HIV-uninfected patients. Among all the possible antigen-specific antibody combinations, only 4 characteristics were sufficient, RV2034-specific FcγR2AR binding was higher in TBI subjects, while RV1528-specific FcγR2B, LAM-specific FcγR3A binding antibodies, and RV2435c IgG1 levels were predominant in TB disease patients. On the other hand, in the HIV-infected patients, RV3583-specific FcγR2AR binding was enriched in TB disease individuals, while anti-RV1508 and anti-Ag85A IgM levels were elevated in the TBI group [Citation65].
Interestingly, it has been also shown that a different humoral Mtb-specific response quality occurs in patients with TB disease or TBI. Mtb-specific antibodies were analyzed and selected by 70 different features including the ability to induce antibody-dependent cellular phagocytosis, and cellular cytotoxicity in response to PPD and Mtb culture fractionations [Citation66]. TBI was found associated with a different immunoglobulin Fc structural and functional profiles. The antibody glycosylation pattern of TBI subjects had less fucose and more galactose and acid sialic compared to that of TB disease patients. Importantly, these differences were associated to distinct functional properties of antibodies. TBI-derived antibodies in response to PPD showed enhanced NK cell–mediated antibody-dependent cellular cytotoxicity, NK cell degranulation, binding to the FcγRIIIa, which is an activating FcRs. Notably, using Mtb-infected primary human macrophages, the authors found that the antibodies derived by TBI subjects increase the co-localization of Mtb with lysosomes and IL1β secretion and, mainly, reduced the intracellular survival of Mtb, when compared to the antibodies derived by TB disease patients [Citation66]. These results indicate that TBI can be characterized by the type of antibodies induced, which may affect the outcome of Mtb intracellular survival.
The different antibodies qualities between TB and TBI subjects has been recently confirmed [Citation67,Citation68]. It has been showed that the di-galactosylated glycan structures (lacking sialic acid) found on IgG-Fc associate with TBI; interestingly, these Abs increase after TB cure. Moreover, the anti-TB-specific IgG4 antibodies associated with TB disease and diminished at the end of TB therapy [Citation68].
Another possible approach to identify new targets for a serological screening was attempted using synthetic mycolic acids, which are similar to Mtb lipid antigens and contain long-chain mycolic acids such as non-wall-bound trehalose, either dimycolates (TDM) or monomycolates (TMM). TDM and TMM are a mixture of different carbon chain lengths that represent a specific Mycobacterium fingerprint. It has been reported that it is possible to identify TB patients with a sensitivity and specificity of 96% and 95%, respectively, when seven antigens were combined, while one single antigen obtained a sensitivity of 85% and a specificity of 88% [Citation69]. Interestingly, using the same approach, it was possible to identify antigens for the serodiagnosis of nontuberculous mycobacterial infections [Citation70].
Overall, these results suggest that the humoral response to Mtb could be used for better defining TB stages ().
Table 4. New strategies for detection of anti-M. tuberculosis antibodies.
3.4. Host blood transcriptomics
Another possible strategy for the diagnosis of the different TB stages is the transcriptomic approach, which characterizes the host response to Mtb and reflects changes in gene expression between different disease states [Citation71]. A large number of host blood mRNA transcript signatures have been identified with the aim to i) detect and differentiate TBI from other respiratory diseases and ii) predict progression from TBI to disease (). The WHO established a Target Product Profile (TPP), which describes the minimal and optimal requirements for non-sputum-based TB diagnostic and prognostic tests [Citation80,Citation81]. In particular, for a test that can predict progression to TB disease in two-year time, the optimal targets for sensitivity and specificity are both 90% while the minimal targets are both 75% [Citation82].
Table 5. Host blood transcriptomic signatures for incipient TB diagnosis.
Efforts for the analysis of the blood transcriptional profile of subjects at different stage of the Mtb infection has been made by several research groups. Among them, Zak et al [Citation72] identified a 16-genes expression signature in a cohort of South African adolescents for predicting the risk of TB disease progression. The authors compared the whole blood RNA sequencing data of subjects with TBI who developed TB disease within 2 years with those who remained healthy. Analyzing 46 TB progressors and 107 matched controls a signature of 16 genes involved in the IFN response was identified [Citation72]. After identification, the signature was validated in two independent cohorts, TBI adolescent donors and household contacts of TB patients from South Africa and Gambia. This signature predicted disease progression within 12 months with sensitivity and specificity of 66.1% and 80.6%, and 53.7% and 82.8%, respectively [Citation72].
More recently, a 4-genes ‘Pan African’ blood signature to predict the risk of progression to TB disease with a broader application in South African, Gambian, and Ethiopian cohorts of recently exposed household contacts of TB was identified and validated [Citation73]. This signature predicted disease progression with an AUC (Area Under the Curve) of 0.66 within 0–1 year (p = 0.002) or AUC = 0.69 within 1 − 2 years (p = 0.02). Interestingly, this 4-gene signature predicted TB progression with a similar accuracy for the three different cohorts, characterized by heterogeneous genetic background, TB epidemiology and Mtb strains, therefore indicating that this signature had a good generalizable prognostic value [Citation73].
The 16-genes signature [Citation72] was subsequently reduced to 11 genes (RISK11) with equivalent diagnostic and prognostic performance [Citation74]. Of note, the performance of this 11-gene expression signature was not different in whole blood and cryopreserved PBMC from HIV-uninfected adults [Citation74]. On the other hand, this transcriptomic signature showed limited prognostic performance for TB disease in HIV-infected individuals on antiretroviral therapy, while it was helpful for following the outcome of TB treatment [Citation75].
In a patient-level pooled meta-analysis, the signatures identified by Zak and Suliman [Citation72,Citation73] were found to reach the minimum accuracy benchmark in WHO’s TPP for predicting TB disease over a short period of 3 months (positive-predictive values 11.2–14.4%). However, none of the 8 evaluated signatures met the minimal target for predictive performance over 2 years (positive-predictive values 6.8–9.4%) [Citation83].
Recently, the diagnostic and prognostic performance of RISK11 was evaluated in HIV-uninfected and HIV-infected individuals in South Africa [Citation76,Citation77]. Among adults without HIV, RISK11 was able to detect microbiologically confirmed TB at enrollment and predicts progression to TB disease of TBI patients within 12 months. However, while the accuracy to predict TB disease exceeded the optimal target within 6 months, it did not meet the minimal TPP criteria over two years [Citation76]. Similarly, it has been shown that RISK11 can be used to diagnose TB and predict risk of progression to TB disease within 15 months in people with HIV infection in a community setting, although the performance did not meet WHO TPP benchmarks for triage and prognostic tests for TB [Citation77].
A six genes transcriptomic signature, named RISK6, was identified comparing blood samples from South African adolescent TB progressors and non-progressor consisting of three transcripts upregulated (GBP2, FCGR1B, and SERPING1) and three transcripts downregulated (TUBGCP6, TRMT2A, and SDR39U1) in TB progressors [Citation78]. The authors compared the progression prognostic performance of RISK 6 with that of 16-genes expression [Citation72] and RISK11 [Citation73] signatures by a model fit, demonstrating a significantly better value (AUC 87.6% vs 81.8% vs 82.2%, respectively). However, RISK6 did not meet the WHO TPP accuracy requirements for a test that predicts the progression from TBI to TB disease [Citation78]. Interestingly, the RISK6 signature was also validated for the capability of be a reliable biomarker for TB treatments monitoring, its score was higher in TB disease patients while significantly decreased after TB treatment [Citation78,Citation79]. Notably, the diagnostic performance of RISK6 tested in blood collected in PAXgene tubes and in capillary blood collected by finger prick was not significantly different from that collected by venous blood sampling, therefore indicating the possibility to translate this research test into a diagnostic test [Citation78].
In a recent work prognostic performance of different mRNA profiles have been compared, finding that the signatures can meet TPP criteria only in the short term [Citation84]. In fact, all signatures had excellent performance for detecting HIV-uninfected participants who progressed to TB disease within 6 months. In particular, RISK11 [Citation74] achieved the optimal target for predicting TB incidence up to 6 months and RISK6 [Citation78] met the minimal target up to 6 months. However, none of the signatures met the minimal requirements for a prognostic test (defined as 75% sensitivity and 75% specificity) within 15 months [Citation84]. Therefore, no prognostic signatures can accurately predict progression to TB disease over 2 years, both in HIV-uninfected and HIV-infected individuals.
4. Conclusions
There is currently no gold standard test to diagnose patients with TBI. However, several procedures for TBI identification are available either as routine or research test (). The experimental tests are based on either the direct identification of Mtb in the circulation or the host response to Mtb. However, up to now, these tests lack accuracy to identify patients that progress from infection to TB disease. A desirable screening test allows i) the identification of TBI patients and ii) indicates progression to TB disease. This needed test, based on the either host or Mtb load detection, would be used as a second confirmation analysis after performing routine EC-based skin or IGRA test (). In conclusion, these experimental tests need further evaluation and validation in randomized clinical trials to prove their ability to predict TB progression with high accuracy.
Figure 1. Routine and research tests for TBI diagnosis. Diagnosis of TBI may be based on the detection of either host responses to Mtb antigens or of the pathogen itself. The routine immune-based tests currently used are the TST, new versions of EC-based skin tests, IGRA and its new developments. Other possible strategies are based on the detection of host factors such as the serological approach, for the detection of mycobacterial-specific antibodies, and the transcriptomic approach, which characterizes the host response in unstimulated blood, reflecting changes in gene expression between different disease states. On the other hand, microbiological tests able to identify Mtb itself are based on the detection of Mtb DNA or antigens from blood circulation. EC: ESAT-6/CFP-10; IGRA: interferon (IFN)-γ release assays; Mtb: Mycobacterium tuberculosis; NK: natural killer; TBI: tuberculosis infection; TST: tuberculin skin test. Created with BioRender.com.
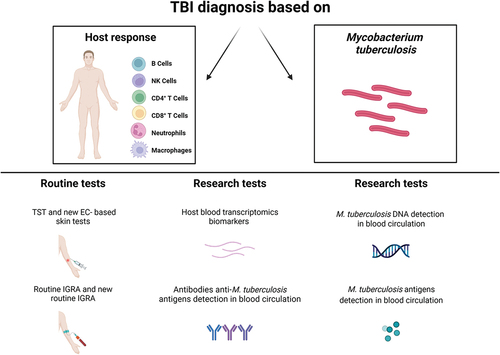
Figure 2. Routine and research tests for the identification of TB progressors. TBI individuals are routinely screened by EC-based skin tests or IGRA that reveal host immune responses to Mtb, in absence of clinically and/or microbiological manifestations of TB disease. The 5–10% of TBI individuals can develop TB disease in their life span, and half of those develop the disease within the first 2 years from infection. Progressors from TBI to TB disease may be identified by host-based or pathogen-based diagnostic tests. EC: ESAT-6/CFP-10; IGRA: interferon (IFN)-γ release assays; Mtb: Mycobacterium tuberculosis; TBI: tuberculosis infection; TST: tuberculin skin test. Created with BioRender.com.
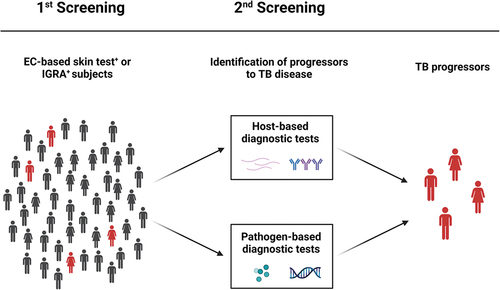
5. Expert opinion
TBI is diagnosed by measuring a specific-cellular immune response to mycobacterial antigens in the absence of symptoms, radiological and microbiological evidence of the disease. TST and IGRA are the routine tests used to measure the immune response; however, they do not discriminate TBI from TB disease and do not identify the progressors from TBI to TB disease with high accuracy. Moreover, these tests cannot be used to predict whether preventive therapy for TBI is effective for decreasing the risk to develop TB disease.
In the last years, several new research-based tests for TBI have been developed. They are based on either the direct detection of Mtb or the host response to the infection. Although these new experimental tests could represent a crucial step forward in TBI diagnosis, many limitations are yet to be overcome for their use in clinical practice. For instance, there is still lack of validated evidence of progression to TB disease of those scored positive to assays detecting Mtb either evaluating DNA or peptides or proteins. The answer to this fundamental question could be obtained solely with dedicated clinical trials, likely carried out in TB endemic regions, where the progression from TBI to TB disease is more probable to be observed. On the other hand, technical challenges must be resolved like for example those related to the complexity to detect Mtb antigens which at present, are not suitable for diagnostic procedures. This is a significant drawback, particularly in developing countries where laboratory infrastructures are still limited. However, it is conceivable that upon the presence of reliable proof of concept for the diagnosis of TBI and progression to TB disease, the industry will surely invest to develop sustainable kits for several aspects including the technology used such as characteristics of the instruments (i.e. size, material, temperature stability, electricity autonomy) and the cost.
In contrast, an assay based on the detection of specific circulating antibodies against Mtb antigens may be developed into simple, inexpensive, and rapid tests, which can also be used in low resource settings. However, until now these tests have shown modest accuracy for TBI diagnosis or TB progressors identification.
At present, the host blood transcriptomic signature is the most clinically validated test for detection of TB progressors. Several clinical trials have been carried out in TB endemic regions, which allowed to design reliable signatures composed by few host RNAs. However, since they are based on host intrinsic characteristics, they may not be specific for TB and overlap with TB-unrelated pneumonia signatures. Moreover, host signatures may also depend on the immune status of the patients, which may be influenced by the presence of chronic coinfections, such as HIV, HBV or HCV or the age [Citation85], immunosuppression induced by therapies, or immune alterations influenced by the host genetic background.
Overall, to date the research tests available still lack the required accuracy in identifying either TBI or TB progressors. Further efforts from research laboratories and from clinicians are needed to design new reliable tools and to test them in specific clinical trials. Simplification of the procedures is also required to develop a translational pipeline for diagnostic application.
Declaration of interest
The authors have no other relevant affiliations or financial involvement with any organization or entity with a financial interest in or financial conflict with the subject matter or materials discussed in the manuscript apart from those disclosed.
Reviewers disclosure
Peer reviewers on this manuscript have no relevant financial relationships or otherwise to disclose.
Acknowledgments
We sincerely apologize to all those colleagues whose important work was not cited due to space constraints.
Additional information
Funding
References
- Rahlwes KC, Dias BRS, Campos PC, et al. Pathogenicity and virulence of Mycobacterium tuberculosis. Virulence. 2023;14(1):2150449. doi: 10.1080/21505594.2022.2150449
- WHO. Global tuberculosis report 2022 [Internet]. [cited 2023 Mar 22]. Available from: https://www.who.int/publications-detail-redirect/9789240061729.
- Migliori GB, Nardell E, Yedilbayev A, et al. Reducing tuberculosis transmission: a consensus document from the world health organization regional office for Europe. Eur Respir J. 2019;53(6):1900391. doi: 10.1183/13993003.00391-2019
- Migliori GB, Ong CWM, Petrone L, et al. The definition of tuberculosis infection based on the spectrum of tuberculosis disease. Breathe. 2021;17(3):210079. doi: 10.1183/20734735.0079-2021
- WHO. WHO consolidated guidelines on tuberculosis: module 1: prevention: tuberculosis preventive treatment [Internet]. [cited 2023 Mar 23]. Available from: https://www.who.int/publications-detail-redirect/9789240001503.
- Carabalí-Isajar ML, Rodríguez-Bejarano OH, Amado T, et al. Clinical manifestations and immune response to tuberculosis. World J Microbiol Biotechnol. 2023;39(8):206. doi: 10.1007/s11274-023-03636-x
- Drain PK, Bajema KL, Dowdy D, et al. Incipient and subclinical tuberculosis: a clinical review of early stages and progression of infection. Clin Microbiol Rev. 2018;31(4):e00021–18. doi: 10.1128/CMR.00021-18
- Goletti D, Pisapia R, Fusco FM, et al. Epidemiology, pathogenesis, clinical presentation and management of TB in patients with HIV and diabetes. Int J Tuberc Lung Dis. 2023;27(4):284–290. doi: 10.5588/ijtld.22.0685
- Cantini F, Niccoli L, Capone A, et al. Risk of tuberculosis reactivation associated with traditional disease modifying anti-rheumatic drugs and non-anti-tumor necrosis factor biologics in patients with rheumatic disorders and suggestion for clinical practice. Expert Opin Drug Saf. 2019;18(5):415–425. doi: 10.1080/14740338.2019.1612872
- Cantini F, Blandizzi C, Niccoli L, et al. Systematic review on tuberculosis risk in patients with rheumatoid arthritis receiving inhibitors of Janus Kinases. Expert Opin Drug Saf. 2020;19(7):861–872. doi: 10.1080/14740338.2020.1774550
- Petruccioli E, Petrone L, Chiacchio T, et al. Mycobacterium tuberculosis immune response in patients with immune-mediated inflammatory disease. Front Immunol. 2021;12:716857. doi: 10.3389/fimmu.2021.716857
- Ong CWM, Migliori GB, Raviglione M, et al. Epidemic and pandemic viral infections: impact on tuberculosis and the lung: A consensus by the World Association for Infectious Diseases and Immunological Disorders (WAidid), Global Tuberculosis Network (GTN), and members of the European Society of Clinical Microbiology and Infectious Diseases Study Group for Mycobacterial Infections (ESGMYC). Eur Respir J. 2020;56(4):2001727. https://erj.ersjournals.com/content/56/4/2001727
- Sarkar S, Khanna P, Singh AK. Impact of COVID-19 in patients with concurrent co-infections: A systematic review and meta-analyses. J Med Virol. 2021;93(4):2385–2395. doi: 10.1002/jmv.26740
- du Bruyn E, Stek C, Daroowala R, et al. Effects of tuberculosis and/or HIV-1 infection on COVID-19 presentation and immune response in Africa. Nat Commun. 2023;14(1):188. doi: 10.1038/s41467-022-35689-1
- Najafi-Fard S, Aiello A, Navarra A, et al. Characterization of the immune impairment of patients with tuberculosis and COVID-19 coinfection. Int J Infect Dis. 2023;130:S34–S42.
- Migliori GB, Wu SJ, Matteelli A, et al. Clinical standards for the diagnosis, treatment and prevention of TB infection. Int J Tuberc Lung Dis. 2022;26(3):190–205. doi: 10.5588/ijtld.21.0753
- Goletti D, Sanduzzi A, Delogu G. Performance of the tuberculin skin test and interferon-γ release assays: an update on the accuracy, cutoff stratification, and new potential immune-based approaches. J Rheumatol Suppl. 2014;91:24–31. doi: 10.3899/jrheum.140099
- Petruccioli E, Scriba TJ, Petrone L, et al. Correlates of tuberculosis risk: predictive biomarkers for progression to active tuberculosis. Eur Respir J. 2016;48(6):1751–1763. doi: 10.1183/13993003.01012-2016
- Goletti D, Petrone L, Ippolito G, et al. Preventive therapy for tuberculosis in rheumatological patients undergoing therapy with biological drugs. Expert Rev Anti Infect Ther. 2018;16(6):501–512. doi: 10.1080/14787210.2018.1483238
- Santos JA, Duarte R, Nunes C. Tuberculin skin test and interferon-γ release assays: Can they agree? Clin Respir J. 2023;17(2):109–114. doi: 10.1111/crj.13569
- Arend SM, Geluk A, van Meijgaarden KE, et al. Antigenic equivalence of human T-Cell responses to mycobacterium tuberculosis-specific RD1-Encoded Protein Antigens ESAT-6 and culture filtrate protein 10 and to mixtures of synthetic peptides. Infect Immun. 2000;68(6):3314–3321. doi: 10.1128/IAI.68.6.3314-3321.2000
- Li F, Xu M, Qin C, et al. Recombinant fusion ESAT6-CFP10 immunogen as a skin test reagent for tuberculosis diagnosis: an open-label, randomized, two-centre phase 2a clinical trial. Clin Microbiol Infect. 2016;22(10):.e889.9–.e889.16. doi: 10.1016/j.cmi.2016.07.015
- Ruhwald M, Aggerbeck H, Gallardo RV, et al. Safety and efficacy of the C-Tb skin test to diagnose Mycobacterium tuberculosis infection, compared with an interferon γ release assay and the tuberculin skin test: a phase 3, double-blind, randomised, controlled trial. Lancet Respir Med. 2017;5(4):259–268. doi: 10.1016/S2213-2600(16)30436-2
- Nikitina IY, Karpina NL, Kasimceva OV, et al. Comparative performance of QuantiFERON-TB Gold versus skin test with tuberculosis recombinant allergen (Diaskintest) among patients with suspected pulmonary tuberculosis in Russia. Int J Infect Dis IJID Off Publ Int Soc Infect Dis. 2019;86:18–24.
- Krutikov M, Faust L, Nikolayevskyy V, et al. The diagnostic performance of novel skin-based in-vivo tests for tuberculosis infection compared with purified protein derivative tuberculin skin tests and blood-based in vitro interferon-γ release assays: a systematic review and meta-analysis. Lancet Infect Dis. 2022;22(2):250–264. doi: 10.1016/S1473-3099(21)00261-9
- Shafeque A, Bigio J, Hogan CA, et al. Fourth-generation quantiferon-TB gold plus: what is the evidence? J Clin Microbiol. 2020;58(9):e01950–19. doi: 10.1128/JCM.01950-19
- Goletti D, Carrara S, Vincenti D, et al. Accuracy of an immune diagnostic assay based on RD1 selected epitopes for active tuberculosis in a clinical setting: a pilot study. Clin Microbiol Infect Off Publ Eur Soc Clin Microbiol Infect Dis. 2006;12(6):544–550. doi: 10.1111/j.1469-0691.2006.01391.x
- Kweon OJ, Lim YK, Kim HR, et al. Evaluation of standard E TB-Feron Enzyme-linked immunosorbent assay for diagnosis of latent tuberculosis infection in health care workers. J Clin Microbiol. 2019;57(12):e01347–19. doi: 10.1128/JCM.01347-19
- Della Bella C, Spinicci M, Alnwaisri HFM, et al. Lioferon®tb/LTBI: A novel and reliable test for LTBI and tuberculosis. Int J Infect Dis IJID Off Publ Int Soc Infect Dis. 2020;91:177–181. doi: 10.1016/j.ijid.2019.12.012
- Hamada Y, Cirillo DM, Matteelli A, et al. Tests for tuberculosis infection: landscape analysis. Eur Respir J. 2021;58(5):2100167. doi: 10.1183/13993003.00167-2021
- Miotto P, Goletti D, Petrone L. Making IGRA testing easier: First performance report of QIAreach QFT for tuberculosis infection diagnosis. Pulmonology. 2022;28(1):4–5. doi: 10.1016/j.pulmoe.2021.07.010
- Kim JJ, Park Y, Choi D, et al. Performance evaluation of a new automated chemiluminescent immunoanalyzer-based interferon-gamma releasing assay advansure I3 in comparison with the quantiFERON-TB gold in‑tube assay. Ann Lab Med. 2020;40(1):33–39. doi: 10.3343/alm.2020.40.1.33
- De Maertelaere E, Vandendriessche S, Verhasselt B, et al. Evaluation of QuantiFERON-TB gold plus on liaison XL in a low-tuberculosis-incidence setting. J Clin Microbiol. 2020;58(4):e00159–20. doi: 10.1128/JCM.00159-20
- Petruccioli E, Farroni C, Cuzzi G, et al. VIDAS® TB-IGRA reagents induce a CD4+ and CD8+ T-cell IFN-γ response for both TB infection and active TB. Int J Tuberc Lung Dis Off J Int Union Tuberc Lung Dis. 2022;26(1):65–68. doi: 10.5588/ijtld.21.0478
- Hur Y-G, Hong JY, Choi DH, et al. A feasibility study for diagnosis of latent tuberculosis infection using an IGRA point-of-care platform in South Korea. Yonsei Med J. 2019;60(4):375–380. doi: 10.3349/ymj.2019.60.4.375
- Goletti D, Delogu G, Matteelli A, et al. The role of IGRA in the diagnosis of tuberculosis infection, differentiating from active tuberculosis, and decision making for initiating treatment or preventive therapy of tuberculosis infection. Int J Infect Dis IJID Off Publ Int Soc Infect Dis. 2022;124 Suppl 1:S12–S19. doi: 10.1016/j.ijid.2022.02.047
- Goletti D, Raja A, Ahamed Kabeer BS, et al. IFN-γ, but not IP-10, MCP-2 or IL-2 response to RD1 selected peptides associates to active tuberculosis. J Infect. 2010;61(2):133–143. doi: 10.1016/j.jinf.2010.05.002
- Kabeer BSA, Raja A, Raman B, et al. IP-10 response to RD1 antigens might be a useful biomarker for monitoring tuberculosis therapy. BMC Infect Dis. 2011;11(1):135. doi: 10.1186/1471-2334-11-135
- Santos VS, Goletti D, Kontogianni K, et al. Acute phase proteins and IP-10 as triage tests for the diagnosis of tuberculosis: systematic review and meta-analysis. Clin Microbiol Infect. 2019;25(2):169–177. doi: 10.1016/j.cmi.2018.07.017
- Gupta RK, Lipman M, Jackson C, et al. Quantitative IFN-γ release assay and tuberculin skin test results to predict incident tuberculosis. A prospective cohort study. Am J Respir Crit Care Med. 2020;201(8):984–991. doi: 10.1164/rccm.201905-0969OC
- Zhou G, Luo Q, Luo S, et al. Interferon-γ release assays or tuberculin skin test for detection and management of latent tuberculosis infection: a systematic review and meta-analysis. Lancet Infect Dis. 2020;20(12):1457–1469. doi: 10.1016/S1473-3099(20)30276-0
- Das B, Kashino SS, Pulu I, et al. CD271 + bone marrow mesenchymal stem cells may provide a niche for dormant mycobacterium tuberculosis. Sci Transl Med. 2013;5(170):170ra13. doi: 10.1126/scitranslmed.3004912
- Garhyan J, Bhuyan S, Pulu I, et al. Preclinical and clinical evidence of mycobacterium tuberculosis persistence in the hypoxic niche of bone marrow mesenchymal stem cells after therapy. Am J Pathol. 2015;185(7):1924–1934. doi: 10.1016/j.ajpath.2015.03.028
- Tornack J, Reece ST, Bauer WM, et al. Human and mouse hematopoietic stem cells are a depot for dormant mycobacterium tuberculosis. Plos One. 2017;12(1):e0169119. doi: 10.1371/journal.pone.0169119
- Reece ST, Vogelzang A, Tornack J, et al. Mycobacterium tuberculosis-infected hematopoietic stem and progenitor cells unable to express inducible nitric oxide synthase propagate tuberculosis in Mice. J Infect Dis. 2018;217(10):1667–1671. doi: 10.1093/infdis/jiy041
- Belay M, Tulu B, Younis S, et al. Detection of Mycobacterium tuberculosis complex DNA in CD34-positive peripheral blood mononuclear cells of asymptomatic tuberculosis contacts: an observational study. Lancet Microbe. 2021;2(6):e267–e275. doi: 10.1016/S2666-5247(21)00043-4
- Verma R, Swift BMC, Handley-Hartill W, et al. A novel, high-sensitivity, bacteriophage-based assay identifies low-level mycobacterium tuberculosis bacteremia in immunocompetent patients with active and incipient tuberculosis. Clin Infect Dis Off Publ Infect Dis Soc Am. 2020;70:933–936. doi: 10.1093/cid/ciz548
- Pan S-W, Su W-J, Chan Y-J, et al. Mycobacterium tuberculosis–derived circulating cell-free DNA in patients with pulmonary tuberculosis and persons with latent tuberculosis infection. Plos One. 2021;16(6):e0253879. doi: 10.1371/journal.pone.0253879
- Hindson BJ, Ness KD, Masquelier DA, et al. High-throughput droplet digital PCR system for absolute quantitation of DNA copy number. Anal Chem. 2011;83(22):8604–8610. doi: 10.1021/ac202028g
- Lei S, Chen S, Zhong Q. Digital PCR for accurate quantification of pathogens: Principles, applications, challenges and future prospects. Int j biol macromol. 2021;184:750–759. doi: 10.1016/j.ijbiomac.2021.06.132
- Zhang L, Parvin R, Fan Q, et al. Emerging digital PCR technology in precision medicine. Biosens Bioelectron. 2022;211:114344. doi: 10.1016/j.bios.2022.114344
- Merino I, de la Fuente A, Domínguez-Gil M, et al. Digital PCR applications for the diagnosis and management of infection in critical care medicine. Crit Care. 2022;26(1):63. doi: 10.1186/s13054-022-03948-8
- Olmedillas-López S, Olivera-Salazar R, García-Arranz M, et al. Current and emerging applications of droplet digital pcr in oncology: an updated review. Mol Diagn Ther. 2022;26(1):61–87. doi: 10.1007/s40291-021-00562-2
- Li J, Wang Y, Yan L, et al. Novel serological biomarker panel using protein microarray can distinguish active TB from latent TB infection. Microbes Infect. 2022;24(8):105002. doi: 10.1016/j.micinf.2022.105002
- Shu Q, Liu S, Alonzi T, et al. Assay design for unambiguous identification and quantification of circulating pathogen-derived peptide biomarkers. Theranostics. 2022;12(6):2948–2962. doi: 10.7150/thno.70373
- Liu C, Zhao Z, Fan J, et al. Quantification of circulating Mycobacterium tuberculosis antigen peptides allows rapid diagnosis of active disease and treatment monitoring. Proc Natl Acad Sci U S A. 2017;114(15):3969–3974. doi: 10.1073/pnas.1621360114
- Kruh-Garcia NA, Wolfe LM, Chaisson LH, et al. Detection of mycobacterium tuberculosis peptides in the exosomes of patients with active and latent M. tuberculosis infection using MRM-MS. Plos One. 2014;9(7):e103811. doi: 10.1371/journal.pone.0103811
- Chen H, Li S, Zhao W, et al. A peptidomic approach to identify novel antigen biomarkers for the diagnosis of tuberculosis. Infect Drug Resist. 2022;15:4617–4626. doi: 10.2147/IDR.S373652
- Rijnink WF, Ottenhoff THM, Joosten SA. B-Cells and antibodies as contributors to effector immune responses in tuberculosis. Front Immunol. 2021;12:640168. doi: 10.3389/fimmu.2021.640168
- Melkie ST, Arias L, Farroni C, et al. The role of antibodies in tuberculosis diagnosis, prophylaxis and therapy: a review from the ESGMYC study group. Eur Respir Rev Off J Eur Respir Soc. 2022;31(163):210218. doi: 10.1183/16000617.0218-2021
- Maekura R, Kitada S, Osada-Oka M, et al. Serum antibody profiles in individuals with latent Mycobacterium tuberculosis infection. Microbiol Immunol. 2019;63(3–4):130–138. doi: 10.1111/1348-0421.12674
- Abraham PR, Devalraju KP, Jha V, et al. PPE17 (Rv1168c) protein of Mycobacterium tuberculosis detects individuals with latent TB infection. Plos One. 2018;13(11):e0207787. doi: 10.1371/journal.pone.0207787
- Singh KK, Dong Y, Patibandla SA, et al. Immunogenicity of the Mycobacterium tuberculosis PPE55 (Rv3347c) protein during incipient and clinical tuberculosis. Infect Immun. 2005;73(8):5004–5014. doi: 10.1128/IAI.73.8.5004-5014.2005
- Alvarez-Corrales N, Ahmed RK, Rodriguez CA, et al. Differential cellular recognition pattern to M. tuberculosis targets defined by IFN-γ and IL-17 production in blood from TB + patients from Honduras as compared to health care workers: TB and immune responses in patients from Honduras. BMC Infect Dis. 2013;13(1):125. doi: 10.1186/1471-2334-13-125
- Nziza N, Cizmeci D, Davies L, et al. Defining discriminatory antibody fingerprints in active and latent tuberculosis. Front Immunol. 2022;13:856906. doi: 10.3389/fimmu.2022.856906
- Lu LL, Chung AW, Rosebrock TR, et al. A functional role for antibodies in tuberculosis. Cell. 2016;167(2):433–443.e14. doi: 10.1016/j.cell.2016.08.072
- Lu LL, Das J, Grace PS, et al. Antibody Fc glycosylation discriminates between latent and active tuberculosis. J Infect Dis. 2020;222(12):2093–2102. doi: 10.1093/infdis/jiz643
- Grace PS, Dolatshahi S, Lu LL, et al. Antibody subclass and glycosylation shift following effective TB treatment. Front Immunol. 2021;12:679973. DOI:10.3389/fimmu.2021.679973
- Jones A, Pitts M, Al Dulayymi JR, et al. New synthetic lipid antigens for rapid serological diagnosis of tuberculosis. Plos One. 2017;12(8):e0181414. doi: 10.1371/journal.pone.0181414
- Bashford J, Flowers W, Haworth C, et al. Evaluation of a novel ELISA test using synthetic mycolic acid antigens for serodiagnosis of non-tuberculous mycobacterial (NTM) infections. Thorax. 2023;78(3):309–312. doi: 10.1136/thorax-2022-218800
- Esmail H, Cobelens F, Goletti D. Transcriptional biomarkers for predicting development of tuberculosis: progress and clinical considerations. Eur Respir J. 2020;55(3):1901957. doi: 10.1183/13993003.01957-2019
- Zak DE, Penn-Nicholson A, Scriba TJ, et al. A blood RNA signature for tuberculosis disease risk: a prospective cohort study. Lancet Lond Engl. 2016;387:2312–2322.
- Suliman S, Thompson EG, Sutherland J, et al. Four-gene pan-african blood signature predicts progression to tuberculosis. Am J Respir Crit Care Med. 2018;197(9):1198–1208. doi: 10.1164/rccm.201711-2340OC
- Darboe F, Mbandi SK, Thompson EG, et al. Diagnostic performance of an optimized transcriptomic signature of risk of tuberculosis in cryopreserved peripheral blood mononuclear cells. Tuberculosis. 2018;108:124–126. doi: 10.1016/j.tube.2017.11.001
- Darboe F, Mbandi SK, Naidoo K, et al. Detection of tuberculosis recurrence, diagnosis and treatment response by a blood transcriptomic risk signature in HIV-Infected persons on antiretroviral therapy. Front Microbiol. 2019;10:1441. doi: 10.3389/fmicb.2019.01441
- Scriba TJ, Fiore-Gartland A, Penn-Nicholson A, et al. Biomarker-guided tuberculosis preventive therapy (CORTIS): a randomised controlled trial. Lancet Infect Dis. 2021;21(3):354–365. doi: 10.1016/S1473-3099(20)30914-2
- Mendelsohn SC, Fiore-Gartland A, Penn-Nicholson A, et al. Validation of a host blood transcriptomic biomarker for pulmonary tuberculosis in people living with HIV: a prospective diagnostic and prognostic accuracy study. Lancet Glob Health. 2021;9(6):e841–e853. doi: 10.1016/S2214-109X(21)00045-0
- Penn-Nicholson A, Mbandi SK, Thompson E, et al. RISK6, a 6-gene transcriptomic signature of TB disease risk, diagnosis and treatment response. Sci Rep. 2020;10(1):8629. doi: 10.1038/s41598-020-65043-8
- Bayaa R, Ndiaye MDB, Chedid C, et al. Multi-country evaluation of RISK6, a 6-gene blood transcriptomic signature, for tuberculosis diagnosis and treatment monitoring. Sci Rep. 2021;11(1):13646. doi: 10.1038/s41598-021-93059-1
- WHO. High priority target product profiles for new tuberculosis diagnostics: report of a consensus meeting [Internet]. [cited 2023 Mar 23]. Available from: https://www.who.int/publications-detail-redirect/WHO-HTM-TB-2014.18.
- Kik SV, Schumacher S, Cirillo DM, et al. An evaluation framework for new tests that predict progression from tuberculosis infection to clinical disease. Eur Respir J. 2018;52(4):1800946. doi: 10.1183/13993003.00946-2018
- WHO. Consensus meeting report: development of a Target Product Profile (TPP) and a framework for evaluation for a test for predicting progression from tuberculosis infection to active disease [Internet]. [cited 2023 Mar 23]. Available from: https://www.who.int/publications-detail-redirect/WHO-HTM-TB-2017.18.
- Gupta RK, Turner CT, Venturini C, et al. Concise whole blood transcriptional signatures for incipient tuberculosis: a systematic review and patient-level pooled meta-analysis. Lancet Respir Med. 2020;8(4):395–406. doi: 10.1016/S2213-2600(19)30282-6
- Mendelsohn SC, Mbandi SK, Fiore-Gartland A, et al. Prospective multicentre head-to-head validation of host blood transcriptomic biomarkers for pulmonary tuberculosis by real-time PCR. Commun Med. 2022;2(1):1–13. doi: 10.1038/s43856-022-00086-8
- Grifoni A, Alonzi T, Alter G, et al. Impact of aging on immunity in the context of COVID-19, HIV, and tuberculosis. Front Immunol. 2023;14:1146704. doi: 10.3389/fimmu.2023.1146704.